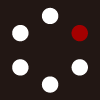
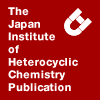
HETEROCYCLES
An International Journal for Reviews and Communications in Heterocyclic ChemistryWeb Edition ISSN: 1881-0942
Published online by The Japan Institute of Heterocyclic Chemistry
e-Journal
Full Text HTML
Received, 6th June, 2012, Accepted, 17th July, 2012, Published online, 23rd July, 2012.
DOI: 10.3987/COM-12-S(N)28
■ Nickel-Catalyzed Borylation of Aryl Halides with 4,4,6-Trimethyl-1,3,2-dioxaborinane
Miki Murata,* Yosuke Sogabe, Takeshi Namikoshi, and Shinji Watanabe
Department of Materials Science and Engineering, Kitami Institute of Technology, Koen-cho 165, Kitami, Hokkaido 090-8507, Japan
Abstract
The nickel-catalyzed borylation of aryl iodides and bromides with 4,4,6-trimethyl-1,3,2-dioxaborinane was achieved. The mild reaction conditions employed allowed for the inclusion of common functional groups in aryl halides to be tolerated. A DFT study on the catalytic cycle shows that C–B bond formation occurs through σ-bond metathesis between dialkoxyborane and arylnickel(II) halide intermediates.Arylboronic acids and their esters are an important class of organometallics, which can be widely used as versatile building blocks in modern organic synthesis particularly with reactions involving carbon–carbon bond formation through the Suzuki–Miyaura cross-coupling.1 As the versatility of arylboronates has made them attractive targets for synthesis, the development of transition metal-catalyzed aryl C–B bond-forming reactions has attracted considerable interest.2 Above all, the borylation of aryl electrophiles with pinacolborane have proved to be an atom-economical and powerful method for preparation of functionalized arylboronates.3 We have demonstrated that 4,4,6-trimethyl-1,3,2-dioxaborinane (1a), which is more cost-effective than pinacolborane, is an alternative boron source for the catalytic borylation of aryl electrophiles. The palladium complex coordinated with bis(2-di-tert-butylphosphinophenyl)ether can catalyze the reaction of aryl iodides with 1a to afford the corresponding arylboronates in high yields.4 However, this work was limited to aryl iodides and used the poorly available and air-sensitive phosphine ligand. After our investigation, the Chavant group has achieved the borylation of aryl iodides and bromides with 1a by using the palladium catalyst coordinated with PPh3 or Buchwald biaryl phosphine ligands, but these conditions resulted in somewhat low yields when aryl halides possesses electron- withdrawing groups.5 We have also achieved the coupling of aryl arenesulfonates with 1a by using Bu4NI and a catalytic amount of Pd(dba)2/1,1'-bis(di-tert-butylphosphino)ferrocene.6 Whereas the scope of the aryl electrophiles in the palladium-catalyzed borylation with 1a has been improved, the reaction of electron-deficient aryl bromides still had some drawbacks. In this paper, we wish to report a practical protocol for the nickel-catalyzed borylation of aryl iodides and bromides 2 using 1a (Scheme 1). Recently, we have demonstrated that the borylation of electron-deficient aryl chlorides was achieved by the use of NiCl2(dppp) catalyst with the aid of Bu4NBr.7
As a test for the optimization of catalyst systems, ethyl 4-bromobenzoate (2a) was used as a substrate for the borylation using 1a. The results are summarized in Table 1. The treatment of 2a with 1a (1.5 equiv) and Et3N (3 equiv) with 5 mol% of NiCl2(dppp) in toluene at 100 °C was found to lead to the corresponding arylboronate 3a in a good yield (entry 1). However, the reaction suffered from small
amounts of dehalogenated arene byproducts 4a by partially reduction of the C–Br bond. We were pleased to observe that the use of additional phosphine as a co-ligand improved the yield and selectivity (entries 2–4). Recently, Percec reported that the mixed-ligand systems provided highly active catalysts for the nickel-catalyzed borylation using in situ-prepared neopentylglycolborane.8 As in the case of the reaction with neopentylglycolborane, DPPF above all other phosphines completely suppressed the formation of 4a
(entry 4). Additionally, NiCl2(dppf)-based catalyst systems provided poor yields, indicating that the ligand exchange was limited and that the benefit of DPPF was achieved only as a co-ligand (entries 5–7).8 Unfortunately, we still have no definitive explanation for the role of co-ligands.
With the optimized conditions in hand, we next investigated the substrate scope of the borylation of the aryl iodides and bromides 2 using 1a (Table 2).9 The presence of functional groups, such as not only ester (Table 1) but also ketone carbonyl (Table 2, entry 1) and cyano groups (entries 2 and 4), in the starting aryl bromides 2 (X = Br) did not interfere with the outcome of the borylation with 1a. Unfortunately, an electron-donating (entry 8) or ortho substituent (entry 10) on the aromatic ring of 2 (X = Br) prevented the present reaction. In contrast to aryl bromides, the differences in the yields among iodide counterparts 2 (X = I) possessing electron-donating or -withdrawing groups were not particularly large (entries 3 and 9). Also, the yields of 3 did not depend on the steric requirement of aryl iodides (entry 11).
