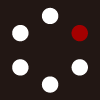
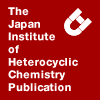
HETEROCYCLES
An International Journal for Reviews and Communications in Heterocyclic ChemistryWeb Edition ISSN: 1881-0942
Published online by The Japan Institute of Heterocyclic Chemistry
e-Journal
Full Text HTML
Received, 23rd June, 2012, Accepted, 18th July, 2012, Published online, 31st July, 2012.
DOI: 10.3987/COM-12-S(N)51
■ Synthesis of 15,28-Dideoxy-15,28-didehydrothyrsenol
Mary A. Smart and Frank E. McDonald*
Department of Chemistry, Emory University, 1515 Dickey Drive, Atlanta, GA 30322, U.S.A.
Abstract
The tetracyclic skeleton of thyrsenols A and B has been assembled, with the construction of ring A by exo-selective oxacyclization of an epoxybromohydrin, B ring formation by exo-selective oxacyclization of hydroxyepoxide followed by solvolytic ring expansion of a tetrahydrofuran to tetrahydropyran, C ring closure by tungsten-promoted endo-selective oxacyclization of a hydroxyalkyne, and D ring preparation by asymmetric dihydroxylation of an epoxyalkene accompanied by exo-selective oxacyclization. A modified Stille coupling linked two fragments at C14-C15 to directly provide the tetracyclic dienylether.INTRODUCTION
Marine polyether triterpenes encompass a large class of natural products arising from oxidative cyclizations of squalenes, exhibiting various arrangements of five, six, and even seven-membered ring ethers.2 Pharmacologically many of these natural products display antitumor, antiinflammatory, analgesic, antiallergy, and antiviral properties, and have been the subject of numerous biological studies. In particular, thyrsiferol (1, Scheme 1) and especially thyrsiferyl 23-acetate (2) exhibit powerful inhibition of cell growth against the P388 lymphoid cancer cell line,3 whereas the diastereomeric natural product venustatriol (3) shows activity against vesicular stomatitis virus (VSV) and Herpes simples virus type 1 (HSV-1),4 and the structurally unique dioxepandehydrothyrsiferol (4) bearing two fused seven-membered rings is apparently inactive against several tumor cell lines.5 Thyrsenols A and B (5, 6) represent more highly oxidized congeners of thyrsiferol, with thyrsenol B displaying much greater cytotoxicity against than P388 cell line (IC50 = 0.016 µM) vs. thyrsenol A (IC50 = 0.40 µM).6
As part of our long-standing interest in the synthesis of marine polycyclic ethers including polyether triterpenes,7 we set out to explore the preparation of thyrsiferol or one of its congeners (2 - 6) by regioselective and stereospecific epoxide cyclizations.8 The total syntheses of thyrsiferols (1, 2), venustatriol (3), and dioxepandehydrothyrsiferol (4) have been achieved by several different laboratories,9-12 but to date synthetic activity directed towards the thyrsenols (5, 6) has not been reported. In considering the synthesis of thyrsenols A and B (5, 6), we proposed that late-stage regio- and enantioselective dihydroxylation of the dideoxydidehydrothyrsenol structure 7 would follow cross-coupling of a tricyclic enol ether derivative 8 with tetrahydrofuranyl-containing 9, with these sectors arising from oxidative cyclization synthetic strategies beginning with farnesol (10) and geraniol (11), respectively (Scheme 2).
RESULTS AND DISCUSSION
Our synthesis began with regioselective preparation of the racemic bromohydrin 13 (Scheme 3) from farnesyl acetate (12) as described in the literature,13 followed by regioselective and enantioselective epoxidation utilizing the Shi ketone,14 in which the alkene bearing the allylic acetate substituent was electronically deactivated for electrophilic epoxidation.15 As the Shi epoxidation required a buffer system with pH 10.5, these basic reaction conditions slowly converted the bromohydrin moiety into the undesired terminal epoxide, which was minimized only by diminishing the reaction time and thus decreasing the conversion of 13 into monoepoxide 14. Although protective groups could be introduced onto the tertiary alcohol of 13,16 the undesired epoxide formed upon removal of these protective groups, so in the end we judged that our original procedure of acid-catalyzed cyclization with careful control of temperature and conversion provided effective throughput of material into the desired bromotetrahydropyran diastereomer 15 as the result of kinetic resolution of the diastereomers of 14.17
The principal challenge in constructing the B ring of thyrsenols was achieving the 6-endo-tet cyclization of an epoxyalcohol instead of the 5-exo-tet cyclization predicted by Baldwin's rules.18 Instead, we elected to undertake a reaction sequence in which the 5-exo-tet cyclization tetrahydrofuranyl alcohol product would subsequently undergo isomerization to give the ring-expanded tetrahydropyranyl alcohol. The Sharpless asymmetric epoxidation19 was conducted from the triethylsilyl ether derivative 17, as the reaction rates and yields were diminished when the epoxidation was attempted with the corresponding diol. The triethylsilyl ether 16 was preferred over other silyl ethers for its wider range of pH stability and reduced tendency towards migration (Scheme 4). Upon deprotection of the silyl ether from the epoxidation product 18, the tetrahydrofuranyl diol 19 was selectively produced in excellent yield. As a model system for the ring expansion step, the primary alcohol was regioselectively converted into the acetate ester 20,20 and then the remaining secondary alcohol was transformed to the chloromesylate 21. Following optimized conditions developed by the Nakata laboratory, upon heating compound 21 in the presence of water and zinc acetate, ring expansion provided the desired tetrahydropyran as a mixture of acetate 23 and diol 24.21,22 As the mechanism for the Nakata rearrangement involved initial generation of epoxonium ion 22 followed by addition of water at the tertiary C10, single inversion of each chiral center at C10 and C11 was anticipated to provide the stereochemistry depicted for compounds 23 and 24.
With the success of the model system, we incorporated the carbon atoms necessary for C-ring synthesis by converting the diol 19 into epoxide 26 (Scheme 5) via intermediacy of the primary tosylate 25,23 followed by addition of lithium acetylide to provide compound 27.24 At this stage, Nakata rearrangement converted the chloromesylate derivative 28 into the tetrahydropyranyl alcohol 29, albeit in lower yield than we had observed in the model system in which the primary acetate had been present. Nonetheless, basic methanolysis of the trimethylsilyl group revealed the terminal alkyne of 30. The C-ring was then formed by endo-selective alkynol cyclization in the presence of stoichiometric tungsten carbonyl to a cyclic Fischer oxacarbene,25 followed by reaction with triethylamine and tributyltin triflate to install the tributyltin substituent in tricyclic compound 31 for subsequent Stille cross-coupling.
Several methods were explored for preparation of the D-ring synthon before we settled on the route depicted (Scheme 6), in which the known epoxytetrahydrofuranyl alcohol 35 was prepared in four steps from geraniol (11).26 Although the literature precedent had shown that asymmetric dihydroxylation27 of the epoxytosylate 33 with stirring for three days directly afforded the epoxytetrahydrofuran 35, we obtained better results when the dihydroxylation was quenched after five hours, followed by acid-catalyzed cyclization for complete conversion to the tetrahydrofuranyldiol 34, which was stable to isolation and characterization. In a separate step, basic methanolysis of 34 afforded the epoxide 35 from which the remaining tertiary alcohol was protected as silyl ether 36. Copper-catalyzed addition of 2-methallylmagnesium chloride,28 silylation of the resulting secondary alcohol 37, and ozonolysis afforded the methyl ketone 39,29 which was converted into enol triflate 40 via the kinetic enolate of 39.30
Stille coupling of the stannyl enol ether 31 and enol triflate 40 proceeded with minimal homodimerization of 31, provided that copper iodide and cesium fluoride additives were present (Scheme 7).31 As the fluoride salt also partially removed the silyl ethers, the dienyl ether product was best isolated as the diol 7 after complete desilylation with excess tetrabutylammonium fluoride.
