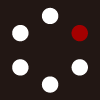
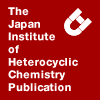
HETEROCYCLES
An International Journal for Reviews and Communications in Heterocyclic ChemistryWeb Edition ISSN: 1881-0942
Published online by The Japan Institute of Heterocyclic Chemistry
e-Journal
Full Text HTML
Received, 29th June, 2012, Accepted, 7th August, 2012, Published online, 15th August, 2012.
DOI: 10.3987/COM-12-S(N)72
■ SYNTHESIS AND REACTIONS OF SILICON-BRIDGED DITHIENYLBIPHENYLS. FINE TUNING OF ELECTRONIC STATES BY BRIDGING SILICON CHAIN LENGTHS
Joji Ohshita,* Kazuya Murakami, Daiki Tanaka, and Hiroto Yoshida
Chemistry and Chemical Engineering, Graduate School of Engineering, Hiroshima University, 1-4-1 Kagamiyama, Higashi-hiroshima, Hiroshima 739-8527, Japan
Abstract
4,4’-Bis(ethylthienyl)-2,2’-(tetramethyl- and tetrabutyldisilano)biphenyls were prepared by the Migita-Kosugi-Stille coupling reactions of ethyl(trimethylstannyl)thiophene with the corresponding dibromo(disilano)biphenyls. The disilane-bridge of the former underwent oxidation with trimethylamine oxide and Pd-catalyzed benzyne-insertion to form siloxane- and disilylbenzene-bridged biphenyls, respectively. The UV spectra of these compounds showed blue-shifted absorption as the silicon-bridges were elongated. A conjugated polymer with an alternating arrangement of disilanobiphenyl and bithiophene units was also prepared and its oxidation was examined.INTRODUCTION
π-Conjugated compounds including oligomers and polymers are of importance as materials for organic electronic devices, such as organic light emitting diodes (OLEDs) and photovoltaic cells.1 To develop high-performance organic devices, the organic materials used in the devices should have appropriate HOMO and LUMO energy levels to exhibit desired electrical and optical properties as well as to promote smooth electron transport between the organic materials and also from/to the electrodes in the devices. Fine tuning of the electronic states of the π-conjugated materials is, therefore, an important subject in areas of organic device chemistry.
Silicon-bridged π-conjugated compounds, such as dibenzo- (1)2 and dithienosiloles (1’)3 are widely studied as the building blocks of π-conjugated functional oligomers and polymers (Chart 1). It is well established that they have extended conjugation due to the high planarity of the bridged π-systems and also to their low-lying LUMO, arising from the bonding interaction between the carbon π*- and the silicon σ* orbital. Recently, we demonstrated that disilane-bridged biphenyl (2 in Chart 1) also possessed low-lying LUMO.4 This gave rise to sufficiently high electron-affinity, which permit the use of its vapor-deposited film as the electron-transporting layer in multi-layered OLEDs, although the reduced planarity of the biphenyl unit by elongating the silicon bridge led to the suppressed π-conjugation slightly. In this paper, we report the synthesis of disilane-bridged biphenyls bearing conjugated thiophene substituents. We examined oxygen- and benzyne-insertion to the disilane unit and found that elongation of the bridging silicon chain by these reactions led to step-by-step blue-shifts of the absorption bands. In addition, a conjugated polymer with alternating disilanobiphenyl and bithiophene units was prepared and its oxidation was examined. The optical properties of the polymers are also described.
