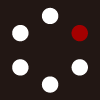
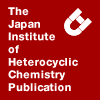
HETEROCYCLES
An International Journal for Reviews and Communications in Heterocyclic ChemistryWeb Edition ISSN: 1881-0942
Published online by The Japan Institute of Heterocyclic Chemistry
e-Journal
Full Text HTML
Received, 19th June, 2012, Accepted, 5th July, 2012, Published online, 17th July, 2012.
DOI: 10.3987/COM-12-S(N)39
■ HYPERVALENT IODINE INDUCED OXIDATIVE CROSS COUPLING VIA Thiophene CATION RADICAL INTERMEDIATE
Toshifumi Dohi, Motoki Ito, Sho Sekiguchi, Yohei Ishikado, and Yasuyuki Kita*
College of Pharmaceutical Sciences, Ritsumeikan University, 1-1-1 Nojihigashi, Kusatsu, Shiga, 525-8577, Japan
Abstract
The hypervalent iodine-induced arene cross coupling via a single- electron-transfer (SET) oxidation process could proceed in some extent even in heteroaromatic compounds, that is, several types thiophenes 1, using alkyl benzenes 2 as coupling partners. The structure of the obtainable heteroaromatic biaryl products could be elaborated by utilizing the known metal-catalyzed coupling technology, i.e., Negishi cross coupling, for the synthesis of multiple arylated (bi)thiophenes.The well-established cross coupling technologies using transition metal catalysts, such as the so-called Negishi coupling and Suzuki coupling,1 are the most promising strategies to form new aryl carbon- carbon bonds of required biary structures.2 The 1st generation cross couplings typically deal with extensive organic halides and organometallic compounds (e.g., metal = [Zn]; Negishi, [B]; Suzuki) as pre-functionalized and activated aryl substrates (Scheme 1, stepwise route). Recently, rapid evolution of this area has opened a new opportunity of cross couplings with non-functionalized substrates.3 One of them, the oxidative coupling between some unactivated aryl molecules, which only causes the loss of two protons from two coupling substrates, has become possible using transition metal catalysts with suitable stoichiometric terminal oxidants (Scheme 1, direct route).4 This method can avoid the stepwise route, and decreases the steps and amount of released metal wastes classically observed in the 1st generation couplings. Needless to say, transition metal catalysts, such as palladium, persists for aiding the reaction progress in the latter oxidative cross coupling method, while a metal catalyst-free system, that only uses an oxidant, providing a direct route to biaryls, is quite limited and still remains as an important challenge in modern biaryl synthesis.
Over the past decades, we have continually tackled the development of a new metal-catalyst-free strategy utilizing an unique oxidation ability of hypervalent iodine reagents for the direct coupling route to biaryls.5 Under suitable activation conditions, hypervalent iodine reagents show single-electron- transfer (SET) oxidation abilities toward various electron-rich aromatic rings.6,7 It was also revealed that oxidative couplings of electron-rich aromatic hydrocarbons, i.e., alkylbenzenes and phenyl ethers, smoothly proceed via their reactive aromatic cation radicals generated in situ by the action of PhI(OCOCF3)2 [phenyliodine(III) bis(trifluoroacetate), PIFA] with Lewis acids.8 Recently, we extended the strategy for the first time to the corresponding intermolecular cross coupling versions.9 The unprecedented formation of the cross coupling products of naphthalenes and alkylbenzenes would well explain the exclusive charge-transfer (CT) complexion of the naphthalene rings toward the suitably activated hypervalent iodine reagent (Scheme 2), from which subsequent generation of the naphthalene cation radicals should occur by the hypervalent iodine-induced SET oxidations. The nucleophilic alkylbenzenes, e.g., mesitylene,10 on the other hand, could attack the cationic intermediates, effectively producing the cross coupling products. We believe that the naphthalenes and the alkylbenzenes are thus regarded as indispensable aromatic cation radical sources and nucleophiles, respectively, for the successful cross couplings. Based on these considerations, the possibility of the SET oxidation strategy for the cross coupling for heteroaromatic compounds has not yet been fully investigated,11,12 though generation of heteroaromatic cation radicals has already been confirmed in the homocoupling studies.13