At this stage, all that remained was the regioselective dihydroxylation of the C15-alkene of 7. We were optimistic of a favorable outcome due to our precedent of a similarly regioselective dihydroxylation of a dienyl ether in a model system directed towards the branched C-glycoside substructure of altromycin B (41 → 42, Scheme 8).32 Surprisingly, the attempted dihydroxylation of compound 7 with both AD-mix β and α gave no reaction, other than eventual decomposition of the dienyl ether after several days. The reaction of 7 with stoichiometric osmium tetraoxide33 afforded several very polar compounds, none of which matched the proton NMR spectra of thyrsenols A or B. The reaction of osmium tetraoxide with the acetate derivative 43 provided a mixture of diastereomers exhibiting mass spectra consistent with the tetraol arising from dihydroxylation of both alkenes of 43.34 It appears that the aryl ring of 41 must have played an activating role in the regioselective dihydroxylation of the 1,1-disubstituted alkene, compared to the corresponding C15 alkene of 7 or 43. Moreover the oxygen substituent at the allylic position of 41 may have deactivated the enol ether to dihydroxylation, whereas dihydroxylation occurred slowly but competitively with both alkenes of 43.
Although the tetracyclic skeleton of the thyrsenols was produced, unfortunately the natural product was not obtained, due to the absence of differential reactivity between the alkenes of dienyl ether 7 in the final dihydroxylation step. However, our work has demonstrated a variety of processes for the construction of cyclic ethers, including diastereoselective epoxyalcohol cyclization to form the A ring, ring expansion of a tetrahydrofuranyl alcohol to the tetrahydropyranyl alcohol isomer in the presence of an alkyne substituent, and the first example of tungsten-promoted alkynol cyclization in the presence of an alkyl bromide.
EXPERIMENTAL
1H and 13C NMR spectra were recorded on a VNMR-400 spectrometer (400 MHz for 1H, 100 MHz for 13C) or an INOVA-600 spectrometer (600 MHz for 1H, 150 MHz for 13C). NMR spectra were recorded on solutions in deuterated chloroform (CDCl3) with residual chloroform (δ 7.27 ppm for 1H NMR and δ 77.23 ppm for 13C NMR) taken as the internal standard, and were reported in parts per million (ppm). Abbreviations for signal coupling are as follows s, singlet; b, broad; d, doublet; t, triplet; q, quartet; m, multiplet. IR spectra were collected on a Mattson Genesis II FT-IR spectrometer as neat films. Mass spectra (high resolution FAB) were recorded on a VG 70-S Nier Johason Mass Spectrometer. Analytical thin layer chromatography (TLC) was performed on precoated glass backed plates purchased from Whatman (silica gel 60 F254; 0.25mm thickness). Flash chromatography was carried out with silica gel 60 (230-400 mesh ASTM) from EM Science. All reactions were carried out with anhydrous solvents in oven-dried or flame-dried and argon-charged glassware. All anhydrous solvents were dried over molecular sieves and water content assayed by Karl Fischer titration prior to use. The pH 7 buffer solution used in aqueous workups was prepared by diluting Na2HPO4 (18.9 g, 133 mmol) and KH2PO4 (9.1 g, 67 mmol) with deionized water to a volume of 1L. All reagents were purchased from Sigma-Aldrich or prepared as described in the cited literature.
Bromotetrahydropyranyl silyl ether (16): Chlorotriethylsilane (0.41 mL, 2.4 mmol) was added dropwise to a solution of bromotetrahydropyranyl alcohol 15 (0.619 g, 1.6 mmol)17 and DMAP (0.0649 g, 0.5 mmol) in pyridine (4 mL). The reaction mixture was stirred overnight, diluted with Et2O (50 mL) and washed with pH 7 buffer (50 mL). The aqueous layer was extracted with additional Et2O (3 x 50 mL). The combined organic layers were dried over MgSO4, concentrated and purified by silica gel column chromatography eluted with a gradient of 3% Et3N in hexanes to 9% Et3N in hexanes yielding the silyl ether 16 (0.688 g, 85%) as a clear oil. [α]23D +23.0 (CH2Cl2, c 1.03); IR (neat) 3583, 3434, 2955, 2876, 1740, 1657, 1456, 1381, 1232, 1115, 1019, 733 cm-1; 1H NMR (400 MHz, CDCl3) δ 0.61 (q, J = 7.6 Hz, 6H), 0.96 (t, J = 7.6 Hz, 9H), 1.17 (s, 3H), 1.26-1.36 (m, 1H), 1.28 (s, 3H), 1.42 (s, 3H), 1.49 (dt, J = 13.2, 4.4 Hz, 1H), 1.67 (t, J = 4.0 Hz, 1H), 1.70 (s, 3H), 1.81-1.89 (m, 1H), 1.99-2.30 (m, 4H), 2.07 (s, 3H), 3.25 (dd, J = 7.8, 3.0 Hz, 1H), 3.88 (dd, J = 12.6, 4.2 Hz, 1H), 4.60 (d, J = 7.2 Hz, 2H), 5.36 (dt, J = 7.2, 1.2 Hz, 1H); 13C NMR (100 MHz, CDCl3) δ 5.8, 7.3, 16.7, 19.4, 21.3, 23.8, 28.6, 30.7, 31.3, 37.1, 37.2, 59.2, 61.7, 75.3, 76.4, 81.0, 118.1, 143.1, 171.4; MS(FAB+) Calcd. for C23H44O479Br128Si1 [(M+H)+] 491.2187, found 491.2189.
Allylic alcohol (17): A solution of DIBAL (1 M in dichloromethane, 2.8 mL, 2.8 mmol) was added slowly to a -78 °C solution of allylic acetate 16 (0.688 g, 1.4 mmol) in dichloromethane (3 mL). The reaction mixture was stirred for 1 h, then allowed to warm to room temperature. After 3 h, sodium sulfate decahydrate was added gradually unti a gel formed. The gel was diluted with a saturated aqueous solution of sodium potassium tartrate (10 mL) and additional dichloromethane (10 mL). The aqueous layer was extracted with dichloromethane (2 x 10 mL) and the combined organic layers were dried over MgSO4. After concentration, the crude product was purified by silica gel column chromatography eluted with Et2O : hexanes (v/v 2 : 8) resulting in allylic alcohol 17 (0.602 g, 96%) as a clear oil. [α]23D +23.2 (CH2Cl2, c 1.74); IR (neat) 3413, 2955, 2876, 2359, 2092, 1640, 1456, 1372, 1237, 1115, 1011, 733 cm-1; 1H NMR (400 MHz, CDCl3) δ 0.61 (q, J = 8.0 Hz, 6H), 0.96 (t, J = 8.0 Hz, 9H), 1.17 (s, 3H) 1.26-1.40 (m, 2H), 1.28 (s, 3H), 1.42 (s, 3H), 1.50 (dt, J = 13.2, 4.4 Hz, 1H), 1.68 (s, 3H), 1.81-1.89 (m, 1H), 1.97-2.04 (m, 1H), 2.08-2.19 (m, 2H), 2.25 (dq, J = 13.2, 3.6 Hz, 1H), 3.25 (dd, J = 7.8, 3.0 Hz), 3.88 (dd, J = 12.6, 3.8 Hz, 1H), 4.17 (d, J = 6.8 Hz, 2H), 5.44 (dt, J = 6.8, 1.2 Hz, 1H); 13C NMR (100 MHz, CDCl3) δ 5.8, 7.3, 16.6, 19.4, 23.8, 28.6, 30.9, 31.3, 37.2, 37.3, 59.2, 59.7, 75.3, 76.4, 81.1, 123.2, 140.7; MS(FAB+) Calcd. for C21H41O379Br123Na128Si1 [(M+Na)+] 471.1901, found 471.1899.