RESULTS AND DISCUSSION
Synthesis and Reactions of Dithienyl(disilanobiphenyl)s. The palladium-catalyzed Stille coupling of dibromo(tetramethyl- and tetrabutyldisilanobiphenyl)s and ethyl(trimethylstannyl)thiophene gave dithienyl(disilanobiphenyl)s 2a and 2b as shown in Scheme 1. For the preparation of compound 2a with Pd(PPh3)4/CuI as the catalyst, formal extrusion of dimethylsilylene competed the coupling leading to the formation of a mixture of 2a and 1a (Scheme 1) in an approximate ratio of 6:4 (42% yield in total, 25% for 2a, after silica gel column chromatography). Further purification by recrystallization improved the ratio to ca. 2a:1a = 9:1, which was used for the optical studies (vide infra). Employing CuO as the cocatalyst instead of CuI (Pd2(dba)3/(o-Tol)3P/CuO) lowered the yield of 2a with the formation of siloxane byproduct 3a giving a mixture of 2a:1a:3a = 4:2:4 (55% yield in total, 22% for 2a). Oxidation of a mixture of 2a and 3a obtained from the reaction above, with trimethylamine oxide gave pure 3a. On contrary, sterically more hindered dibromo(tetrabutyldisilano)biphenyl underwent smooth coupling with ethyl(trimethylstannyl)thiophene in the presence of Pd2(dba)3/(o-Tol)3P/CuO as the catalyst to give 2b in 55% isolated yield with the formation of a trace of the dibutyl analogue of 1a. No siloxane derivative analogous to 3a was detected in the reaction mixture.
Optical data of compounds 1a, 2a,b, and 3a were collected as shown in Table 1. The UV absorption and photoluminescence (PL) spectra are depicted in Figure 1. Interestingly, the UV absorption maxima of these compounds shifted to shorter wavelengths as elongating the bridging silicon chain. Silole 1a showed a smaller Stokes shift than the others, probably reflecting its rigid structure. Generally, dibenzosiloles show highly PL properties.2a-c Among the present compounds, however, siloxane 3a exhibited the highest PL quantum efficiency of Φ = 0.73 in chloroform. Table 1 summarizes also the results of DFT calculations of these silicon-bridged biphenyls at the level of B3LYP/6-31G(d). The enlargement of the angles between the benzene planes seems to be primarily responsible for the increased HOMO-LUMO energy gaps in the order of 1a < 2a,b < 3a, although the degree of σ-π and σ*-π* interactions would be also affected by the nature of the silicon-bridges to an extent. To prepare compounds with a further enlarged interplane angle, we examined palladium-catalyzed benzyne insertion to the Si-Si bond of 2a.5 The reaction of a mixture of 2a gave a disilylbenzene-bridged compound (4a) as a 10:1 mixture with 3a as shown in Scheme 2. As expected, compound 4a exhibited the absorption and PL bands at even shorter wavelengths than those of 3a. No benzyne-insertion occurred with 2b under the same reaction conditions. One might consider the possibility of that the optical properties of 2a and 4a were affected by traces of 1a and 3a, respectively. However, as shown in Figure 1, no peaks of these contaminations were seen in the UV spectra. Moreover, compounds 2a showed closely similar UV absorption and PL spectra to those of 2b that was obtained in a pure form, again indicating no significant influence of the contamination of 1a on the optical properties of 2a.
Polymer Synthesis. We next studied the synthesis of disilanobiphenyl-containing polymers. However, an attempt to prepare a tetrabutyldisilanobiphenyl-bithiophene alternating polymer by the Stille coupling under the same conditions as those for the preparation of 2b was unsuccessful (Scheme 3). In this reaction, we obtained a polymeric substance in only 22% yield, which was almost insoluble in any organic solvents. On the other hand, a similar reaction without using CuO proceeded smoothly to give polymer P2b as red-brown solids in 85% yield (Scheme 3 and Table 2). Polymer P2b thus obtained was soluble in chloroform and THF, and slightly soluble in hexane, but insoluble in 2-propanol and acetone. Treatment of P2b with trimethylamine oxide gave a siloxane polymer P3b in 54% yield. The formation of siloxane bonds was confirmed by the generation of the stretching band at 974 cm-1 in the IR spectrum (Figure 2). Polymer P3b exhibited lower molecular weight than that of P2b. This was presumably due to the less soluble properties of P3b. The higher molecular weight fraction of P3b would be separated by filtration as insoluble substances. As expected, polymer P3b exhibited blue-shifted absorption and emission bands from those of P2b as shown in Figure 3.
In conclusion, we prepared a series of silicon-bridged biphenyls and found that their electronic states could be finely tuned by changing the bridging silicon chain length, being potentially applicable for the development of the organic electronic device materials. It is also noteworthy that such tuning could be operative even in the polymeric system.