In this paper, we report the examinations and results of our cross coupling based on the SET oxidation strategy in heteroaromatic compounds, specifically, thiophenes 1. Actually, halothiophene 1a via its cation radical was first found to be a substrate for coupling with mesitylene 2a (Eq. 1). Using 3 equiv. of mesitylene 2a referred to the thiophene 1a, the desired cross coupling thiophene biaryl 3aa was formed in a moderate yield (56%) under the standard condition using PIFA (1 equiv.) and BF3·Et2O (2 equiv.) in dichloromethane (0.1 M concentration for the substrate 1a).9a However, we were also aware that the use of an excess amount of mesitylene 2a produced a significant amount of its homocoupling dimer (ca. 20% formation). Thus, the reaction was re-tested using a lower amount of mesitylene 2a (1.5 equiv.), but the yield of the coupling product 3aa decreased to 40%. Unfortunately, screening of the concentration, temperature, and solvent for the reaction resulted in no considerable improvement in the ratio of the cross coupling product 3aa versus the mesitylene homocoupling dimer. FPIFA (pentafluorophenyliodine(III) bis(trifluoroacetate), C6F5I(OCOCF3)2) was apparently an effective reagent for inducing the biaryl coupling reaction,9b and indeed could provide the two products, 3aa (63%) and the mesitylene homodimer (33%), in a 96% total yield, while the selectivity decreased to 66 : 34 ratio (3aa : homodimer) similar to the previous study in mesitylene 2a.9a
In spite of the low reaction yield, other alkylbenzene 2b could partially produce the cross coupling product 3ab by the reaction of the halothiophene 1a under the standard conditions at -40 oC (Eq. 2). In comparison, the homocoupling problem was rather suppressed in the sterically hindered alkylbenzene 2b. Indeed, the formation of very small amount (<5%) of homodimer was observed. Similarly, the alkylthiophene 1b was coupled with mesitylene 2a, albeit in a lower product yield than in the case of the halothiophene 1a (Eq. 3).
It is known that multiple arylated thiophenes are interesting synthetic targets being valuable in modern organic chemistry.14 Inspired by this and expand the range of the obtainable thiophene biaryl products 3, our coupling strategy should be utilized along with the well-known metal-catalyzed coupling technologies. Thus, we prepared 3-arylthiophene 1c from the halothiphene 1a by the standard Negishi coupling,15a and examined it for the hypervalent iodine-induced oxidative cross coupling in order to obtain a diarylated thiophene product (Scheme 3). Consequently, the thiophene 1c containing a phenyl group has become a possible entry to our cross coupling strategy, and the two coupling sequences, i.e., the Negishi coupling followed by our metal-free coupling, could provide the diarylthiophene 3ca in only two steps from the halothiophene 1a.
Notably, bithiophenes have appeared as acceptable substrates, whose successes in oxidative coupling are very rare and quite specific in our method (Scheme 4). One example is that the metal-free coupling method was compatible with the alkylated bithiophene 1d15b to give a linear teraryl compound 3da (Eq. 4). The more electron-rich oxygenated bithiophene 1e, prepared by our coupling method,12 could also couple with pentamethylbenzene 2c to give a linear teraryl compound 3ec (Eq. 5). The oxygenated thiophene dimers and oligomers obtained in such a sequence have been found to be new applications as important structural units of several low band gap organic materials.16 Due to the lower oxidation potential of bithiophenes compared to the corresponding thiophene monomers, the oxidation-sensitive bithiophene 1e should be the most problematic substrate to control its reaction in other oxidative coupling methods. Thus, the hypervalent iodine-used case in Eq. 5 is, to our knowledge, the only successful example to date of the oxygenated bithiophene being supplied as a successful substrate in an oxidative cross coupling reaction.
In summary, we have evaluated in this paper a series of halo-, alkyl-, aryl-, and bi-thiophenes 1a-e, as useful heteroaromatic coupling substrates in the cross coupling with mesitylene 2a and its analogues via the SET oxidation processes using hypervalent iodine reagents. The obtainable aryl-aryl linked systems in our coupling method should be further expanded when combined with the known Negishi cross coupling technology for the expeditious access to multiple arylated thiophenes.