Epoxy alcohol (18): 4Å powdered, activated molecular sieves (0.686 g) were suspended in a solution of allylic alcohol 17 (0.602 g, 1.3 mmol) in dichloromethane (11 mL) with stirring. The suspension was cooled to -20 °C and L-(+)-diethyl tartrate (0.07 mL, 0.41 mmol) followed by Ti(Oi-Pr)4 (0.09 mL, 0.30 mmol) were added. The precatalyst mixture was stirred for 30 min. An anhydrous solution of t-butyl hydroperoxide (5.5 M in decane, 0.7 mL, 3.9 mmol) was added slowly to the mixture and stirred at -20 °C for 1 h, then placed in the freezer (-25 °C) overnight. The reaction mixture was filtered though celite to remove the sieves and diluted with additional dichloromethane (50 mL) and an aqueous KOH solution (1.5 M, 50 mL). The biphasic mixture was stirred for 1 h. The aqueous layer was extracted with dichloromethane (2 x 50 mL). The combined organic layers were dried over MgSO4, concentrated and purified by silica gel column chromatography eluted with Et2O : hexanes (v/v 1 : 3) producing epoxy alcohol 18 (0.605 g, 97%) as a clear oil. [α]23D +19.1 (CH2Cl2, c 1.07); IR (neat) 3435, 2955, 2876, 1641, 1456, 1382, 1237, 1116, 1017, 732 cm-1; 1H NMR (400 MHz, CDCl3) δ 0.59 (q, J = 8.0 Hz, 6H), 0.95 (t, J = 8.0 Hz, 9H), 1.17 (s, 3H), 1.28 (s, 3H), 1.30 (s, 3H), 1.42 (s, 3H), 1.49 (dt, J = 13.4, 4.0 Hz, 1H), 1.56-1.72 (m, 4H), 1.75-1.87 (m, 1H), 2.11 (qd, J = 13.6, 4.0 Hz, 1H), 2.25 (dq, J = 13.2, 3.6 Hz, 1H), 2.97 (dd, J = 7.0, 4.0 Hz, 1H), 3.25 (dd, J = 7.4, 3.4 Hz, 1H), 3.67-3.73 (m, 1H), 3.82-3.89 (m, 2H); 13C NMR (100 MHz, CDCl3) δ 5.8, 7.3, 17.0, 19.4, 23.8, 28.0, 28.6, 31.3, 36.2, 37.2, 59.1, 61.7, 61.7, 62.8, 75.4, 76.4, 81.2; MS(FAB+) Calcd. for C21H42O479Br128Si1 [(M+H)+] 465.2030, found 465.2024.
Tetrahydrofuranyl diol (19): A solution of tetrabutylammonium fluoride (1 M in THF, 6 mL, 6 mmol) was added to a solution of epoxy alcohol 18 (0.605 g, 1.3 mmol) and glacial acetic acid (0.3 mL, 5.2 mmol) in THF (1 mL). The reaction was monitored by TLC. After starting material was fully consumed, the reaction was diluted with dichloromethane (10 mL) and washed with a saturated brine solution (3 x 10 mL). The organic layer was dried over MgSO4 and purified by silica gel column chromatography eluted with methanol : dichloromethane (v/v 2 : 98) producing diol 19 (0.410 g, 90%) as a clear oil. [α]23D +30.7 (CH2Cl2, c 1.47); IR (neat) 3400, 2975, 2939, 2875, 2359, 2340, 1646, 1455, 1380, 1130, 1089, 1111, 1035, 901, 731 cm-1; 1H NMR (400 MHz, CDCl3) δ 1.14 (s, 3H), 1.22 (s, 3H), 1.29 (s, 3H), 1.41 (s, 3H), 1.53-1.60 (m, 2H), 1.65 (dt, J = 13.2, 4.4 Hz, 1H), 1.76-1.89 (m, 2H), 1.96-2.04 (m, 1H), 2.11 (qd, J = 13.2, 4.0 Hz, 1H), 2.26 (dq, J = 13.0, 4.0 Hz, 1H), 3.53 (dd, J = 10.2, 7.4 Hz, 1H), 3.64-3.71 (m, 3H), 3.88 (dd, J = 12.4, 4.0 Hz, 1H); 13C NMR (100 MHz, CDCl3) δ 21.7, 23.3, 23.8, 26.6, 28.1, 31.1, 33.0, 34.7, 58.6, 63.5, 74.8, 75.3, 76.7, 65.0, 88.4; MS(FAB+) Calcd. for C15H28O479Br128 [(M+H)+] 351.1166, found 351.1166.
Primary acetate ester (20): Acetyl chloride (0.16 mL, 2.3 mmol) was added dropwise to a -78 °C solution of tetrahydrofuranyl diol 19 (0.648 g, 1.8 mmol) and 2,4,6-trimethylpyridine (0.49 mL, 3.7 mmol) in dichloromethane (3.7 mL). The reaction mixture was stirred for 2 h at -78 °C, then placed in a -25 °C freezer overnight. The reaction was quenched by the addition of an aqueous HCl solution (1 M, 2 mL, 2 mmol). The aqueous layer was extracted with Et2O (3 x 10 mL). The combined organic layers were dried over MgSO4, concentrated, and purified by silica gel column chromatography eluted with Et2O : hexanes (v/v 1 : 1) yielding primary acetate ester 20 (0.650 g, 90%) as a clear oil. 1H NMR (600 MHz, CDCl3) δ 1.18 (s, 3H), 1.25 (s, 3H), 1.30 (s, 3H), 1.43 (s, 3H), 1.58-1.61 (m, 3H), 1.88-1.91 (m, 2H), 2.04 (q, J = 12.0 Hz, 1.0 H), 2.11 (s, 3H), 2.12 (dq, J = 13.8, 4.2 Hz, 1H), 2.27 (dq, J = 13.0, 4.0 Hz, 1H), 3.66 (t, J = 7.8 Hz, 1H), 3.76 (dd, J = 7.8, 2.4 Hz, 1H), 3.87 (dd, J = 12.6, 4.2 Hz, 1H), 4.00 (dd, J = 11.7, 8.7 Hz, 1H), 4.27 (dd, J = 12.0, 2.4 Hz, 1H).
Bistetrahydropyran diol (24): Chloromethanesulfonyl chloride (0.16 mL, 1.8 mmol) was added dropwise to a 0 °C solution of primary acetate 20 (0.137 g, 0.35 mmol) and 2,6-lutidine (0.32 mL, 2.7 mmol) in dichloromethane (0.94 mL). The mixture was stirred for 30 min, then diluted with Et2O (10 mL) and an aqueous HCl solution (0.5 M, 5 mL). The aqueous layer was extracted with additional Et2O (2 x 5 mL). The combined organic layers were dried over MgSO4 and concentrated. The crude chloromesylate ester and zinc acetate dihydrate were dissolved in 1,4-dioxane (5 mL) and water (5 mL). The reaction mixture was heated to 72 °C and stirred for 3 h. The reaction mixture was diluted with aqueous HCl solution (0.5 M, 5 mL) and Et2O (10 mL). The aqueous layer was extracted with additional Et2O (2 x 10 mL). The combined organic layers were dried over MgSO4, concentrated and purified by silica gel column chromatography eluted with a gradient of EtOAc : hexanes (v/v 1: 3 to 5 : 7) yielding a mixture of diol 24 (0.0302 g, 25%) and the corresponding primary acetate 23 (0.0673 g, 49%). The primary acetate was removed by treatment with LiOH (0.012 g, 0.5 mmol) in a mixture of water (1 mL) and methanol (3 mL). After monitoring the reaction by TLC, the mixture was diluted with water (1 mL) and Et2O (5 mL). The aqueous layer was extracted with additional Et2O (2 x 5 mL). The combined organic layers were dried over MgSO4 and concentrated, and no additional purification was necessary. 1H NMR (400 MHz, CDCl3) δ 1.19 (s, 3H), 1.21 (s, 3H), 1.27 (s, 3H), 1.38-1.47 (m, 1H), 1.41 (s, 3H), 1.53-1.62 (m, 2H), 1.75-1.86 (m, 3H), 2.11 (qd, J = 13.6, 4.0 Hz, 1H), 2.26 (dq, J = 13.4, 4.0 Hz, 1H), 3.07 (dd, J = 17.2, 2.0 Hz, 1H), 3.31 (dd, J = 8.0, 6.6 Hz, 1H), 3.62 (dd, J = 17.2, 8.0 Hz, 1H), 3.78 (dd, J = 17.2, 6.6 Hz, 1H), 3.89 (dd, 12.4, 4.0 Hz, 1H).