EXPERIMENTAL
General. All reactions were carried out in dry argon. THF was dried over sodium/benzophenone ketyl and distilled immediately before use. Toluene and DMF were distilled from P2O5 and CaH2, respectively, and stored over activated molecular sieves until use. NMR spectra were recorded on Varian 400-MR and System-500 spectrometers. APCI mass spectra were measured on a Thermo Fisher Scientific LTQ Orbitrap XL spectrometer at N-BARD Hiroshima University, while El-mass spectra were recorded on a Shimadzu QP-2020A spectrometer. UV absorption and PL spectra were measured on HITACHI U2910 and F4500 spectrophotometers, respectively. PL quantum yields were determined in an integrating sphere fitted with a Hamamatsu Photonic C7473 multi-channel analyzer. DSC was performed on an SII EXTAR 6000-DSC6200 thermal analyzer. GPC was carried out using subsequently connected Shodex KF2001 and KF2002 columns and THF as the eluent. Hydrolytic workup mentioned below involves hydrolysis of the reaction mixture with water, separation of the organic layer, and extraction of the aqueous layer with chloroform or toluene, drying the combined organic layer and extracts over anhydrous magnesium sulfate, and evaporation of the solvent, in that order.
Preparation of Dibromo(disilano)biphenyls. To a solution of 2.81 g (5.00 mmol) of 4,4’-dibromo-2,2’-diiodobiphenyl2a in 60 mL of THF was added 11.8 mL (4.00 eq) of a 1.70 M tert-butyllithium in pentane at -90 °C over a period of 2 h and the resulting mixture was stirred at this temperature for 1 h. To this was added 1.9 mL (2.0 eq) of 1,2-dichlorotetramethyldisilane and the resulting mixture was allowed to warm to room temperature. After hydrolytic workup as above, silica gel column chromatography of the residue eluting with hexane, followed by recrystallization of the resulting crude solids from ethanol afforded 1.51 g of dibromo(tetramethyldisilano)biphenyl (71% yield) as colorless solids: mp 159.0-160.8 ºC; EI-MS m/z 424 [M+]; 1H NMR (CDCl3) δ 0.23 (s, 12H, SiMe), 7.25 (d, 2H, J = 8.0 Hz, ring H), 7.52 (d, 2H, J = 2.4 Hz, ring H), 7.55 (dd, 2H, J = 8.0, 2.4 Hz, ring H); 13C NMR (CDCl3) δ -5.57, 121.95, 131.33, 132.85, 135.89, 138.71, 144.88; 29Si NMR (CDCl3) δ -27.11. Anal. Calcd for C16H18Br2Si2: C, 45.08; H, 4.26. Found: C, 45.12; H, 4.14.
Dibromo(tetrabuthyldisilano)biphenyl was obtained in a similar fashion to that above, using 1,1,2,2-tetrabutyldichlorodisilane instead of 1,2-dichlorotetramethyldisilane, as colorless solids in 52% yield after silica gel column chromatography followed by recrystallization from ethanol: mp 65.1-65.7 ºC; EI-MS m/z 594 [M+]; 1H NMR (CDCl3) δ 0.75-0.84 (m, 20H, Bu), 1.16-1.58 (m, 16H, Bu), 7.21 (br d, 2H, J = 6.8 Hz, benzene ring H), 7.51 (br s, 2H, benzene ring H), 7.52 (dd, 2H, J = 6.8, 2.4 Hz, benzene ring H); 13C NMR (CDCl3) δ 10.81, 13.62, 26.45, 27.16, 121.75, 131.42, 132.66, 136.19, 137.85, 145.50; Anal. Calcd for C28H42Br2Si2: C, 56.56; H, 7.12. Found: C, 56.63; H, 7.12.