Our focus then turned to the mechanism of this nickel-catalyzed borylation. The proposed catalytic cycle was computationally addressed by density functional theory (DFT) calculations (Figure 1).9 We have adopted the reaction of bromobenzene (2m) with 1,3,2-dioxaborolane (1b) as a model reaction, and the DPPP ligand was replaced by 1,2-diphosphinopropane (DHPP). Recently, Marder and Lin reported that the palladium-catalyzed borylation involves σ-bond metathesis between hydroboranes and a cationic [L2Pd(Ar)]+ species as the B–C bond-forming step.10 Our supposition is that the present mechanism involves the oxidative addition of 2m to Ni(0) 5 to form (η2-dhpp)Ni(Ph)(Br) 6;11 however, our calculations indicate that the barrier of the similar charge-separated pathway for the
nickel-catalyzed borylation is significantly large even with inclusion of the solvation energies. Thus, 6 undergoes partial ligand dissociation to afford (η1-dhpp)Ni(Ph)(Br) 7. After the coordination of 1b to 7, the σ-complex-assisted metathesis (σ-CAM) with the Ni–Ph bond takes place through a transition state TS8–9, in which the boron atom is pointing toward the aryl ligand, to form the desired borylation product and a nickel(II) hydride 10. The activation barrier of the σ-CAM is 15.6 kcal/mol, indicating that this neutral pathway is favored over the cationic one. Finally, deprotonation of 10 would regenerate the Ni(0) active species 5 with the aid of Et3N.
In conclusion, the combination of NiCl2(dppp) and DPPF was found to catalyze the borylation of aryl iodides and bromides using 4,4,6-trimethyl-1,3,2-dioxaborinane (1). Theoretical calculations suggest that the catalytic cycle involves the σ-bond metathesis between the Ni–C bond of neutral arylnickel(II) halide intermediate and the B–H bond of hydroborane. Further studies are currently underway to obtain detailed mechanistic insights.
ACKNOWLEDGEMENTS
This work was partially supported by a Grant-in-Aid for Scientific Research from Japan Society for Promotion in Science (JSPS).
References
1. N. Miyaura, 'Metal-Catalyzed Cross-Coupling Reactions, Second Edition,' ed. by A. de Meijere and F. Diederich, Wiley-VCH, Weinheim, 2004, pp. 41-123. CrossRef
2. T. Ishiyama and N. Miyaura, 'Boronic Acids, Second, Completely Revised Edition,' ed. by D. G. Hall, Wiley-VCH, Weinheim, 2011, pp. 135-170. CrossRef
3. M. Murata, Heterocycles, 2012, 85, 1795. CrossRef
4. M. Murata, T. Oda, S. Watanabe, and Y. Masuda, Synthesis, 2007, 351. CrossRef
5. (a) N. PraveenGanesh and P. Y. Chavant, Eur. J. Org. Chem., 2008, 4690; CrossRef (b) N. PraveenGanesh, E. Demory, C. Gamon, V. Blandin, and P. Y. Chavant, Synlett, 2010, 2403. CrossRef
6. M. Murata, T. Oda, Y. Sogabe, H. Tone, T. Namikoshi, and S. Watanabe, Chem. Lett., 2011, 40, 962. CrossRef
7. M. Murata, T. Sambommatsu, T. Oda, S. Watanabe, and Y. Masuda, Heterocycles, 2010, 80, 213. CrossRef
8. (a) C. Moldoveanu, D. A. Wilson, C. J. Wilson, P. Corcoran, B. M. Rosen, and V. Percec, Org. Lett., 2009, 11, 4974; CrossRef (b) C. Moldoveanu, D. A. Wilson, C. J. Wilson, P. Leowanawat, A. M. Resmerita, C. Liu, B. M. Rosen, and V. Percec, J. Org. Chem., 2010, 75, 5438; CrossRef (c) P. Leowanawat, A. M. Resmerita, C. Moldoveanu, C. Liu, N. Zhang, D. A. Wilson, L. M. Hoang, B. M. Rosen, and V. Percec, J. Org. Chem., 2010, 75, 7822; CrossRef (d) D. A. Wilson, C. J. Wilson, C. Moldoveanu, A. M. Resmerita, P. Corcoran, L. M. Hoang, B. M. Rosen, and V. Percec, J. Am. Chem. Soc., 2010, 132, 1800. CrossRef
9. Supporting Information is available on the Heterocycles web site, http://www.heterocycles.jp/.
10. K. C. Lam, T. B. Marder, and Z. Lin, Organometallics, 2010, 29, 1849. CrossRef
11. Treatment of Ni(Ar)(Br)(PPh3)2 (Ar = 4-EtO2C-C6H4) with DPPP (1 equiv) and 1a (20 equiv) was found to lead to the corresponding arylboronate 3a.