Primary tosylate (25): p-Toluenesulfonyl chloride (0.455 g, 2.4 mmol) was added to a 0 °C solution of diol 19 (0.756 g, 2.2 mmol) in pyridine (31 mL). The reaction was stirred for 1 h, then placed in the refrigerator (4 °C) for 24 h. The reaction mixture was diluted with dichloromethane (50 mL) and washed with aqueous saturated brine (3 x 50 mL). The organic layer was dried over MgSO4, concentrated, and purified by silica gel column chromatography eluted with Et2O : hexanes (v/v 1 : 3) yielding primary tosylate 25 (0.815 g, 75%) as a clear oil. [α]23D +7.6 (CH2Cl2, c 5.57); IR (neat) 3522, 3442, 2980, 2876, 2359, 1599, 1454, 1362, 1176, 1128, 1094, 1034, 970, 909, 835, 815, 765, 732, 662, 554 cm-1; 1H NMR (400 MHz, CDCl3) δ 1.09 (s, 3H), 1.19 (s, 3H), 1.27 (s, 3H), 1.40 (s, 3H), 1.51-1.60 (m, 2H), 1.81-1.87 (m, 2H), 1.92-2.00 (m, 1H), 2.09 (qd, J = 13.6, 4.0 Hz, 1H), 2.19-2.30 (m, 1H), 2.45 (s, 3H), 2.60 (d, J = 3.2 Hz, 1H), 3.58 (t, J = 7.4 Hz, 1H), 3.72 (dt, J = 8.0, 2.8 Hz, 1H), 3.86 (dd, J = 12.4, 4.0 Hz, 1H), 3.98 (dd, J = 10.6, 8.0 Hz, 1H), 4.24 (dd, J = 10.6, 2.8 Hz, 1H), 7.35 (d, J = 8.0 Hz, 2H), 7.81 (d, J = 8.4 Hz, 2H); 13C NMR (100 MHz, CDCl3) δ 21.4, 21.9, 22.7, 23.8, 26.3, 28.1, 31.1, 33.8, 35.4, 58.7, 71.9, 74.7, 74.7, 75.1, 83.8, 88.5, 128.2, 130.1, 133.0, 145.1; MS(FAB+) Calcd. for C22H34O679Br132S1 [(M+H)+] 505.1254, found 505.1254.
Terminal epoxide (26): Potassium carbonate (0.670 g, 4.8 mmol) was added to a solution of primary tosylate 25 (0.815 g, 1.6 mmol) in methanol (16 mL) and stirred for 1 h. The reaction mixture was concentrated, diluted with Et2O (50 mL) and washed with pH 7 buffer (3 x 50 mL). The organic layer was dried over MgSO4, concentrated, and purified by silica gel column chromatography eluted with Et2O : hexanes (v/v 1 : 3) producing terminal epoxide 26 (0.491 g, 91%) as a clear oil. [α]23D +23.2 (CH2Cl2, c 0.94); IR (neat) 3583, 2980, 2873, 2359, 1462, 1452, 1381, 1370, 1242, 1130, 1036 cm-1; 1H NMR (400 MHz, CDCl3) δ 1.23 (s, 3H), 1.24 (s, 3H), 1.29 (s, 3H), 1.42 (s, 3H), 1.54-1.67 (m, 2H), 1.87-1.92 (m, 2H), 2.12 (qd, J = 13.6, 4.0 Hz, 1H), 2.26 (dq, J = 13.0, 4.0 Hz, 1H), 2.58 (dd, J = 5.2, 2.8 Hz, 1H), 2.72 (t, J = 4.6 Hz, 1H), 2.97 (dd, J = 4.4, 2.8 Hz, 1H), 3.66 (t, J = 7.0 Hz, 1H), 3.89 (dd, J = 12.4, 4.0 Hz, 1H); 13C NMR (100 MHz, CDCl3) δ 20.9, 23.9, 24.0 26.5, 28.2, 31.1, 33.1, 36.1, 44.4, 57.4, 59.0, 75.1, 81.6, 88.3; MS(FAB+) Calcd. for C15H26O379Br128 [(M+H)+] 333.1060, found 333.1060.
Silyl alkynyl alcohol (27): A solution of n-butyllithium (1.6 M in hexanes, 3.5 mL, 5.6 mmol) was added to a -78 °C solution of trimethylsilyl acetylene (0.69 mL, 4.9 mmol) in THF (10 mL). The alkynyllithium solution was allowed to warm to -55 °C and stirred for 1 h. The mixture was cooled to -78 °C, boron trifluoride tetrahydrofuran complex (0.69 mL, 6.3 mmol) was added and the acetylide mixture stirred for 20 min. A solution of terminal epoxide 26 (0.491 g, 1.5 mmol) in THF (8 mL) was added via cannula to the acetylide solution. The reaction mixture was stirred for -78 °C for 2 h, then allowed to warm slowly to room temperature over the period of 2 h. The reaction mixture was diluted with Et2O (75 mL) and washed with pH 7 buffer (3 x 30 mL). The organic layer was dried over MgSO4, concentrated, and purified by silica gel column chromatography eluted with Et2O : hexanes (v/v 1 : 10) yielding alkynyl alcohol 27 (0.634 g, 99%) as a clear oil. [α]23D +31.0 (CH2Cl2, c 3.19); IR (neat) 3436, 2973, 2898, 2877, 2175, 1642, 1456, 1381, 1250, 1132, 1076, 1034, 844, 760 cm-1; 1H NMR (400 MHz, CDCl3) δ 0.15 (s, 9H), 1.15 (s, 3H), 1.22 (s, 3H), 1.29 (s, 3H), 1.41 (s, 3H), 1.56-1.63 (m, 2H), 1.83-1.89 (m, 2H), 1.98-2.05 (m, 1H), 2.11 (qd, J = 13.6, 4.0 Hz, 1H), 2.20-2.30 (m, 1H), 2.35 (dd, J = 16.8, 9.0 Hz, 1H), 2.50 (dd, J = 16.8, 3.6 Hz, 1H), 3.61-3.67 (m, 2H), 3.88 (dd, J = 12.8, 4.0 Hz, 1H); 13C NMR (100 MHz, CDCl3) δ 0.26, 21.2, 22.9, 23.8, 24.0, 26.6, 28.2, 31.1, 33.0, 35.7, 58.9, 74.8, 75.1, 75.2, 85.2, 86.9, 88.6, 104.5; MS(FAB+) Calcd. for C20H35O379Br123Na128Si1 [(M+Na)+] 453.1431, found 453.1432.
Alkynyl alcohol (29): Chloromethanesulfonyl chloride (0.21 mL, 2.4 mmol) was added dropwise to a solution of alkynyl alcohol 27 (0.207 g, 0.48 mmol) and 2,6-lutidine (0.45 mL, 3.9 mmol) in dichloromethane (1.3 mL) at 0 °C. The reaction mixture was stirred for 1 h, diluted with ethyl acetate (10 mL) and washed with saturated aqueous brine (2 x 5 mL). The organic layer was dried over MgSO4 and concentrated. The crude chloromesylate 28 was dissolved in dioxane (6.5 mL) and water (6.5 mL). Zinc acetate dihydrate (0.422 g, 1.9 mmol) was added to the solution and the reaction mixture was heated to 60 °C for 5 h. The reaction mixture was diluted with dichloromethane (10 mL) and pH 7 buffer (5 mL). The aqueous layer was extracted with additional dichloromethane (2 x 10 mL), the combined organic layers were dried over MgSO4, concentrated, and purified by silica gel column chromatography eluted with Et2O : hexanes (v/v 1 : 10) yielding bistetrahydropyranyl alcohol 29 (0.0638 g, 31%) as a clear oil. [α]23D +40.8 (CH2Cl2, c 0.25); IR (neat) 3413, 2959, 2859, 2361, 2178, 1718, 1631, 1470, 1380, 1249, 1129, 1103, 1072, 1017, 843, 760 cm-1; 1H NMR (400 MHz, CDCl3) δ 0.14 (s, 9H), 1.19 (s, 3H), 1.20 (s, 3H), 1.27 (s, 3H), 1.40 (s, 3H), 1.50-1.64 (m, 2H), 1.75-1.87 (m, 4H), 2.10 (qd, J = 13.6, 4.0 Hz, 1H), 2.25 (dq, J = 13.4, 4.0 Hz, 1H), 2.34 (dd, J = 17.2, 8.0 Hz, 1H), 3.04 (dd, J = 12.4, 3.6 Hz, 1H), 3.38 (dd, J = 8.0, 6.8 Hz, 1H), 3.91 (dd, J = 12.0, 4.4 Hz, 1H); 13C NMR (100 MHz, CDCl3) δ 0.2, 20.2, 20.3, 21.4, 23.2, 23.9, 28.4, 29.9, 31.2, 37.2, 39.2, 59.2, 70.6, 74.6, 75.2, 82.3, 86.5, 86.9, 104.8; MS(FAB+) Calcd. for C20H36O379Br128Si1 [(M+H)+] 431.1612, found 431.1600.
