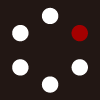
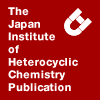
HETEROCYCLES
An International Journal for Reviews and Communications in Heterocyclic ChemistryWeb Edition ISSN: 1881-0942
Published online by The Japan Institute of Heterocyclic Chemistry
e-Journal
Full Text HTML
Received, 4th May, 2012, Accepted, 3rd August, 2012, Published online, 9th August, 2012.
DOI: 10.3987/REV-12-SR(N)2
■ Synthesis of Two Neurokinin NK1 Receptor Antagonists: (+)-L-733,060 and (-)-L-733,061
Anne Cochi, Domingo Gomez Pardo, and Janine Cossy*
Laboratoire de Chimie Organique, ESPCI, 10, rue Vauquelin
75231 PARIS Cedex 05, France
Abstract
This review describes synthetic approaches to (+)-L-733,060 and (–)-L-733,061 reported since 1994, focusing on methods allowing the construction and control of the two stereogenic centers. The methods are divided into three groups: (1) resolution of racemates (chemical or enzymatic resolution), (2) diastereoselective reactions using chiral pools or auxiliaries, and (3) enantioselective reactions (aminohydroxylation, epoxidation, deprotonation, allylic amination, aza-Henry reaction, vinylogous aldolization).CONTENTS
1. Introduction
2. Resolution of Racemates
2-1. Chemical Resolution
2-2. Enzymatic Resolution
3. Diastereoselective Reactions
3-1. From Amino Acids
3-1-1. L-Glutamic Acid
3-1-2. L-Phenylglycine
3-1-3. L-Pyroglutamic Acid and L-Proline
3-1-3-1. L-Pyroglutamic Acid
3-1-3-2. L-Proline
3-1-4. L-Serine
3-2. From Chiral Inductors: Optically Active Sufinyl Amides and Amines
3-2-1. Optically Active Sulfinyl Amides
3-2-2. Optically Active Phenylethylamine
3-3-3. Amino Chiral Auxiliairies
4. Enantioselective Reactions
4-1. Sharpless Asymmetric Aminohydroxylation
4-2. Sharpless Asymmetric Epoxidation
4.3. Shi Asymmetric Epoxidation
4-4. Enantioselective Deprotonation and Ring-Expansion
4-5. Enantioselective Allylic Amination
4-6. Enantioselective Aza-Henry Reaction
4-7. Enantioselective Organocatalytic Direct Vinylogous Aldolization
5. Conclusion
1. INTRODUCTION
The neurokinin Substance P (SP),1 an 11 amino-acid peptide, has been associated with a variety of biological effects such as smooth muscle contraction, neurological inflammation,2 gain transmission and regulation of the immune response.3 Thus, Substance P antagonists may be useful in a variety of disorders including migraine,4 rheumatoid arthritis,5 and pain.6 It has been reported that (2S,3S)-2-phenyl-3-[(3,5)-bis(trifluoromethyl)benzyloxy]piperidine, (+)-L-733,060, has a better affinity for the hNK1 receptor stably expressed in CHO cells than (2R,3R)-2-phenyl-3-[(3,5)-bis(trifluoromethyl)- benzyloxy]piperidine, (–)-L-733,061 (IC50= 1 nM versus IC50= 300 nM).7 These affinities were determined by competition with 125I-SP.8
In view of the potential pharmacological applications, several syntheses of enantiomers (+)-L-733,060 and (–)-L-733,061 have been reported, both in racemic and optically active forms. As (+)-L-733,060 is more biologically active than (–)-L-733,061, only a few syntheses of this latter compound have been realized. Different strategies have been utilized to access these two compounds; however, most of the reports deal with classical resolution of racemates (chemical and enzymatic resolution), use of chiral pool, chiral auxiliaries, enantioselective reagents or catalysts to produce one of the enantiomer. In most cases, the precursor of (+)-L-733,060 [or (–)-L-733,061] is N-Boc piperidin-3-ol (+)-4 [or (–)-4], which is transformed to the desired NK1 receptor antagonist in two steps. The first step is an etherification of the hydroxyl group in (+)-4 [or (–)-4] with 3,5-bistrifluoromethylbenzyl bromide under basic conditions, followed by the cleavage of the N-t-butyl carbamate using trifluoroacetic acid (or HCl) (Scheme 1).
2. RESOLUTION OF RACEMATES
In order to obtain the two enantiomers (+)-L-733,060 and (–)-L-733,061 and to compare their biological activities, the resolution of a racemate was performed.
2-1. Chemical Resolution
The first synthesis of L-733,060 and L-733,061 was performed at Merck in 1994.7 The synthesis of these two compounds started from keto lactam (±)-1 which was reduced (NaBH4, –20 °C) to provide hydroxyl lactam (±)-2. This lactam was then reduced with borane to produce cis-disubstituted piperidine (±)-3 which after resolution using (–)- and (+)-dibenzoyl tartaric acid led respectively to (+)-3 and (–)-3. The individual enantiomers were then converted to N-Boc protected piperidines (+)-4 and (–)-4, which were then converted in the previously described two-step sequence (etherification, deprotection, see Scheme 1) to L-733,060 and L-733,061 respectively (Scheme 2).