Preparation of Compounds 1a and 2a,b. A mixture of 0.213 g (0.500 mmol) of dibromo(tetramethyldisilano)biphenyl, 0.275 g (2.00 eq) of ethyl(trimethylstannyl)thiophene, 5 mol% of tetrakis(triphenylphosphine)palladium, 5 mol% of CuI, and 10 mL of toluene was stirred at 110 °C for 24 h. After hydrolytic workup as above, the residue was subjected to silica gel column chromatography eluting with hexane to give 0.103 g of a 6:4 mixture of 2a and 1a as yellow solids (42% yield in total, 25% for 2a), which was recrystallized from hexane to improve the purity of 2a to 9:1. Analytically pure 1a was obtained by recycling preparative GPC with toluene as eluent. Data for 1a: mp 155.6-157.4 ºC; EI-MS m/z 430 [M+];1H NMR (CDCl3) δ 0.47 (s, 6H, SiMe), 1.35 (t, 6H, J = 7.6 Hz, Et), 2.88 (qd, 4H, J = 7.6, 1.2 Hz, Et), 6.78 (dt, 2H, J = 3.6, 1.2 Hz, thiophene ring H), 7.17 (d, 2H, J = 3.6 Hz, thiophene ring H), 7.62 (dd, 2H, J = 8.4, 2.0 Hz, benzene ring H), 7.77 (d, 2H, J = 8.4 Hz, benzene ring H), 7.80 (d, 2H, J = 2.0 Hz, benzene ring H); 13C NMR (CDCl3) δ -3.18, 15.92, 23.63, 121.09, 122.55, 124.37, 127.45, 129.72, 133.58, 139.66, 141.71, 146.29, 147.12; APCI Exact MS Calcd for C26H27S2Si [M+H+]: 431.13180, Found: 431.13132. Data for 2a: EI-MS m/z 488 [M+]; 1H NMR (CDCl3) δ 0.27 (s, 12H, SiMe), 1.35 (t, 6H, J = 7.6 Hz, Et), 2.88 (qd, 4H, J = 7.6, 1.2 Hz, Et), 6.78 (dt, 2H, J = 3.6, 1.2 Hz, thiophene ring H), 7.17 (d, 2H, J = 3.6 Hz, thiophene ring H), 7.44 (d, 2H, J = 8.4 Hz, benzene ring H), 7.61 (dd, 2H, J = 8.4, 2.4 Hz, benzene ring H), 7.67 (d, 2H, J = 2.4 Hz, benzene ring H); 13C NMR (CDCl3) δ -5.37, 15.93, 23.64, 122.61, 124.36, 126.77, 129.90, 130.37, 132.67, 136.16, 141.51, 145.61, 147.22; APCI Exact MS Calcd for C28H33S2Si2 [M+H+]: 489.15567, Found: 489.15561.
Compound 2b was prepared in a similar fashion to that above, using dibromo(tetrabutyldisilano)biphenyl instead of dibromo(tetramethyldisilano)biphenyl and the Pd2(dba)3 (5 mol%)/(o-Tol)3P (20 mol%)/CuO (1 eq) catalyst, as yellow solids in 55% yield after purification by silica gel column chromatography followed by recycling preparative GPC. In this reaction, the formation of a trace of the silole derivative, analogous to 1a was detected by DI-EI-MS analysis of the reaction mixture. Data for 2b: mp 81.5 ºC (DSC); EI-MS m/z 656 [M+]; 1H NMR (CDCl3) δ 0.80-0.85 (m, 20H, Bu), 1.22-1.37 (m, 16H, Bu), 1.36 (t, 6H, J = 7.6 Hz, Et), 2.88 (q, 4H, J = 7.6 Hz, Et), 6.79 (d, 2H, J = 3.6 Hz, thiophene ring H), 7.17 (d, 2H, J = 3.6 Hz, thiophene ring H), 7.41 (d, 2H, J = 8.4 Hz, benzene ring H), 7.60 (dd, 2H, J = 8.4, 2.0 Hz, benzene ring H),7.64 (d, 2H, J = 2.0 Hz, benzene ring H); 13C NMR (CDCl3) δ 11.11, 13.69, 15.97, 23.64, 26.55, 27.28, 122.44, 124.36, 126.57, 129.94, 130.72, 132.38, 135.19, 141.73, 146.28, 147.11; APCI Exact MS Calcd for C40H57S2Si2 [M+H+]: 657.34347, Found: 657.34320.