Bistetrahydropyranyl alcohol (30): Potassium carbonate (0.0984 g, 0.71 mmol) was added to a solution of silyl alkynyl alcohol 29 (0.0638 g, 0.15 mmol) in methanol (3 mL) and stirred overnight. The reaction mixture was concentrated, diluted with dichloromethane (10 mL) and washed with pH 7 buffer (3 x 5 mL). The organic layer was dried over MgSO4, concentrated, purified by silica gel column chromatography eluted with methanol : dichloromethane (v/v 1 : 199) resulting in alkynyl alcohol 30 (0.0319 g, 60%) as a white solid. mp 61-62 °C; [α]23D +46.4 (CH2Cl2, c 0.83); IR (neat) 3413, 3307, 2924, 2856, 1462, 1381, 1265, 1128, 1103, 1072, 1028, 980, 737 cm-1; 1H NMR (400 MHz, CDCl3) δ 1.17 (s, 3H), 1.19 (s, 3H), 1.27 (s, 3H), 1.40 (s, 3H), 1.56 (tt, J = 13.2, 4.0 Hz, 2H), 1.74-1.87 (m, 4H), 2.00 (t, J = 2.6 Hz, 1H), 2.11 (qd, J = 13.6, 4.0 Hz, 1H), 2.19-2.30 (m, 2H), 2.49 (ddd, J = 16.8, 5.2, 2.8 Hz, 1H), 3.05 (dd, J = 11.6, 2.0 Hz, 1H), 3.56 (dd, J = 8.2, 5 Hz, 1H), 3.90 (dd, J = 12.2, 4.0 Hz, 1H); 13C NMR (100 MHz, CDCl3) δ 19.9, 20.2, 20.2, 23.3, 23.8, 28.4, 29.9, 31.2, 37.0, 39.5, 59.2, 69.7, 70.4, 74.6, 75.1, 82.4, 82.6, 86.4; MS(FAB+) Calcd. for C17H28O379Br1 [(M+H)+] 359.1216, found 359.1218.
α-Stannyldihydropyran (31): A solution of alkynyl alcohol 30 (0.0319 g, 0.09 mmol) and tungsten hexacarbonyl (0.163 g, 0.5 mmol) in anhydrous degassed THF (4 mL) was irradiated at 350 nm for 2 h. The reaction mixture was stirred for 16 h, then irradiated for an additional 2 h. After stirring for an additional 24 h, the reaction mixture was concentrated. Powdered, activated 4 Å molecular sieves (0.6 g) and Et2O were added to the crude tungsten oxacarbene and stirred for 10 min. A solution of tributyltin triflate35 (0.103 g, 0.24 mmol) in Et2O (0.5 mL) was added and stirred for 10 min, followed by the addition of Et3N (0.86 mL, 6.2 mmol). After stirring overnight the mixture was filtered to remove the sieves and concentrated. The crude yellow solid was purified by silica gel column chromatography eluted with Et2NH : hexanes (v/v 2 : 98), producing stannyl dihydropyran 31 as an off white solid (0.0229 g, 40%). [α]23D -2.5 (CH2Cl2, c 0.49); IR (neat) 2954, 2926, 2852, 1600, 1455, 1375, 1132, 1101, 1044, 1022, 952, 903. 736 cm-1; 1H NMR (400 MHz, CDCl3) δ 0.87-0.92 (m, satellite signals from coupling to 117/119Sn were observed, JSn-H = 26.0 Hz, 15H), 1.10, (s, 3H), 1.21 (s, 3H), 1.26-1.36 (m, 6H), 1.28 (s, 3H), 1.41 (s, 3H), 1.47-1.58 (m, 9H), 1.76-1.92 (m, 4H), 2.00-2.14 (m, 2H), 2.25 (dq, J = 13.6, 4.0 Hz, 1H), 3.08 (dd, J = 10.6, 2.6 Hz, 1H), 3.33 (dd, J =11.0, 5.6 Hz, 1H), 3.91 (dd, J = 12.0, 4.0 Hz, 1H), 4.61 (dd, J = 5.6, 2.0 Hz, satellite signals from coupling to 117/119Sn were observed, 3JSn-H = 14.0 Hz, 1H); 13C NMR (100 MHz, CDCl3) δ 9.8, 14.0, 15.8, 20.2, 23.2, 23.9, 26.0, 27.4, 28.4, 29.1, 29.2, 31.2, 36.9, 37.4, 59.3, 73.9, 74.5, 75.1, 86.4, 108.7, 160.0; MS(FAB+) Calcd. for C29H54O379Br1120Sn1 [(M+H)+] 649.2273, found 649.2258.
2,3-Epoxygeraniol (32):19 4Å powdered, activated molecular sieves (5.18 g) were suspended in anhydrous dichloromethane (50 mL) in a 100 mL 3-necked round bottom flask equipped with a thermometer. The suspension was cooled to -20 °C and L-(+)-diethyl tartrate (0.22 mL, 1.28 mmol) and Ti(Oi-Pr)4 (0.25 mL, 0.84 mmol) were added with stirring. A solution of anhydrous TBHP (5.5M in decane, 4.8 mL, 26.4 mmol) was added dropwise and the catalyst mixture was aged for 30 min at -20 °C. Geraniol (11, 3 mL, 17.3 mmol) was added over a period of 20 min. The reaction mixture was stirred for 5 h at -20 °C then placed in a -25 °C freezer overnight. The cold mixture was filtered through celite and stirred with a solution of KOH (1.5 M, 50 mL) for 1 h. The biphasic mixture was separated and the aqueous layer was extracted with additional dichloromethane (3 x 50 mL). The combined organic layers were dried over MgSO4 and concentrated. The resultant oil was purified by silica gel column chromatography eluted with diethyl ether : hexanes (v/v 1 : 3) yielding 2,3-epoxygeraniol 32 (2.88 g, 98%) as a colorless oil. [α]23D -5.8 (CH2Cl2, c 0.99) {lit.,19 [α]25D -5.3 (CHCl3, c 3.0)}; IR (neat) 3430, 2968, 2925, 2859, 1673, 1453, 1384, 1251, 1222, 1076, 1037, 865 cm-1; 1H NMR (400 MHz, CDCl3) δ 1.31 (s, 3H), 1.44-1.52 (m, 1H), 1.62 (s, 3H), 1.65-1.73 (m, 1H), 1.69 (s, 3H), 2.09 (bq, J = 7.6 Hz, 2H), 2.99 (dd, J = 6.4, 4.4 Hz, 1H), 3.67-3.72 (m, 1H), 3.81-3.87 (m, 1H), 5.09 (tt, J = 7.0, 1.6 Hz, 1H); 13C NMR (100 MHz, CDCl3) δ 17.0, 17.9, 23.9, 25.9, 38.7, 61.4, 61.7, 63.1, 123.5, 132.4; MS(FAB+) Calcd. for C10H19O2 [(M+H)+] 171.1380, found 171.1375.
1-Tosyl-2,3-epoxygeraniol (33):26 2,3-Epoxygeraniol 32 (2.88 g, 16.9 mmol) was dissolved in pyridine (16 mL) and cooled to 0 °C. p-Toluenesulfonyl chloride (3.56 g, 18.7 mmol) was added and stirred until just dissolved. The reaction mixture was then placed in the freezer (-25 °C) overnight. The cold mixture was filtered to remove the solids and the solids were rinsed with cold Et2O. The filtrate was washed with pH 7 buffer (3 x 50 mL) and the organic layers were dried over MgSO4 and concentrated. The resultant oil was purified by silica gel column chromatography eluted with Et2O : hexanes (v/v 1 : 10) yielding tosylepoxygeraniol 33 (4.42 g, 81%) as a clear oil. [α]23D -4.6 (CH2Cl2, c 0.99); IR (neat) 2968, 2925, 2859, 1598, 1452, 1364, 1177, 1097, 966, 816 cm-1; 1H NMR (400 MHz, CDCl3) δ 1.21 (s, 3H), 1.40-1.47 (m, 1H), 1.60 (s, 3H), 1.60-1.68 (m, 1H), 1.68 (s, 3H), 2.04 (bq, J = 8.0 Hz, 2H), 2.46 (s, 3H), 2.98 (t, J = 5.6 Hz, 1H), 4.12 (dq, J = 11.2, 5.6 Hz, 2H), 5.04 (tt, J = 7.2, 1.4 Hz, 1H), 7.37 (d, J = 8.0 Hz, 2H), 7.82 (d, J = 8.4 Hz, 2H); 13C NMR (100 MHz, CDCl3) δ 16.9, 17.9, 21.9, 23.7, 25.9, 38.2, 58.9, 61.1, 68.8, 123.2, 128.2, 130.2, 132.6, 132.8, 145.3; MS(FAB+) Calcd. for C17H24O423Na132S1 [(M+Na)+] 347.1288, found 347.1284.