2-2. Enzymatic Resolution
As N-protected amino alcohols can be efficiently resolved by lipase catalyzed kinetic resolution, this process was successfully applied to amino alcohol (±)-69 to access L-733,060. Amino alcohol (±)-6 was prepared in five steps from 2-phenylethanoic acid 5 and then stirred with 2-propenyl acetate in toluene with particles of CAL-B at 70 °C. A clean acetylation of one enantiomer afforded acetylated (3R,4R)-7 with excellent enantiomeric purity (ee > 99%). Furthermore, unreacted amino alcohol (3S,4S)-6 also showed an excellent enantiomeric excess (ee > 99%). Due to the enzymatic resolution of amino alcohol (±)-syn-6, both L-733,060 and L-733,061 were obtained. The synthesis of L-733,060 was the only one reported. Thus, after benzoylation of (3S,4S)-6 (BzCl, pyridine, rt), the piperidine framework of L-733,060 was constructed through a Rh-catalyzed hydroformylation [Rh(acac)(CO)2 (3 mol %), BIPHEPHOS (6 mol %), 5 atm of CO–H2 (1:1)] providing enamide 8 (92%). Hydrogenation of 8 followed by alkaline hydrolysis [(1M) NaOH/MeOH/1,4-dioxane (2:3:6)] gave piperidine (+)-4, which was transformed in two steps to L-733,060 (etherification, deprotection, 85%) (Scheme 3).10
3. DIASTEREOSELECTIVE REACTIONS
The control of the C3 stereogenic center in L-733,060 and L-733,061 was realized by applying diastereoselective reactions to derivatives issued from natural amino acids and optically active amines in which the stereogenic center corresponds to the C2 stereogenic center present in L-733,060 and L-733,061.
3-1. From Amino Acids
L-Glutamic acid is a versatile starting material which allowed the synthesis of L-733,060 as well as L-733,061. Three other natural amino acids, L-phenylglycine, L-pyroglutamic acid, and L-proline were also used as precursors of L-733,060.
3-1-1. L-Glutamic Acid
A common precursor to L-733,060 and L-733,061 was the optically active glutarimide 11 which was synthesized in four steps from L-glutamic acid. At first, L-glutamic acid was transformed to γ-lactone carboxylic acid 9 by using a diazotation (H2SO4, NaNO2).11 Treatment of 9 with thionyl chloride and then p-methoxybenzylamine afforded lactone amide 10 (70% for the two steps). The ring expansion to convert 10 to glutarimide 11, precursor of L-733,060 and L-733,061, was achieved by treatment with t-BuOK at –78 °C (90%, ee = 98%).12 To realize the transformation of 11 to L-733,060, 11 was protected with t-butyldiphenylsilyl chloride (TBDPSCl, imidazole, CH2Cl2) and the resulting protected glutarimide was reduced with NaBH4 (–20 °C to –10 °C) to afford two separable diastereomers in a 82/18 ratio in favor of cis-isomer 12. The key step in the synthesis of L-733,060 is an intramolecular deoxygenative phenylation of 12 which was realized using BF3.OEt2 (CH2Cl2, rt) to produce the desired cis-disubstituted piperidine 13 in 80% yield via intermediate A. After reduction of 13 (LiAlH4, THF, rt) and a one-pot deprotection/protection sequence, (+)-4 was isolated in 71% yield and then transformed to L-733,060 in two steps (Scheme 4).12
In the synthesis of L-733,061, in order to control the (R)-configuration at C2, glutarimide 11 was protected as a benzyl ether. After reductive alkylation with phenylmagnesium bromide, and treatment with an excess of BF3.OEt2 (3 equiv) and triethylsilane, the predominantly trans-14 isomer (trans/cis = 94:6) was isolated with a global yield of 72%. After reduction and a one-pot hydrogenolysis/N-Boc protection sequence, the (2R,3S)-disubstituted piperidine 15 was isolated (75%). As the configuration of the hydroxyl group at C3 has to be inverted, a Swern oxidation followed by a selective reduction with L-selectride was conducted to lead to the desired L-733,061 precursor, compound (–)-4 (74% yield). Compound (–)-4 was transformed to L-733,061 by using the two usual final steps (Scheme 5).13
3-1-2. L-Phenylglycine
L-733,060 was synthesized from L-phenylglycine using a ring-closing metathesis (RCM)14 to construct the piperidine framework. The synthesis of L-733,060 started from commercially available L-phenylglycine, which was transformed to syn-1,2-amino alcohol 17 in four steps. L-Phenylglycine was converted to an N-Boc methyl ester (AcCl, MeOH, then Boc2O, Et3N, THF) which was reduced to alcohol 16 (NaBH4, LiCl, Et2O/THF, 84%). The obtained alcohol was then oxidized (Swern conditions) and the resulting aldehyde was treated in situ with vinylmagnesium bromide, yielding the corresponding allylic alcohol 17 with good diastereoselectivity (90/10) in favor of the syn-isomer according to a Cram-chelate model. After protection of the hydroxyl group (TBDMSCl, imidazole), an N-allylation was performed (NaH, allylBr, DMF) and, after desilylation (TBAF-AcOH), the obtained dienic compound 18 was involved in a RCM and then in a hydrogenation to produce piperidine (+)-4. This piperidine was then transformed to L-733,060 by the usual procedure (etherification, deprotection) (Scheme 6).15
A second synthesis of L-733,060 was realized from L-phenylglycine via a trans-vinyloxazoline intermediate. L-Phenylglycine was transformed to allylic benzamide 19, precursor of trans-vinyl- oxazoline 22, in eight steps.15 Acyclic allylic benzamide 19 was treated with Pd(0) [Pd(PPh3)4] under basic conditions (K2CO3, CH3CN, reflux) to obtain trans-vinyloxazoline 20 (78%).16 It is worth noting that under these conditions an elimination product, conjugated enamide 21, was formed in 13% yield. After ozonolysis of 20, the obtained aldehyde was reacted with methyl trimethylphosphonoacetate to yield α,β-unsaturated methyl ester 22 (87%). 1,4-Reduction of 22 with copper bromide, Red-Al, and 2-butanol gave saturated methyl ester 23 (83%). Hydrogenation of 23 with Pd(OH)2 in AcOH/MeOH was performed under 70 psi of H2 at room temperature followed by solvolysis of the resulting oxazolidine to directly afford hydroxypiperidinone 24. Reduction of 24 using borane-methyl sulfide complex and protection with Boc2O led to (+)-4, which was then transformed to L-733,060 using the two usual final steps (Scheme 7).17
3-1-3. L-Pyroglutamic Acid and L-Proline
L-733,060 and L-733,061 were obtained using a ring enlargement of pyrrolidine methanols I as the key tep to form 3-hydroxypiperidines II via aziridinium Z intermediates.18 Pyrrolidine methanols I were obtained either from L-pyroglutamic acid or L-proline (Scheme 8).