EXPERIMENTAL
Melting points (mp) are uncorrected. All 1H- and 13C-NMR spectra of the products were measured in CDCl3. Chemical shifts of 1H-NMR were recorded in parts per million (ppm, δ) relative to tetramethyl silane (δ = 0.00 ppm) as an internal standard. Data are reported as follows: chemical shift in ppm (δ), multiplicity (s = singlet, d = doublet, sep = septet, m = multiplet), coupling constant (J) in Hz, and integration. Chemical shifts of 13C-NMR were reported in ppm with the solvent as reference peak (CDCl3: δ = 77.0 ppm). Infrared spectra (IR) were recorded by using a Hitachi 270-50 spectrometer; intensities of absorptions are reported in reciprocal centimeters (cm-1). High resolution mass measurements and elemental analysis were performed by the Elemental Analysis Section of Osaka University and the Analysis Section of Osaka University of Pharmaceutical Sciences. Flash column chromatography was performed with Merck Silica Gel 60 (230-400 mesh) eluting with hexane and ethyl acetate. Thin-layer chromatography (TLC) was performed on Merk Silica Gel F254 plates (0.25 mm). The spots and bands were detected by UV light of irradiation (254, 365 nm).
Materials
Hypervalent iodine reagents, PhI(OCOCF3)2 (PIFA) and C6F5I(OCOCF3)2 (FPIFA), are commercially available. Trimethylthiophene 1b was prepared according to literature.17 All other chemicals and solvents for the experiments and chromatography were obtained from commercial suppliers, and used as received.
General procedure for the cross coupling reaction of thiophenes 1 using hypervalent iodine reagent
To a stirred solution of iodothiophene 1a (1.04 g, 5 mmol) and alkylbenzene nucleophile 2a (1.80 g, 15 mmol, 3 equiv.) in CH2Cl2 (0.1 M referred to 1a) was successively added BF3·Et2O (1.27 mL, 10 mmol, 2 equiv.) and PIFA (2.15 g, 5 mmol, 1 equiv.) under a nitrogen atmosphere at -40 °C. The reaction mixture was stirred for 3 h at the same temperature, then quenched with a saturated aqueous NaHCO3 solution. After extraction using CH2Cl2, the obtained organic layer was dried over anhydrous Na2SO4. After filtration, removal of the solvent in vacuo followed by column chromatography of the residue gave the pure cross coupling product, the thiophene biaryl 3aa (0.92 g, 2.8 mmol), in 56% yield.
3-Iodo-2-(2,4,6-trimethylphenyl)thiophene (3aa)
Colorless crystals; mp 120-122 °C; IR (KBr): 3101, 2914, 2729, 1735, 1608, 1473, 1440, 1373, 1334, 1224, 1136, 1031, 968, 910, 852, 790, 734, 715, 640, 553 cm-1; 1H-NMR (300 MHz, CDCl3): 2.04 (s, 6H), 2.33 (s, 3H), 6.95 (s, 2H), 7.14 (d, J = 5.1 Hz, 1H), 7.33 (d, J = 5.1 Hz, 1H) ppm; 13C-NMR (67.8 MHz, CDCl3): 20.3, 21.2, 81.7, 127.4, 128.2, 130.5, 134.9, 138.2, 138.6, 142.1 ppm; HRMS (FAB): Calcd for C13H13IS (M)+: 327.9783, Found 327.9794.
3-Iodo-2-[2,4,6-tris(propan-2-yl)phenyl]thiophene (3ab)
Colorless crystals; mp 82-83 °C; IR (KBr): 2959, 1606, 1458, 1382, 1361, 911, 877, 856, 743, 706, 655 cm-1; 1H-NMR (400 MHz, CDCl3): 1.09 (d, J = 6.8 Hz, 6H), 1.21 (d, J = 6.8 Hz, 6H), 1.29 (d, J = 6.8 Hz, 6H), 2.59 (sep, J = 6.8 Hz, 2H), 2.96 (sep, J = 6.8 Hz, 1H), 7.04 (s, 2H), 7.13 (d, J = 5.4 Hz, 1H), 7.31 (d, J = 5.4 Hz, 1H) ppm; 13C-NMR (100 MHz, CDCl3): 24.1, 25.1, 30.7, 30.9, 34.5, 83.5, 121.2, 127.3, 128.2, 134.9, 142.4, 148.9, 150.0 ppm.