Tosyltetrahydrofuran (34): Tosylepoxygeraniol 33 (2.20 g, 6.77 mmol) was dissolved in a mixture of t-BuOH : H2O (v/v 1 : 1, 70 mL). AD mix β (9.48 g) and methanesulfonamide (0.647 g, 6.8 mmol) were added to the solution. After the solids had dissolved completely, the biphasic mixture was cooled to 0 °C and stirred for 5 h. The reaction was allowed to warm to room temperature and stirred overnight. Sodium sulfite (10.2 g, 81 mmol) was added and stirred for 1 h. The layers were separated and the aqueous layer was extracted with dichloromethane (3 x 150 mL). The combined organic layers were dried over MgSO4 and concentrated. The yellow oil was diluted with dichloromethane (100 mL); camphorsulfonic acid (0.149 g, 0.6 mmol) was added and stirred for 10 min. The acid solution was concentrated producing a yellow oil which was purified by silica gel column chromatography eluted with MeOH : dichloromethane (v/v 2 : 98). The tosylated tetrahydrofuran 34 was obtained as a clear oil (2.26 g, 93%). [α]23D -8.8 (CH2Cl2, c 0.79); IR (neat) 3535, 3409, 2974, 2876, 1598, 1453, 1358, 1175, 1094, 968, 837, 816 cm-1; 1H NMR (400 MHz, CDCl3) δ 1.11 (s, 3H), 1.13 (s, 3H), 1.18 (s, 3H), 1.64-1.70 (m, 1H), 1.82-1.88 (m, 2H), 2.02-2.09 (m, 1H), 2.46 (s, 3H), 3.72 (dd, J = 9.0, 6.6 Hz, 1H), 3.78 (dd, J = 8.0, 2.8 Hz, 1H), 4.01 (dd, J = 10.4, 7.6 Hz, 1H), 4.25 (dd, J = 10.4, 2.2 Hz, 1H), 7.37 (d, J = 8.0 Hz, 2H), 7.82 (d, J = 8.8 Hz, 2H); 13C NMR (100 MHz, CDCl3) δ 21.9, 22.9, 24.2, 26.4, 27.7, 34.3, 70.7, 71.7, 74.9, 83.8, 87.4, 128.2, 130.2, 132.9, 145.3; MS(FAB+) Calcd. for C17H27O632S1 [(M+H)+] 359.1523, found 359.1513.
Epoxytetrahydrofuranyl alcohol (35):26 Tosyltetrahydrofuran 34 (2.26 g, 6.3 mmol) was dissolved in MeOH (30 mL) with stirring. Potassium carbonate (3.49 g, 25.2 mmol) was added and the suspension was stirred for 15 min. The reaction mixture was concentrated to 5 mL, the residue was diluted with dichloromethane (50 mL) followed by an aqueous solution of NH4Cl (1 M, 25 mL, 25 mmol). The aqueous layer was diluted with pH 7 buffer (50 mL) and extracted with dichloromethane (3 x 50 mL). The combined organic layers were dried over MgSO4 and concentrated. The crude product was purified by silica gel column chromatography eluted with Et3N : EtOAc : hexanes (3 : 22 : 75) yielding epoxytetrahydrofuranyl alcohol 35 (1.03 g, 87%). [α]23D -2.7 (CH2Cl2, c 1.39) {lit.26 [α]D -2.45 (CHCl3, c 6.72)}; IR (neat) 3461, 3057, 2975, 2934, 2874, 1725, 1642, 1464, 1373, 1308, 1241, 1178, 1142, 1055, 986, 943, 915, 898, 863, 814 cm-1; 1H NMR (400 MHz, CDCl3) δ 1.13 (s, 3H), 1.23 (s, 3H), 1.28 (s, 3H), 1.61-1.64 (m, 2H), 1.82-1.87 (m, 2H), 2.59 (dd, J = 5.2, 2.8 Hz, 1H), 2.75, (t, J = 4.4 Hz, 1H), 3.04 (dd, J = 4.0, 2.8 Hz, 1H), 3.78 (t, J = 7.6 Hz, 1H); 13C NMR (100 MHz, CDCl3) δ 24.3, 24.5, 26.4, 27.7, 32.9, 44.1, 57.3, 70.8, 81.5, 87.0; MS(FAB+) Calcd. for C10H19O3 [(M+H)+] 187.1329, found 187.1327.
Epoxytetrahydrofuranyl silyl ether (36): Trimethylsilyl chloride was added dropwise to a solution of epoxytetrahydrofuranyl alcohol 35 (1.02 g, 5.5 mmol) and imidazole (1.51 g, 22.1 mmol) in THF (5 mL). The solution was stirred overnight. The reaction mixture was diluted with Et2O (50 mL) and pH 7 buffer (50 mL). The organic layer was washed with additional pH 7 buffer (2 x 50 mL), then dried over MgSO4. After concentration of the crude product, purification by silica gel column chromatography eluted with Et3N : hexanes (v/v 3 : 97) yielded the silyl ether 36 (1.03 g, 72%). [α]23D -2.9 (CH2Cl2, c 1.41); IR (neat) 3436, 3052, 2974, 2897, 2874, 1632, 1462, 1382, 1369, 1250, 1174, 1101, 1063, 1044, 916, 840 cm-1; 1H NMR (400 MHz, CDCl3) δ 0.12 (s, 9H), 1.18 (s, 3H), 1.22 (s, 3H), 1.24 (s, 3H), 1.57-1.63 (m, 1H), 1.75-1.81 (m, 1H), 1.86-1.93 (m, 2H), 2.59 (dd, J = 5.2, 2.8 Hz, 1H), 2.73 (bt, J = 4.6 Hz, 1H), 3.00 (dd, J = 4.2, 3.0 Hz, 1H), 3.72 (t, J = 7.0 Hz, 1H); 13C NMR (100 MHz, CDCl3) δ 2.8, 24.0, 25.9, 26.7, 27.8, 33.2, 44.4, 57.4, 75.0, 81.7, 87.7; MS(FAB+) Calcd. for C13H27O328Si1 [(M+H)+] 259.1724, found 259.1721.
Alkenyl tetrahydrofuranyl alcohol (37): A solution of 2-methylallylmagnesium chloride (0.5 M in THF, 13 mL, 6.5 mmol) was added dropwise to a -40 °C suspension of CuI (0.184 g, 0.97 mmol) in THF (3 mL) and stirred for 10 min. A solution of epoxytetrahydrofuran 36 (1.03 g, 5.0 mmol) in THF (4 mL) was added to the Grignard solution. The reaction was warmed to 0 °C and stirred for 30 min, then allowed to warm to room temperature. The reaction was stirred for an additional 30 min, then diluted with Et2O (50 mL) and pH 7 buffer (50 mL). The aqueous layer was extracted with additional Et2O (2 x 50 mL). The combined organic layers were dried over MgSO4 and concentrated. The crude product was purified by neutral alumina column chromatography eluted with Et2O : toluene (v/v 1 : 199) yielding unsaturated alcohol 47 (0.992 g, 79%) as a clear oil. [α]23D +6.8 (CH2Cl2, c 0.27); IR (neat) 3462, 2970, 2872, 2095, 1648, 1454, 1380, 1250, 1176, 1131, 1040, 909, 883, 840 cm-1; 1H NMR (400 MHz, CDCl3) δ 0.12 (s, 9H), 1.13 (s, 3H), 1.17 (s, 3H), 1.24 (s, 3H), 1.36-1.45 (m, 1H), 1.51 (ddd, J = 11.4, 7.4, 2.0 Hz, 1H), 1.55-1.63 (m, 1H), 1.75 (s, 3H), 1.80-1.96 (m, 2H), 2.01-2.15 (m, 2H), 2.29-2.36 (m, 1H), 2.44 (t, J = 2.0 Hz, 1H), 3.51 (dt, J = 10.8, 2.0 Hz, 1H), 3.69 (dd, J = 9.8, 5.8 Hz, 1H), 4.72-4.73 (m, 2H); 13C NMR (100 MHz, CDCl3) δ 2.8, 22.8, 24.1, 26.5, 26.9, 27.3, 29.8, 31.1, 35.1, 74.5, 76.4, 86.3, 88.6, 110.1, 146.2; MS(FAB+) Calcd. for C17H38O3N128Si1 [(M+NH4)+] 332.2616, found 332.2614.