3-1-3-1. L-Pyroglutamic Acid
The synthesis of L-733,060 started with the transformation of methyl L-pyroglutamate, which was prepared from L-pyroglutamic acid, to the Weinreb amide which was treated with phenylmagnesium bromide to furnish phenyl ketone 25. To access pyrrolidine methanol syn-26, precursor of the 3-hydroxypiperidine core, phenyl ketone 25 was reduced with NaBH4 (THF, MeOH, 90%) to furnish a mixture of pyrrolidines syn-26 and anti-26 in a ratio 88/12.19 After treatment of the mixture with LiAlH4 and N-benzylation, prolinols syn-27 and anti-27 were formed and separated. The ring expansion of syn-27 was then performed with mesyl chloride (Et3N, THF) to afford 3-chloropiperidine 28 quantitatively via aziridinium B. N-Benzyl-3-chloropiperidine 28 was then heated with AcONa-Ac2O to produce the corresponding 3-acetoxypiperidine 29 as well as acetoxymethylpyrrolidine 30 in a ratio 80/20.20 In order to obtain (+)-4 with the (R)-configuration at C3, an inversion of the stereogenic center had to be realized. Thus, after hydrogenolysis in the presence of Boc2O (H2, Pd/C, Boc2O), acetolysis (NaOH 3N, 65 °C), Swern oxidation, and stereoselective reduction of the ketone with L-selectride, the precursor of L-733,060, piperidine (+)-47 was isolated with an overall yield of 70% (4 steps) (Scheme 9).21
3-1-3-2. L-Proline
The syntheses of L-733,060 and L-733,061 were realized from L-proline by utilizing a ring enlargement of prolinols with TFAA22 to access 3-hydroxypiperidine precursors (–)-4 and 36 (Scheme 11).
L-Proline was transformed to N-Boc proline methyl ester 31 which was then transformed to the corresponding amino alcohols anti-32 and syn-32 with excellent diastereoselectivity (de = 98%), in favor of the isomer anti-32, using Dibal-H followed by the addition of phenylmagnesium bromide (CH2Cl2, –78 °C to rt). This excellent diastereoselectivity is probably due to the complexation of aluminium with the N-Boc group (intermediate C). It is worth pointing out that when an N-benzyl group is present instead of a Boc group, no diastereoselectivity was observed. Indeed, treatment of N-benzylproline methyl ester 33 with Dibal-H followed by the addition of phenylmagnesium bromide (CH2Cl2, –78 °C to rt) afforded two separable diastereomers anti-27 and syn-27 in a 50/50 ratio with a yield of 72%. After a deprotection/protection sequence, amino alcohol anti-32 was transformed to anti-27 (64%) (Scheme 10).23
Amino alcohols anti-27 and syn-27 were respectively treated with TFAA/Et3N and then with NaOH to afford, via aziridiniums D and E respectively, piperidinols 34 and 35 with excellent diastereoselectivities (de > 99%) and enantioselectivities (ee > 99%). The transformation of 34 to (–)-47 and 35 to 36,21,33 precursors of L-733,061 and L-733,060 respectively, was realized in a two-step one-pot sequence hydrogenolysis/N-Boc protection (Scheme 11).23
3-1-4. L-Serine
Ethyl glycidate, which was prepared from L-serine,24 was transformed to L-733,060. This transformation began with the addition of phenyllithium to ester 37.25 Subsequent diastereoselective reductive amination of obtained ketone 38 led to anti-39/syn-39 in a ratio of 85/15. After separation of the two diastereomers, anti-39 was converted to oxazolidinone 40 by a regioselective intramolecular epoxide opening upon treatment with di-tert-butyldicarbonate.26 As oxidation difficulties were encountered due to the presence of the N-benzyl protecting group, the benzyl group was replaced by a N-Boc protecting group. Thus, 40 was treated with NaOH, the primary hydroxyl group was protected as a TBS-ether and after a hydrogenolysis/protection sequence (H2, Pd/C then Boc2O), carbamate alcohol 41 was isolated in 70% yield. In order to oxidize the primary alcohol, 41 was treated with dimethoxypropane (PTSA, CH2Cl2, rt), and the deprotection of the primary alcohol was realized affording oxazolidine 42. A Dess-Martin oxidation followed by a Horner-Wadsworth-Emmons reaction led to α,β-unsaturated ester 43 (67% overall yield). After cyclization of 43 by treatment under acidic conditions followed by hydrogenation (H2, Pd/C, THF), lactam 44 was isolated (68%) and transformed to (+)-4 after reduction (LiAlH4) and N-Boc protection (Boc2O, Et3N, DMAP, CH2Cl2) in 81% overall yield (Scheme 12).27
3-2. From Chiral Inductors: Optically Active Sulfinyl Amides and Amines
Optically active sulfinyl amides and amines were used to synthesize L-733,060 either to introduce the nitrogen of the piperidine ring or used to synthesize L-733,061 as a chiral auxiliary.