Preparation of Compound 3a. From a mixture of 7.8 mg (0.070 mmol) of trimethylamine oxide dihydrate and 30 mL of toluene was distilled approximately 20 mL of an azeotropic mixture of toluene/water to give an absolute trimethylamine oxide-toluene solution. The resulting solution of triethylamine oxide was added to a solution of 34.2 mg (0.070 mmol in total) of a 5:5 mixture of 2a and 3a in 50 mL and the resulting mixture was stirred at 50 °C for 6 h. After usual workup as above, the residue was subjected to recycling preparative GPC eluting with toluene to give 26.8 mg of compound 3a as colorless solids (76% yield from 2a). Data for 3a: mp 142.7-144.7 ºC; EI-MS m/z 504 [M+]; 1H NMR (CDCl3) δ -0.22 (s, 6H, SiMe), 0.58 (s, 6H, SiMe), 1.37 (t, 6H, J = 6.0 Hz, Et), 2.90 (qd, 4H, J = 6.0, 1.0 Hz, Et), 6.81 (dt, 2H, J = 3.5, 1.0 Hz, thiophene ring H), 7.21 (d, 2H, J = 3.5 Hz, thiophene ring H), 7.41 (d, 2H, J = 8.0 Hz, benzene ring H), 7.69 (dd, 2H, J = 8.0, 2.0 Hz, benzene ring H), 7.78 (d, 2H, J = 2.0 Hz, benzene ring H); 13C NMR (CDCl3) δ -0.67, 0.32, 15.96, 23.64, 122.82, 124.44, 126.94, 130.14, 130.98, 132.76, 138.55, 141.26, 147.48, 147.65; IR 977 cm-1 (Si-O-Si); APCI Exact MS Calcd for C28H33OS2Si2 [M+H+]: 505.15059, Found: 505.15015.
Preparation of Compound 4a. To a solution of 1.5 mg (20 mol%) of Pd(OAc)2, 2.8 mg (30 mol%) of 1,1,3,3-tetramethylbutyl isocyanide, 27.2 mg (3 eq) of 18-crown-6 in 1.0 mL of THF was added 16.7 mg (0.0342 mmol in total) of a 10:1 mixture of 2a and 1a, 19.1 mg (1.5 eq) of 2-trimethylsilylphenyl triflate, and 5.9 mg (3 eq) of KF at room temperature in that order. After the resulting mixture was stirred for 2.5 h at this temperature, the mixture was diluted with 10 mL of ethyl acetate and filtered to remove the resulting precipitates. After removal of the solvent, the residue was subjected to silica gel column chromatography eluting with hexane/ethyl acetate = 30/1, giving crude solids that were further purified by recycling preparative GPC eluting with toluene to afford 13.6 mg of compound 4a as a mixture with 3a in a ratio of 10:1 (71% yield for 4a from 2a). In this reaction, compound 1a was recovered unchanged. Data for 4a (as a 10:1 mixture with 3a): mp 161.0-170.4 ºC; EI-MS m/z 564 [M+]; 1H NMR (CDCl3) δ -0.59 (s, 6H, SiMe), 0.70 (s, 6H, SiMe), 1.33 (t, 6H, J = 7.5 Hz, Et), 2.85 (q, 4H, J = 7.5 Hz, Et), 6.75 (br d, 2H, J = 4.0 Hz, thiophene ring H), 7.13 (d, 2H, J = 4.0 Hz, thiophene ring H), 7.39-7.41 (m, 2H, benzene ring H), 7.41 (d, 2H, J = 8.0 Hz, benzene ring H), 7.63 (dd, 2H, J = 8.0 Hz, benzene ring H), 7.70 (dd, 2H, J = 6.0, 3.5 Hz, benzene ring H), 7.79 (d, 2H, J = 2.0 Hz, benzene ring H); 13C NMR (CDCl3) δ -0.41, 0.32, 15.94, 23.60, 122.77, 124.35, 126.35, 128.11, 128.99, 132.53, 133.21, 134.11, 138.11, 141.31, 146.61, 147.34, 149.44; APCI Exact MS Calcd for C34H37S2Si2 [M+H+]: 565.18697, Found: 565.18658.