Unsaturated bisilyl ether tetrahydrofuran (38): Trimethylsilyl chloride (1.3 mL, 10.3 mmol) was added dropwise to a solution of unsaturated alcohol 37 (0.992 g, 3.2 mmol) and imidazole (1.42 g, 20.9 mmol) in THF (20 mL). The reaction mixture was stirred overnight then concentrated. The resulting yellow oil was diluted with dichloromethane (50 mL) and pH 7 buffer (50 mL). The organic layer was washed with additional pH 7 buffer (2 x 50 mL) then dried over MgSO4. The crude product was purified by silica gel column chromatography eluted with Et3N : hexanes (v/v 3 : 97) yielding silyl ether 38 (1.12 g, 92%). [α]23D +0.5 (CH2Cl2, c 1.26); IR (neat) 3076, 2960, 2873, 1650, 1454, 1373, 1250, 1173, 1131, 1102, 1066, 1042, 840 cm-1; 1H NMR (400 MHz, CDCl3) δ 0.11 (s, 9H), 0.13 (s, 9H), 1.09 (s, 3H), 1.17 (s, 3H), 1.21 (s, 3H), 1.35-1.44 (m, 1H), 1.50-1.55 (m, 1H), 1.66-1.73 (m, 1H), 1.74 (s, 3H), 1.76-2.00 (m, 4H), 2.14-2.21 (m, 1H), 3.52 (dd, J = 9.8, 2.6 Hz, 1H), 3.66 (dd, J = 9.0, 5.8 Hz, 1H), 4.71 (bd, J = 5.6 Hz, 2H); 13C NMR (100 MHz, CDCl3) δ 1.2, 2.8, 22.9, 23.5, 26.2, 26.7, 27.6, 31.5, 33.6, 35.2, 74.8, 78.2, 85.9, 87.3, 109.8, 146.5; MS(FAB+) Calcd. for C20H46O3N128Si2 [(M+NH4)+] 404.3011, found 404.3011.
Methyl ketone (39): Ozone was bubbled through a -78 °C solution of alkene 38 (1.78 g, 4.6 mmol), pyridine (9 mL), methanol (15 mL), and isopropanol (15 mL) with stirring. The reaction was monitored by TLC. After complete consumption of alkene, dimethyl sulfide (1.4 mL, 19.1 mmol) was added and the solution was warmed to room temperature. The reaction mixture was stirred overnight, then concentrated. The crude product mixture was diluted with Et2O (50 mL), washed with pH 7 buffer (3 x 50 mL), then dried over MgSO4 and concentrated. Trimethylsilyl chloride (1.4 mL, 11.1 mmol) was added dropwise to a solution of the resultant oil and imidazole (1.80 g, 26.4 mmol) in THF (7 mL). The reaction mixture was stirred overnight then concentrated. The yellow oil was dissolved in Et2O (50 mL), washed with pH 7 buffer (3 x 50 mL), dried over MgSO4, and concentrated. The crude product was purified by silica gel column chromatography eluted with Et3N : hexanes (v/v 3 : 97) resulting in methyl ketone 39 (1.20 g, 67%). [α]23D +1.1 (CH2Cl2, c 1.69); IR (neat) 2962, 2899, 2873, 1720, 1454, 1410, 1362, 1250, 1174, 1103, 1041, 912, 841 cm-1; 1H NMR (400 MHz, CDCl3) δ 0.10 (s, 9H), 0.12 (s, 9H), 1.09 (s, 3H), 1.15 (s, 3H), 1.20 (s, 3H), 1.47-1.56 (m, 2H), 1.75-1.97 (m, 4H), 2.15 (s, 3H), 2.39-2.47 (m, 1H), 2.57-2.65 (m, 1H), 3.52 (dd, J = 9.4, 3.4 Hz, 1H), 3.63 (dd, J = 9.0, 5.8 Hz, 1H); 13C NMR (100 MHz, CDCl3) δ 1.0, 2.8, 23.5, 26.2, 26.5, 27.5, 27.6, 30.1, 33.4, 41.2, 74.7, 85.9, 87.5, 209.4; MS(FAB+) Calcd. for C19H41O428Si2 [(M+H)+] 389.2538, found 389.2539.
Enol triflate (40): A solution of KHMDS (0.5 M in toluene, 1.5 mL, 0.75 mmol) was added dropwise to a -78 °C solution of methyl ketone 39 (0.261 g, 0.67 mmol) in THF (7 mL). The enolate solution was stirred for 1 h. A solution of N-phenyl-bis(trifluoromethanesulfonimide) (0.362 g, 1.0 mmol) in THF (2 mL) was added dropwise to the enolate solution. The reaction was stirred at -78 °C for 10 min, then allowed to warm to room temperature. After 1 h the reaction mixture was concentrated, diluted with Et2O (10 mL), washed with pH 7 buffer (2 x 5 mL), and dried over MgSO4. After concentration the crude product was purified by silica gel column chromatography eluted with Et3N : hexanes (v/v 3 : 97) yielding enol triflate 40 (0.304 g, 87%). [α]23D +1.1 (CH2Cl2, c 0.51); IR (neat) 2962, 2900, 2873, 1670, 1419, 1252, 1213, 1176, 1146, 1101, 1066, 1041, 939, 883, 841 cm-1; 1H NMR (400 MHz, CDCl3) δ 0.11 (s, 9H), 0.13 (s, 9H), 1.09 (s, 3H), 1.16 (s, 3H), 1.21 (s, 3H), 1.47-1.61 (m, 2H), 1.75-1.95 (m, 4H), 2.27-2.35 (m, 1H), 2.48-2.56 (m, 1H), 3.52 (dd, J = 9.4, 3.4 Hz, 1H), 3.64 (dd, J = 9.0, 5.8 Hz, 1H), 4.97 (d, J = 3.6 Hz, 1H), 5.11 (d, J = 3.6 Hz, 1H); 13C NMR (100 MHz, CDCl3) δ 1.0, 2.8, 23.0, 26.2, 26.5, 27.5, 29.9, 30.0, 31.4, 34.2, 74.6, 85.6, 87.5, 104.3, 113.9, 117.1, 120.3, 123.5, 157.4; MS(FAB+) Calcd. for C20H39O6F323Na132S128Si2 [(M+Na)+] 543.1850, found 543.1860.