3-2-1. Optically Active Sulfinyl amides
Three syntheses of L-733,060 were easily realized diastereo- and enantioselectively from (R)-tert-butylsulfinyl amide. The addition of ketyl radicals or organometallics onto optically active sulfinyl imines allows the synthesis of protected 1,2-amino alcohols in good diastereoselectivies.
One route to access L-733,060 featured the SmI2-induced reductive coupling of N-tert-butanesulfinyl imine 45 with aldehyde 46 to lead to protected anti-1,2-amino alcohol 47 with excellent diastereoselectivity (de > 80%) and excellent enantiomeric excess (ee > 99%). As an inversion of the hydroxyl group is necessary to access L-733,060, hydrolysis of the sulfinyl amine (HCl, MeOH) followed by selective N-acylation with 4-methoxybenzoic anhydride were applied to 47, leading to amide 48 (88%). Upon treatment of 48 with MsCl/Et3N, oxazoline 49 was obtained with complete inversion of configuration at C2. Reductive ring-opening of oxazoline (NaBH3CN, AcOH) gave syn-1,2-amino alcohol 50 (90%). Due to deprotection problems of the para-methoxybenzyl (PMB) group at the ultimate stage of the synthesis of L-733,060, the PMB group was replaced by an N-Boc group and, after a selective O-benzylation [3,5-(CF3)2C6H3CH2Br, NaH, TBAI], 51 was formed (66% for the 3 steps). Routine removal of the pivaloyl group and ring-closure afforded N-Boc protected piperidine 52, which after removal of the Boc group led to L-733,060 (Scheme 13).28
(+)-(S)-(N-Benzylidene)-p-toluenesulfinamide 53 was transformed to protected syn-1,2-amino alcohol 57 in three steps. Addition of lithium-1,3-dithiane 54 to 53 gave 55 in 94% de and 81% yield for the major diastereomer. Hydrolysis and oxidation of the sulfonyl group in 55 by 1,3-dibromo-5,5-dimethylhydantoin in acetone/H2O afforded 56. A diastereoselective reduction of amino ketone 56 with NaBH4 gave the protected syn-1,2-amino alcohol 57 (dr = 91:9). After a protection/deprotection/tosylation sequence, piperidine 58 was formed (57% yield). Piperidine 58 was then transformed to L-733,060 after deprotection of the hydroxy group, O-benzylation, and N-tosyl deprotection with Na/NH3(liq.) at –78 °C (Scheme 14).29
A third synthesis of L-733,060 was realized from N-tert-butanesulfinyl imine 45, which was transformed to a protected anti-1,2-amino alcohol 60 by addition of the allenyl zinc 59. This protected amino alcohol was then transformed to piperidine 36. This represents a formal synthesis of L-733,060.
The synthesis of L-733,060 began with the addition of racemic allenyl zinc (±)-59 (4 equiv) to N-tert-butylsulfinyl imine 4530 to produce protected anti-1,2-amino alcohol 60 as a single isomer. This protected anti-1,2-amino alcohol was transformed to diene 61 after protodesilylation of the acetylenic group by TBAF, hydrogenation with Lindlar catalyst and N-allylation (NaH, AllylBr, THF/HMPA). To construct the piperidine core, 61 was subjected to a ring-closing metathesis (RCM) using Grubbs second generation catalyst (95% yield). After hydrogenation of the olefin using Raney nickel (MeOH, rt), acidic removal of the tert-butylsulfoxide group and concomitant MOM-ether cleavage, then N-Boc protection, 36 which represents a precursor of L-733,060, was isolated in 89% yield (Scheme 15).31
3-2-2. Optically Active Phenylethylamine
The synthesis of L-733,060 started from Baylis-Hillman adduct 63 formed from benzaldehyde and methyl acrylate. The obtained hydroxyl α,β-unsaturated ester 63 was transformed to acetyl derivative 64 by treatment with Ac2O and pyridine. When 64 was treated with chiral lithium amide (S)-65, a domino reaction featuring a stereoselective Ireland-Claisen rearrangement and an asymmetric Michael addition32 took place to produce 66 in 60% yield with good diastereoselectivity (dr > 97/3). Hydrogenolysis of δ-amino acid 66 (H2 50psi, Pd/C in AcOH) induced a subsequent lactamization and gave piperidinone 67 which was transformed to methyl ketone 68 in two steps, via a Weinreb amide using MeONHMe.HCl and n-BuLi as the base followed by the addition of MeMgBr in THF. A Baeyer-Villiger oxidation [urea hydrogen peroxide (UHP), TFAA], then reduction of the lactam (LiAlH4) and N-Boc protection provided 36. The synthesis of L-733,060 required the inversion of the hydroxy group at C3 in 36. This inversion was realized after TPAP-oxidation followed by stereoselective reduction of the formed ketone using CeCl3/NaBH4. Piperidine (+)-4 was obtained in 72% yield and then transformed to L-733,060 in two steps (etherification, deprotection) (Scheme 16).33
3-3-3. Using Amino Chiral Auxiliary
L-Valine can be transformed easily to amide 69, which was used as a chiral auxiliary in the preparation of a pyridinium salt that was transformed to an optically active 2,3-disubstituted 1,2-dihydropyridine.