Trimethyl-2-(2,4,6-trimethylphenyl)thiophene (3ba)
Colorless crystals; mp 73-75 °C; IR (KBr): 2920, 2856, 1454, 1377, 912, 742, 711 cm-1; 1H-NMR (300 MHz, CDCl3): 1.77 (s, 3H), 2.05 (s, 9H), 2.31 (s, 3H), 2.36 (s, 3H), 6.91 (s, 2H) ppm; 13C-NMR (67.8 MHz, CDCl3): 12.7, 13.1, 13.3, 20.4, 21.0, 127.9, 130.9, 131.0, 132.6, 133.9, 137.3, 138.6 ppm; Anal. Calcd for C16H20S: C, 78.63; H, 8.25; S, 13.12. Found C, 78.79; H, 8.28; S, 12.90.
3-Phenyl-2-(2,4,6-trimethylphenyl)thiophene (3ca)
Colorless crystals; mp 52-53 °C; IR (KBr): 2915, 2366, 1601, 1496, 1438, 1375, 1211, 1027, 877, 849, 769, 722, 694, 670, 658, 648 cm-1; 1H-NMR (400 MHz, CDCl3): 2.08 (s, 6H), 2.36 (s, 3H), 6.94 (s, 2H), 7.21-7.25 (m, 5H), 7.37 (d, J = 4.0 Hz, 1H), 7.45 (d, J = 4.0 Hz, 1H) ppm; 13C-NMR (100 MHz, CDCl3): 21.5, 22.1, 125.7, 127.5, 128.3, 129.2, 129.3, 129.4, 131.3, 137.4, 137.7, 138.8, 139.2, 139.3 ppm; HRMS (EI): Calcd for C19H18S (M)+: 278.1129, Found 278.1124.
3,3’-Dimethyl-5-(2,4,6-trimethylphenyl)-2,2’-bithiophene (3da)
Colorless oil; IR (KBr): 2920, 2856, 1446, 1377, 848, 833, 709 cm-1; 1H-NMR (300 MHz, CDCl3): 2.19 (s, 9H), 2.22 (s, 3H), 2.31 (s, 3H), 6.60 (s, 1H), 6.91 (d, J = 4.8 Hz, 1H), 6.93 (s, 2H), 7.26 (d, J = 4.8 Hz, 1H) ppm; 13C-NMR (67.8 MHz, CDCl3): 14.9, 15.0, 20.7, 20.8, 21.1, 124.6, 127.9, 129.3, 129.9, 130.8, 136.0, 136.1, 137.6, 138.0, 140.8 ppm; Anal. Calcd for C16H20S: C, 73.03; H, 6.45. Found C, 73.20; H, 6.65.
3,3’,4,4’-Tetramethoxy-5-pentamethylphenyl-2,2’-bithiophene (3ec)
Pale-yellow crystals; mp 115-120 °C; IR (KBr): 3111, 2933, 1556, 1520, 1477, 1388, 1308, 1276, 1255, 1204, 1156, 1127, 1088, 1066, 1037, 997, 961, 750, 695 cm-1; 1H-NMR (400 MHz, CDCl3): 2.16 (s, 6H), 2.23 (s, 6H), 2.36 (s, 3H), 3.57 (s, 3H), 3.83 (s, 3H), 3.85 (s, 3H), 3.94 (s, 3H), 6.09 (s, 1H) ppm; 13C-NMR (100 MHz, CDCl3): 16.7, 16.9, 18.5, 57.2, 59.1, 59.9, 60.1, 94.2, 116.3, 117.9, 120.6, 129.3, 132.4, 134.2, 135.5, 142.3, 144.6, 145.0, 150.4 ppm; HRMS (FAB): Calcd for C23H28O4S2 (M)+: 432.1249, Found 432.1434.
ACKNOWLEDGEMENTS
This work was supported by a Grant-in-Aid for Scientific Research (A) and Encouragement of Young Scientists (A) from JSPS, a Grant-in-Aid for Scientific Research on Innovative Areas “Advanced Molecular Transformations by Organocatalysts” from MEXT, and Ritsumeikan Global Innovation Research Organization (R-GIRO) project. T.D. also acknowledges financial support from the Asahi Glass Foundation and the Industrial Technology Research Grant Program from NEDO of Japan. M.I. thanks support from the Research Activity Start-up of the JSPS.