Preparation of Polymers P2b. A mixture of 89.2 mg (0.15 mmol) of dibromotetrabutyldisilanobiphenyl, 6.9 mg (5 mol%) of Pd2(dba)3, 74.1 mg (1.00 eq) of bis(trimethylstannyl)bithiophene, 9.1 mg (20 mol%) of tri(o-tolyl)phosphine, and 10 mL of toluene was stirred at 80 °C for 12 h. The resulting mixture was diluted with 20 mL of chloroform and filtered to remove the resulting precipitates. After concentration of the filtrate to about 3 mL, the concentrated filtrate was poured to 50 mL of acetone to precipitate 68.0 mg of P2b as brown solids (85% yield), which were collected and dried under reduced pressure: Mn = 11,600, Mw/Mn = 4.44; 1H NMR (CDCl3) δ 0.35-1.74 (m, 36H, Bu), 6.84-7.80 (m, 10H, ring H).
Preparation of Polymer P3b. To a 20 mL toluene solution of trimethylamine oxide (1.2 eq) dried by azeotropic distillation (see the preparation of 3a) was added 11.0 mg of polymer P2b and the mixture was heated to reflux for 15 h. After hydrolytic workup as above, the residue was reprecipitated from chloroform/ethanol to give 6.1 mg of P3b as orange solids (54% yield): Mn = 8,700, Mw/Mn = 2.30; 1H NMR (CDCl3) δ 0.13-1.32 (m, 36H, Bu), 7.25 (br s, 2H, thiophene ring H), 7.32 (br s, 2H, thiophene ring H), 7.41 (d, 2H, J = 8.0 Hz, benzene ring H), 7.73 (br d, 2H, J = 8.0 Hz, benzene ring H), 7.80 (br s, 2H, benzene ring H); IR 974 cm-1 (Si-O-Si).
ACKNOWLEDGEMENT
This work was supported in part by a Grant–in–Aid for Scientific Research (No.23350097) from the Ministry of Education, Culture, Sports, Science and Technology, Japan.
References
1. (a) K. Müllen and U. Scherf, 'Organic Light-Emitting Devices, Wiley-VCH, Weinheim, 2006; (b) H. Klauk, 'Organic Electronics, Wiley-VCH, Weinheim, 2006. CrossRef
2. (a) K. L. Chan, M. J. AcKiernan, C. R. Towns, and A. B. Holmes, J. Am. Chem. Soc., 2005, 127, 7662; CrossRef (b) M. Shimizu and T. Hiyama, Synlett, 2012, 973; CrossRef (c) T. Ago, M. D. Hossain, and T. Kawashima, Chem. Eur. J., 2010, 16, 368; CrossRef (d) P.-L. T. Boudreault, A. Michaud, and M. Leclerc, Macromol. Rapid Commun., 2007, 28, 2176; CrossRef (e) E. Wang, L. Wang, L. Lan, C. Luo, W. Zhang, J. Pen, and Y. Cao, Appl. Phys. Lett., 2008, 92, 033307. CrossRef
3. (a) J. Chen and Y. Cao, Macromol. Rapid Commun., 2007, 28, 1714; CrossRef (b) J. Ohshita, Macrmol. Chem. Phys., 2009, 210, 1360; CrossRef (c) J. Ohshita, M. Nodono, H. Kai, T. Watanabe, A. Kunai, K. Komaguchi, M. Shiotani, A. Adachi, K. Okita, Y. Harima, K. Yamashita, and M. Ishikawa, Organometallics, 1999, 18, 1453; CrossRef (d) J. Ohshita, H. Kai, A. Takata, T. Iida, A. Kunai, N. Ohta, K. Komaguchi, M. Shiotani, A. Adachi, K. Sakamaki, and K. Okita, Organometallics, 2001, 20, 4800. CrossRef
4. H. Kai, J. Ohshita, S. Ohara, N. Nakayama, A. Kunai, I.-S. Lee, and Y.-W. Kwak, J. Organomet. Chem., 2008, 693, 3490. CrossRef
5. H. Yoshida, J. Ikadai, M. Shudo, J. Ohshita, and A. Kunai, J. Am. Chem. Soc., 2003, 125, 6638; CrossRef (b) H. Yoshida, J. Ikadai, M. Shudo, J. Ohshita, and A. Kunai, Organometallics, 2005, 24, 156. CrossRef