The synthesis of L-733,061 started with the deprotonation of 3-hydroxypyridine 71 (NaH, DMF), followed by the addition of 3,5-bis(trifluoromethyl)benzyl bromide allowing the formation of pyridine 72 (70% yield). The addition of phenylmagnesium bromide to pyridinium salt F derived from amide 69 via 70 and pyridine 72 produced 1,2-dihydropyridine 73 (84%). The diastereoselective hydrogenation of 73 from the opposite face of the phenyl ring at C2 afforded piperidine 74 as a single diastereomer (dr > 95/5) in 52% yield. Finally, alane reduction of the amidine led to L-733,061 (75% yield) (Scheme 17).34
4. ENANTIOSELECTIVE REACTIONS
As enantioselective reactions can be versatile, both enantiomers L-733,060 and L-733,061 can be obtained easily.
4-1. Sharpless Asymmetric Aminohydroxylation
L-733,060 was synthesized by applying an aminohydroxylation of an unsaturated ester by utilizing (DHQ)2PHAL as the chiral inductor. The synthesis of L-733,060 started from isopropyl cinnamate 75 which was subjected to a Sharpless asymmetric aminohydroxylation35 using (DHQ)2PHAL, potassium osmate as the oxidant and N-bromoacetamide as the nitrogen source to produce desired protected amino alcohol 76 in 76% yield with excellent enantiomeric excess (superior to 99%). In order to access (+)-4, precursor of L-733,060, a deprotection/protection sequence was applied to 76 to produce 77. This compound was then subjected to a reduction/oxidation sequence to produce an aldehyde, which on a subsequent Wittig reaction gave α,β-unsaturated ester 78. Olefin reduction and concomitant deprotection of the hydroxyl group furnished hydroxyl ester 79. After reduction of the ester with Dibal-H, the resulting alcohol was transformed to the mesylate to furnish (+)-4, which was transformed to L-733,060 (Scheme 18).36
4-2. Sharpless Asymmetric Epoxidation
As the Sharpless epoxidation is versatile and the epoxide ring-opening can be regioselective, these reactions can be used to synthesize L-733,060 and L-733,061. The starting material to access L-733,060 was (E)-cinnamyl alcohol 80, which was epoxidized according to Sharpless conditions using (S,S)-(–)-DET as the chiral inductor. The regioselective epoxide opening of 81 with NaN3 gave a single regioisomer in excellent yield (98%). To obtained the desired syn configuration of the azido alcohol to access L-733,060, epoxy alcohol 81 was transformed to azido epoxide 83 in a three-step sequence involving a chemoselective pivaloylation of diol 82, mesylation of the secondary alcohol (MsCl, Et3N, DMAP) and treatment of the mesylate with K2CO3 in MeOH. In order to construct the carbon skeleton of L-733,060, epoxide 83 was then treated with allylsilane (3 equiv) and TiCl4 in CH2Cl2 at –78 °C. After elimination of byproduct 84, azido alcohol 85 was protected (TBSOTf, 2,6-lutidine). After oxidative cleavage of the double bond (OsO4, NaIO4), 86 was isolated without further purification. When a one-pot Staudinger/aza-Wittig reaction was applied to 86, an intramolecular condensation took place to produce a six-membered imine that was reduced (NaBH4) in situ to produce 87. The resulting piperidine 87 was then protected as an N-Boc derivative and the TBS protecting group was cleaved to furnish (+)-4, a precursor of L-733,060. The usual two final reactions (etherification/deprotection) applied to (+)-4 afforded L-733,060 (Scheme 19).37
4-3. Shi Asymmetric Epoxidation
The Shi epoxidation was used as an alternative to the Sharpless epoxidation to access L-733,060, using a D-fructose dioxirane derivative. The synthesis of L-733,060 started with a Johnson-Claisen [3,3]-sigmatropic rearrangement applied to allylic alcohol 88 [MeC(OMe)3, EtCO2H, 135 °C] to construct its carbon backbone, and led exclusively to (E)-homoallylic ester 89 (82% yield).38 After alkaline hydrolysis of the ester, the potassium carboxylate was subjected to Shi epoxidation39 using D-fructose-derivative ketone 90 as the chiral catalyst (30 mol %), and oxone (2 KHSO5-KHSO4-K2SO4) as the stoichiometric oxidant to afford hydroxylactone 91 in 62% yield and 92% ee. Mesylation of the alcohol, followed by treatment of the obtained mesylate with NaN3, afforded azidolactone 92 with inversion of configuration. Reduction of the azide under Staudinger conditions (PPh3, THF, H2O) produced lactam 93 (91% yield) via presumably intramolecular O-to-N-ring expansion by the amine generated in situ. Reduction of lactam 93 with BH3.SMe2 in THF followed by protection of the secondary amine with Boc2O gave the cis-amino alcohol (+)-4 (73% yield over the two steps), which was transformed to L-733,060 (Scheme 20).40
Four other enantioselective reactions were utilized to access L-733,060 and L-733,061: an enantioselective deprotonation/ring-expansion, an enantioselective allylic amination, an enantioselective aza-Henry reaction, and an enantioselective organo-catalyzed direct vinylogous aldolization.