References
1. (a) E. Negishi, Angew. Chem. Int. Ed. (Nobel Lecture), 2011, 50, 6738; CrossRef (b) A. Suzuki, Angew. Chem. Int. Ed. (Nobel Lecture), 2011, 50, 6722; CrossRef (c) Metal-Catalyzed Cross-Coupling Reactions; F. Diederich and P. J. Stang, Eds.; Wiley-VCH: New York, 2004.
2. (a) R. Noyori, Chem. Soc. Rev., 1989, 18, 187; CrossRef (b) K. Narasaka, Synthesis, 1991, 1; CrossRef (c) G. Bringmann, R. Walter, and R. Weirich, Angew. Chem., Int. Ed. Engl., 1990, 29, 977; CrossRef (d) G. M. Keserú and M. Nógrádi, In Studies in Natural Products Chemistry; Atta-ur-Rahman, Ed.; Elsevier: Amsterdam, 1998; Vol. 20, pp 263; (e) I. Cepanec, Synthesis of Biaryls, Elsevier: New York, 2004.
3. (a) G. P. McGlacken and L. M. Bateman, Chem. Soc. Rev., 2009, 38, 2447; CrossRef (b) J. A. Ashenhurst, Chem. Soc. Rev., 2010, 39, 540; CrossRef (c) C. S. Yeung and V. M. Dong, Chem. Rev., 2011, 111, 1215. CrossRef
4. (a) D. R. Stuart and K. Fagnou, Science, 2007, 316, 1172; CrossRef (b) D. R. Stuart, E. Villemure, and K. Fagnou, J. Am. Chem. Soc., 2007, 129, 12072; CrossRef (c) T. A. Dwight, N. R. Rue, D. Charyk, R. Josselyn, and B. DeBoef, Org. Lett., 2007, 9, 3137; CrossRef (d) S. H. Cho, S. J. Hwang, and S. Chang, J. Am. Chem. Soc., 2008, 130, 9254; CrossRef (e) P. Xi, F. Yang, S. Qin, D. Zhao, J. Lan, G. Gao, C. Hu, and J. You, J. Am. Chem. Soc., 2010, 132, 1822; CrossRef (f) C.-Y. He, S. Fan, and X. Zhang, J. Am. Chem. Soc., 2010, 132, 12850; CrossRef (g) Z. Wang, K. Li, D. Zhao, J. Lan, and J. You, Angew. Chem. Int. Ed., 2011, 50, 5365. CrossRef
5. For our recent accounts, see: (a) Y. Kita, T. Dohi, and K. Morimoto, J. Synth. Org. Chem. Jpn., 2011, 69, 1241; CrossRef (b) Y. Kita, T. Dohi, and K. Morimoto, Kagaku, 2011, 66, 12; (c) Y. Kita and T. Dohi, Fain Kemikaru, 2011, 40, 49; (d) Y. Kita and T. Dohi, Shokubai, 2012, 54, 145.