4-4. Enantioselective Deprotonation and Ring-Expansion
A catalytic asymmetric deprotonation step of N-Boc pyrrolidine 94 using s-BuLi, (–)-sparteine in the presence of lithiated 2-dimethylaminoethanol (LiDMAE) led after addition of benzaldehyde to prolinols syn-95 and anti-95 in a ratio of 75/25. Prolinol syn-95 was isolated in 64% yield with an ee of 80% and the isomer anti-95 was isolated in 25% yield with an ee of 78%. After separation of syn-95 and anti-95, syn-95 was N-deprotected and converted to N-allyl-2-hydroxymethylpyrroline 96 that was subjected to a ring expansion leading to piperidine 97 using TFAA/ET3N, then NaOH.22 The remaining two steps proceeded in high yield. O-Benzylation of 97 was achieved by using a NaH deprotonation followed by alkylation of the alcoholate with functionalized benzyl bromide. Finally, treatment of obtained 98 with Pd(0) and N,N’-dimethylbarbituric acid produced a deallylation to give L-733,060 with an enantiomeric ratio of 90:10 (Scheme 21).41
4-5. Enantioselective Allylic Amination
A palladium-catalyzed enantioselective allylic amination has been used as the key step in the synthesis of L-733,061. Pd-catalyzed asymmetric allylic amination of allyl acetate 99 using chiral ligand 100 afforded allyl amine 101 in 90% yield and 99% ee.42 Compound 101 was then converted to diene 102 by treatment with 3-butenoic acid (DCC, DMAP, CH2Cl2). A RCM applied to 102, using the second-generation Grubbs catalyst, afforded the desired unsaturated 2-phenylpiperidinone 103 (94% yield). The 3-hydroxy group of L-733,061 was introduced by epoxidation of 103 (m-CPBA). Reduction with LiAlH4 allowed the reduction of the amide and the regioselective ring-opening of the epoxide. This regioselective ring-opening occurred probably via conformer G, in which the steric interaction between the benzyl group on the nitrogen and the phenyl group at C2 is minimal. Then, the hydride anion can attack from the β-axial site at C4, affording desired trans-product 104, which was then converted to N-Boc product 15 [Pd(OH)2, H2, Boc2O, EtOAc, 45 °C]. After four steps, 15 was transformed to L-733,061 by inversion of the configuration of the stereogenic center at C3. After Swern oxidation, stereoselective reduction of the formed ketone by L-Selectride (THF, –20 °C), O-benzylation of the hydroxyl group in (–)-4 (NaH, 3,5-bistrifluoromethylbenzyl bromide), and N-Boc cleavage, L-733,061 was isolated with an overall yield of 63% (4 steps) (Scheme 22).43
4-6. Enantioselective Aza-Henry Reaction
Recently, an efficient enantioselective synthesis of L-733,061 was accomplished using an organo-catalyzed enantioselective aza-Henry reaction.
The reaction of N-Boc sulfone 105 with nitro compound 106 employing CsOH.H2O and phase transfer catalyst quinidine salt 107 (15 mol %) in toluene at –55 °C for 44 h resulted in product 108 in 85% yield with an anti/syn ratio of 85:15 in favor of the anti-isomer with an ee of 94%. After oxidation of the nitro functionality of 108 to a ketone (NaNO2, DMF/H2O) and reduction with NaBH4/CeCl3, the syn-selective secondary alcohol 109 was obtained with a dr of 90/10. The secondary alcohol was then O-benzylated with 3,5-bis-trifluoromethylbenzyl bromide under basic conditions, and after cleavage of the TBS group, the resulting primary alcohol was mesylated and the amine deprotected (TFA). When the resulting TFA ammonium salt was treated under basic conditions (Et3N, MeOH, 2h, 60 °C), the desired L-733,061 was isolated in 38% overall yield (5 steps) (Scheme 23).44
4-7. Enantioselective Organo-catalytic Direct Vinylogous Aldolization
L-733,060 was synthesized by applying an enantioselective organo-catalyzed direct vinylogous aldolization to γ-crotonolactone45 by utilizing a chiral aminosquaramide as the inductor.
The synthesis of L-733,060 started from γ-crotonolactone 110. The direct vinylogous aldolization of this γ-crotonolactone with benzaldehyde in the presence of aminosquaramide catalyst 111 provided butenolide 112 in 74% yield, with good diastereoselectivity (anti/syn: 89/11) and excellent enantiomeric excess (ee >99% for the anti-diastereomer). The diastereomeric mixture 112 was converted to lactam 114 via a series of simple transformations. After hydrogenation of the double bond in 112, subsequent mesylation of the secondary alcohol, and displacement of the mesylate by an azide anion with inversion of configuration, azido butyrolactone 113 was isolated. Reduction of the azide in 113 (H2, Pd/C) in the presence of a base (K2CO3, MeOH), via an intramolecular N-acylation of the in situ generated amino butyrolactone, led to required piperidones 114. At this stage, cis-114 was separated from minor diastereomer trans-114. After reduction of piperidone cis-114 with borane, the corresponding piperidine was formed and converted to N-Boc derivative (+)-4 in 82% yield. Compound (+)-4 was then transformed (etherification, deprotection) to L-733,060 by the two usual final reactions (Scheme 24).46
5. CONCLUSION
This review described the syntheses of two enantiomeric neurokinin NK1 receptor antagonists (+)-L-733,060 and (–)-733,061 reported up to date. As most of the syntheses are versatile, library of (+)-L-733,060 and (–)-733,061 should be obtained for biological tests.
References
1. U. S. von Euler and J. H. Gaddum, J. Physiol., 1931, 72, 74; M. M. Chang, S. E. Leeman, and H. D. Niall, Nat. New Biol., 1971, 232, 86. CrossRef
2. M. Lotz, J. H. Vaughan, and D. A. Carson, Science, 1988, 241, 1218. CrossRef
3. A. Perianan, R. Synderman, and B. Malfroy, Biochem. Biophys. Res. Commun., 1989, 161, 520. CrossRef
4. M. A. Moskowitz, Trends Pharmacol. Sci., 1992, 13, 307. CrossRef
5. M. Lotz, D. A. Carson, and J. H. Vaughan, Science, 1987, 235, 893. CrossRef
6. M. Otsuka and M. Yanagisawa, J. Physiol., 1988, 395, 255.
7. T. Harrison, B. J. Williams, C. J. Swain, and R. G. Ball, Bioorg. Med. Chem. Lett., 1994, 4, 2545. CrossRef
8. T. M. Fong, S. A. Anderson, H. Yuh, R. R. C. Huang, and C. D. Strader, Mol. Pharm., 1992, 41, 24; A. M. Cascieri, E. Ber, T. M. Fong, S. Sadowski, A. Bansal, C. Swain, E. Seward, B. Frances, D. Burns, and C. D. Strader, Mol. Pharm., 1992, 42, 458.
9. T. Oshitari and T. Mandai, Synlett, 2003, 2374. CrossRef
10. T. Oshitari and T. Mandai, Synlett, 2006, 3395. CrossRef
11. O. H. Gringore and F. P. Rouessac, 'Organic Syntheses,' Coll. Vol. VII, ed. by J. P. Freeman, John Wiley & Sons, New York, 1990, pp. 99.
12. P.-Q. Huang, L.-X. Liu, B.-G. Wei, and Y.-P. Ruan, Org. Lett., 2003, 5, 1927. CrossRef
13. L.-X. Liu, Y.-P. Ruan, Z.-Q. Guo, and P.-Q. Huang, J. Org. Chem., 2004, 69, 6001. CrossRef
14. For recent review see: P. Merino, T. Tejero, G. Greco, E. Marca, I. Delso, A. Gomez-SanJuan, and R. Matute, Heterocycles, 2012, 84, 75 and references therein. CrossRef
15. G. Bhaskar and B. V. Rao, Tetrahedron Lett., 2003, 44, 915. CrossRef
16. K. Y. Lee, Y. H. Kim, M. S. Park, and W. H. Ham, Tetrahedron Lett., 1998, 39, 8129; CrossRef K. Y. Lee, Y. H. Kim, M. S. Park, C. Y. Oh, and W. H. Ham, J. Org. Chem., 1999, 64, 9450. CrossRef
17. Y.-J. Yoon, J.-E. Joo, K.-Y. Lee, Y.-H. Kim, C.-Y. Oh, and W.-H. Ham, Tetrahedron Lett., 2005, 46, 739. CrossRef
18. For reviews; J. Cossy and D. Gomez Pardo, Chemtracts, 2002, 15, 579; J. Cossy and D. Gomez Pardo, 'Targets in Heterocyclic Systems,' Vol. 6, ed. by O. A. Attanasi and D. Spinelli, Italian Society of Chemistry, Rome, 2002, pp. 1; J. Cossy, D. Gomez Pardo, C. Dumas, O. Mirguet, I. Déchamps, T.-X. Métro, B. Burger, R. Roudeau, J. Appenzeller, and A. Cochi, Chirality, 2009, 21, 850. CrossRef
19. A. Ookawa and K. Soai, J. Chem. Soc., Perkin Trans. 1, 1987, 1465; CrossRef K. Soai and K. Ohi, Bull. Chem. Soc. Jpn., 1985, 58, 1601; CrossRef K. Soai, A. Ookawa, T. Kaba, and K. Ogawa, J. Am. Chem. Soc., 1987, 109, 711. CrossRef
20. O. Calvez, A. Chiaroni, and N. Langlois, Tetrahedron Lett., 1998, 39, 9447. CrossRef
21. O. Calvez and N. Langlois, Tetrahedron Lett., 1999, 40, 7099. CrossRef
22. J. Cossy, C. Dumas, and D. Gomez Pardo, Tetrahedron Lett., 1995, 36, 549; CrossRef J. Cossy, C. Dumas, and D. Gomez Pardo, Synlett, 1997, 905; CrossRef J. Cossy, C. Dumas, and D. Gomez Pardo, Bioorg. Med. Chem. Lett., 1997, 7, 1343; CrossRef J. Wilken, M. Kossenjans, W. Saak, D. Haase, S. Pohl, and J. Martens, Liebigs Ann./Recl., 1997, 573; CrossRef N. Langlois and O. Calvez, Synth. Commun., 1998, 28, 4471; CrossRef P. W. Davis, S. A. Osgood, N. Hébert, K. G. Sprankle, and E. E. Swayze, Biotechnol. Bioeng., 1999, 28, 4471; J. Cossy, C. Dumas, and D. Gomez Pardo, Eur. J. Org. Chem., 1999, 1693; CrossRef P. Michel and A. Rassat, J. Org. Chem., 2000, 65, 2572; CrossRef J. Cossy, O. Mirguet, and D. Gomez Pardo, Synlett, 2001, 1575; CrossRef A. Brandi, S. Cicchi, V. Paschetta, D. Gomez Pardo, and J. Cossy, Tetrahedron Lett., 2002, 43, 9357; CrossRef A. Deyine, J.-M. Delcroix, and N. Langlois, Heterocycles, 2004, 64, 207; CrossRef I. Déchamps, D. Gomez Pardo, P. Karoyan, and J. Cossy, Synlett, 2005, 1170; CrossRef R. Roudeau, D. Gomez Pardo, and J. Cossy, Tetrahedron, 2006, 62, 2388; CrossRef M. Mena, J. Bonjoch, D. Gomez Pardo, and J. Cossy, J. Org. Chem., 2006, 71, 5930; CrossRef I. Déchamps, D. Gomez Pardo, and J. Cossy, ARKIVOC, 2007, v, 38; I. Déchamps, D. Gomez Pardo, and J. Cossy, Tetrahedron, 2007, 63, 9082; CrossRef T.-X. Métro, D. Gomez Pardo, and J. Cossy, J. Org. Chem., 2007, 72, 6556; CrossRef T.-X. Métro, D. Gomez Pardo, and J. Cossy, Synlett, 2007, 2888; CrossRef A. Rives, Y. Génisson, V. Faugeroux, N. Saffon, and M. Baltas, Synthesis, 2009, 3251. CrossRef
23. A. Cochi, B. Burger, C. Navarro, D. Gomez Pardo, J. Cossy, Y. Zhao, and T. Cohen, Synlett, 2009, 2157. CrossRef
24. Y. Petit and M. Larchevêque, 'Organic Syntheses,' Vol. 75, ed. by A. B. Smith, III, John Wiley & Sons, New York, 1998, pp. 37.