6. Original paper: (a) Y. Kita, H. Tohma, K. Hatanaka, T. Takada, S. Fujita, S. Mitoh, H. Sakurai, and S. Oka, J. Am. Chem. Soc., 1994, 116, 3684; CrossRef Accounts: (b) Y. Kita, T. Takada, and H. Tohma, Pure Appl. Chem., 1996, 68, 627; CrossRef (c) T. Dohi, M. Ito, N. Yamaoka, K. Morimoto, H. Fujioka, and Y. Kita, Tetrahedron, 2009, 65, 10797. CrossRef
7. Selected recent reviews of hypervalent iodine reagents: (a) T. Wirth, M. Ochiai, V. V. Zhdankin, G. F. Koser, H. Tohma, and Y. Kita, in Top. Curr. Chem.: Hypervalent Iodine Chemistry Modern Developments in Organic Synthesis, T. Wirth (ed.), Springer-Verlag, Berlin (2003); (b) V. V. Zhdankin and P. J. Stang, Chem. Rev., 2008, 108, 5299; CrossRef (c) T. Dohi and Y. Kita, Chem. Commun., 2009, 2073; CrossRef (d) L. F. Silva, Jr. and B. Olofsson, Nat. Prod. Rep., 2011, 28, 1722; CrossRef (e) V. V. Zhdankin, J. Org. Chem., 2011, 76, 1185. CrossRef
8. (a) Y. Kita, M. Gyoten, M. Ohtsubo, H. Tohma, and T. Takada, Chem. Commun., 1996, 1481; CrossRef (b) T. Takada, M. Arisawa, M. Gyoten, R. Hamada, H. Tohma, and Y. Kita, J. Org. Chem., 1998, 63, 7698; CrossRef (c) H. Tohma, H. Morioka, S. Takizawa, M. Arisawa, and Y. Kita, Tetrahedron, 2001, 57, 345; CrossRef (d) H. Tohma, M. Iwata, T. Maegawa, and Y. Kita, Chem. Eur. J., 2002, 8, 5377. CrossRef
9. (a) T. Dohi, M. Ito, K. Morimoto, M. Iwata, and Y. Kita, Angew. Chem. Int. Ed., 2008, 47, 1301; CrossRef (b) T. Dohi, M. Ito, I. Itani, N. Yamaoka, K. Morimoto, H. Fujioka, and Y. Kita, Org. Lett., 2011, 13, 6208. CrossRef
10. Polyalkyl-substituted benzenes were estimated to possess higher nucleophilicities than naphthalene: (a) H. Mayr and A. R. Ofial, Angew. Chem., Int. Ed. Engl., 1992, 31, 1613; CrossRef (b) H. Mayr and M. Patz, Angew. Chem., Int. Ed. Engl., 1994, 33, 938. CrossRef
11. Cross coupling of heteroaromatic compounds via diaryliodonium bromides were reported: (a) Y. Kita, K. Morimoto, M. Ito, C. Ogawa, A. Goto, and T. Dohi, J. Am. Chem. Soc., 2009, 131, 1668; CrossRef (b) T. Dohi, M. Ito, N. Yamaoka, K. Morimoto, H. Fujioka, and Y. Kita, Angew. Chem., Int. Ed., 2010, 49, 3334; CrossRef (c) K. Morimoto, N. Yamaoka, C. Ogawa, T. Nakae, H. Fujioka, T. Dohi, and Y. Kita, Org. Lett., 2010, 12, 3804. CrossRef
12. The methods in ref. 11 are also applicable for the bithiophene synthesis: K. Morimoto, T. Nakae, N. Yamaoka, T. Dohi, and Y. Kita, Eur. J. Org. Chem., 2011, 6326. CrossRef
13. (a) H. Tohma, M. Iwata, T. Maegawa, Y. Kiyono, A. Maruyama, and Y. Kita, Org. Biomol. Chem., 2003, 1, 1647; CrossRef (b) T. Dohi, K. Morimoto, Y. Kiyono, A. Maruyama, H. Tohma, and Y. Kita, Chem. Commun., 2005, 2930. CrossRef
14. For known synthetic methods and utility of multiple arylated thiophenes, see: A. Rahimi, J. C. Namyslo, M. H. H. Drafz, J. Halm, E. Huebner, M. Nieger, N. Rautzenberg, and A. Schmidt, J. Org. Chem., 2011, 76, 7316 and references cited therein. CrossRef
15. Synthesis of 3-phenylthiophene 1c by the Negishi coupling: (a) R. M. S. Maior, K. Hinkelmann, H. Eckert, and F. Wudl, Macromolecules, 1990, 23, 1268; CrossRef bithiophene 1d synthesis: (b) M. Takahashi, K. Masui, H. Sekiguchi, N. Kobayashi, A. Mori, M. Funahashi, and N. Tamaoki, J. Am. Chem. Soc., 2006, 128, 10930. CrossRef
16. (a) P. Amaladass, J. A. Clement, and A. K. Mohanakrishnan, Tetrahedron, 2007, 63, 10363 and references therein; CrossRef (b) A. Borghese, G. Geldhof, and L. Antoine, Tetrahedron Lett., 2006, 47, 9249. CrossRef
17. B. F. Crowe and F. F. Nord, J. Org. Chem., 1950, 15, 1177. CrossRef