25. L. Pégorier, Y. Petit, A. Mambu, and M. Larchevêque, Synthesis, 1994, 1403. CrossRef
26. M. Haddad and M. Larchevêque, Tetrahedron Lett., 1996, 37, 4525. CrossRef
27. S. Prévot, P. Phansavath, and M. Haddad, Tetrahedron: Asymmetry, 2010, 21, 16. CrossRef
28. R.-H. Liu, K. Fang, B. Wang, M.-H. Xu, and G.-Q. Lin, J. Org. Chem., 2008, 73, 3307. CrossRef
29. F. A. Davis and T. Ramachandar, Tetrahedron Lett., 2008, 49, 870. CrossRef
30. F. Ferreira, C. Botuha, F. Chemla, and A. Pérez-Luna, Chem. Soc. Rev., 2009, 38, 1162. CrossRef
31. B. Hélal, F. Ferreira, C. Botuha, F. Chemla, and A. Pérez-Luna, Synlett, 2009, 3115. CrossRef
32. N. M. Garrido, M. Garcia, D. Diez, M. R. Sanchez, F. Sanz, and J. G. Urones, Org. Lett., 2008, 10, 1687. CrossRef
33. N. M. Garrido, M. Garcia, M. R. Sanchez, D. Diez, and J. G. Urones, Synlett, 2010, 387. CrossRef
34. A. Lemire, M. Grenon, M. Pourasharf, and A. B. Charette, Org. Lett., 2004, 6, 3517. CrossRef
35. G. Li, H. H. Angert, and K. B. Sharpless, Angew. Chem., Int. Ed. Engl., 1996, 35, 2813; CrossRef M. Bruncko, G. Schlingloff, and K. B. Sharpless, Angew. Chem., Int. Ed. Engl., 1997, 36, 1483; CrossRef S. H. Lee, J. Yoon, S. H. Chung, and Y. S. Lee, Tetrahedron, 2001, 57, 2139. CrossRef
36. S. R. V. Kandula and P. Kumar, Tetrahedron: Asymmetry, 2005, 16, 3579. CrossRef
37. S. K. Cherian and P. Kumar, Tetrahedron: Asymmetry, 2007, 18, 982. CrossRef
38. W. S. Johnson, L. Werthemann, W. R. Bartlett, T. J. Brocksom, T.-T. Li, D. J. Faulkner, and M. R. Petersen, J. Am. Chem. Soc., 1970, 92, 741; CrossRef K. Mori, T. Nukada, and T. Ebata, Tetrahedron, 1981, 37, 1343. CrossRef
39. D. J. Ager, K. Anderson, E. Oblinger, Y. Shi, and J. VanderRoest, Org. Process Res. Dev., 2007, 11, 44. CrossRef
40. L. Emmanuvel and A. Sudalai, Tetrahedron Lett., 2008, 49, 5736. CrossRef
41. J. L. Bilke, S. P. Moore, P. O’Brien, and J. Gilday, Org. Lett., 2009, 11, 1935. CrossRef
42. H. Nakano, K. Takahashi, Y. Suzuki, and R. Fujita, Tetrahedron: Asymmetry, 2005, 16, 609. CrossRef
43. K. Takahashi, H. Nakano, and R. Fujita, Tetrahedron Lett., 2005, 46, 8927. CrossRef
44. G. Kumaraswamy and A. Pitchaiah, Tetrahedron, 2011, 67, 2536. CrossRef
45. H. Ube, N. Shimada, and M. Terada, Angew. Chem. Int. Ed., 2010, 49, 1858; CrossRef Y. Yang, K. Zheng, J. Zhao, J. Shi, L. Lin, X. Liu, and X. Feng, J. Org. Chem., 2010, 75, 5382; CrossRef S. V. Pansare and E. K. Paul, Chem. Commun., 2011, 47, 1027. CrossRef
46. S. V. Pansare and E. K. Paul, Org. Biomol. Chem., 2012, 10, 2119. CrossRef