Dienyl ether (7): A solution of enol triflate 40 (39.6 mg, 76 μmol) in DMF (6 mL) was degassed by three freeze-pump-thaw cycles, and then was transferred to a flask containing α-stannyl dihydropyran 31 (42.1 mg, 65 μmol), tetrakis(triphenylphosphine)palladium(0) (1.8 mg, 1.6 μmol), CuI36 (10.4 mg, 55 μmol), and CsF (0.02 g, 0.13 mmol). The reaction mixture was degassed an additional 2 cycles. The mixture was heated to 50 °C and stirred for 22 h. After cooling, the solution was diluted with EtOAc (20 mL), dichloromethane (20 mL) and water (10 mL) and stirred for an additional 1 h. The biphasic mixture was filtered through celite and diluted with saturated brine (20 mL). The aqueous layer was extracted with a EtOAc : dichloromethane (1 : 1) solution (20 mL x 3). The combined organic layers were dried over MgSO4, concentrated, and purified by silica gel column chromatography eluted with a gradient of Et2O : hexanes (v/v 1 : 4 to 1 : 1) resulting in a mixture of monosilyl product and free diol. The mixture was diluted with THF (1 mL) and a solution of tetrabutylammonium fluoride (1 M in THF, 1 mL, 1 mmol) and stirred for 1 h. The crude diol solution was concentrated and purified by silica gel column chromatography eluted with methanol : dichloromethane (v/v 2 : 98) yielding the dienyl ether 7 (23.3 mg, 61%) as a waxy solid. mp 119-122 °C; [α]23D -7 (CH2Cl2, c 0.145); IR (neat) 3435, 2972, 2926, 2852, 1641, 1458, 1377, 1321, 1180, 1122, 1105, 1022, 895 cm-1; 1H NMR (600 MHz, CDCl3) δ 1.11 (s, 3H), 1.13 (s, 6H), 1.22 (s, 6H), 1.28 (s, 3H), 1.41 (s, 3H), 1.50-1.58 (m, 3H), 1.62-1.70 (m, 2H), 1.82-1.89 (m, 3H), 1.93-1.98 (m, 3H), 2.07-2.14 (m, 2H), 2.17-2.28 (m, 3H), 2.32 (bt, J = 1.8 Hz, 1H), 2.50-2.55 (m, 1H), 3.10 (dd, J = 11.4, 1.8 Hz, 1H), 3.36 (dd, J = 10.8, 6.0 Hz, 1H), 3.54 (bd, J = 10.2 Hz, 1H), 3.76 (dd, J = 10.5, 5.7 Hz, 1H), 3.91 (dd, J = 12.6, 4.2 Hz, 1H), 4.92-4.93 (m, 2H), 5.41 (bd, J = 1.2 Hz, 1H); 13C NMR (100 MHz, CDCl3) δ 15.4, 20.2, 23.0, 23.9, 24.1, 24.1, 25.2, 26.8, 28.0, 28.4, 30.1, 31.1, 31.2, 31.4, 36.7, 37.4, 59.2, 70.6, 73.5, 74.6, 75.2, 76.5, 76.7, 86.3, 86.5, 87.9, 96.0, 111.4, 142.8, 149.1; MS(FAB+) Calcd. for C30H50O679Br1 [(M+H)+] 585.2785, found 585.2783.
ACKNOWLEDGEMENTS
This work was supported primarily by the National Science Foundation (CHE-0516793).
References
1. Based on the M.S. thesis of Mary A. Smart, Emory University, 2008.
2. J. J. Fernández, M. L. Souto, and M. Norte, Nat. Prod. Rep., 2000, 17, 235. CrossRef
3. J. W. Blunt, M. P. Hartshorn, J. T. McLennan, and M. H. G. Munro, Tetrahedron Lett., 1978, 19, 69; CrossRef T. Suzuki, M. Suzuki, A. Furusaki, T. Matsumoto, A. Kato, Y. Imanaka, and E. Kurosawa, Tetrahedron Lett., 1985, 26, 1329. CrossRef
4. S. Sakemi, T. Higa, C. W. Jefford, and G. Bernardinelli, Tetrahedron Lett., 1986, 27, 4287. CrossRef
5. C. P. Manríquez, M. L. Souto, J. A. Gavín, M. Norte, and J. J. Fernández, Tetrahedron, 2001, 57, 3117. CrossRef
6. M. Norte, J. J. Fernández, M. L. Souto, J. Gavín, and M. D. Gracía-Grávalos, Tetrahedron, 1997, 53, 3173. CrossRef
7. M. A. Boone, R. Tong, F. E. McDonald, S. Lense, R. Cao, and K. I. Hardcastle, J. Am. Chem. Soc., 2010, 132, 5300. CrossRef
8. J. C. Valentine and F. E. McDonald, Synlett, 2006, 1816. CrossRef
9. M. Hashimoto, T. Kan, K. Nozaki, M. Yanagiya, H. Shirahama, and T. Matsumoto, Tetrahedron Lett., 1988, 29, 1143; CrossRef M. Hashimoto, T. Kan, K. Nozaki, M. Yanagiya, H. Shirahama, and T. Matsumoto, J. Org. Chem., 1990, 55, 5088; CrossRef I. C. González and C. J. Forsyth, J. Am. Chem. Soc., 2000, 122, 9099. CrossRef
10. E. J. Corey and D. Ha, Tetrahedron Lett., 1988, 29, 3171. CrossRef
11. J. Tanuwidjaja, S.-S. Ng, and T. F. Jamison, J. Am. Chem. Soc., 2009, 131, 12084. CrossRef
12. C. A. Broka, L. Hu, W. J. Lee, and T. Shen, Tetrahedron Lett., 1987, 28, 4993; CrossRef C. A. Broka, and Y. Lin, J. Org. Chem., 1988, 53, 5876; CrossRef G. A. Nishiguchi, and R. D. Little, J. Org. Chem., 2005, 70, 5249; CrossRef G. A. Nishiguchi, J. Graham, A. Bouraoui, R. S. Jacobs, and R. D. Little, J. Org. Chem., 2006, 71, 5936. CrossRef
13. R. P. Hanzlik, Organic Syntheses; Wiley: New York, 1988; Collect. Vol. VI, 560.
14. Z. Wang, Y. Tu, M. Frohn, J. Zhang, and Y. Shi, J. Am. Chem. Soc., 1997, 119, 11224; CrossRef Y. Shi, Acc. Chem. Res., 2004, 37, 488; CrossRef O. A. Wong and Y. Shi, Chem. Rev., 2008, 108, 3958. CrossRef
15. R. Tong, M. A. Boone, and F. E. McDonald, J. Org. Chem., 2009, 74, 8407. CrossRef
16. Trimethylsilyl, para-methoxybenzyl, and tetrahydropyranyl ether protective groups were successfully attached to the tertiary alcohol of 13.
17. This preparation of compound 15 was previously described: F. E. McDonald and X. Wei, Org. Lett., 2002, 4, 593. If the cyclization/resolution was not completely selective (reaction mixture too warm or reaction time too long) the desired bromotetrahydropyran 15 could be separated from the undesired diastereomer by silica column chromatography (eluent hexane : Et2O v/v 3:1), although the yield was lower.. CrossRef
18. J. E. Baldwin, J. Chem. Soc., Chem. Commun., 1976, 734. CrossRef
19. Y. Gao, J. M. Klunder, R. M. Hanson, H. Masamune, S. Y. Ko, and K. B. Sharpless, J. Am. Chem. Soc., 1987, 109, 5765. CrossRef
20. K. Ishihara, H. Kurihara, and H. Yamamoto, J. Org. Chem., 1993, 58, 3791. CrossRef
21. N. Hori, K. Nagasawa, T. Shimizu, and T. Nakata, Tetrahedron Lett., 1999, 40, 2145; CrossRef T. Nakata, S. Nomura, and H. Matsukura, Tetrahedron Lett., 1996, 37, 213; CrossRef S. Takahashi, K. Fujisawa, N. Sakairi, and T. Nakata, Heterocycles, 2000, 53, 1361; CrossRef Y. Sakamoto, M. Koshizuka, H. Koshino, and T. Nakata, Heterocycles, 2002, 56, 113. CrossRef
22. The mesylate could be used in place of the chloromesylate 21, but the rearrangement transformation then required refluxing in aqueous acetic acid. Sc(OTf)3 was a viable alternative to Zn(OAc)2, although longer reaction times were required.
23. W. S. Johnson, J. C. Collins, R. Pappo, M. B. Rubin, P. J. Kropp, W. F. Johns, J. E. Pike, and W. Bartmann, J. Am. Chem. Soc., 1963, 85, 1409. CrossRef
24. M. Yamaguchi and I. Hirao, Tetrahedron Lett., 1983, 24, 391; CrossRef M. J. Eis, J. E. Wrobel, and B. Ganem, J. Am. Chem. Soc., 1984, 106, 3693; CrossRef A. B. Evans and D. W. Knight, Tetrahedron Lett., 2001, 42, 6947. CrossRef
25. F. E. McDonald and J. L. Bowman, Tetrahedron Lett., 1996, 37, 4675; CrossRef J. L. Bowman and F. E. McDonald, J. Org. Chem., 1998, 63, 3680. CrossRef
26. D. F. Taber, R. S. Bhamidipati, and M. L. Thomas, J. Org. Chem., 1994, 59, 3442. CrossRef
27. H. C. Kolb, M. S. VanNieuwenhze, and K. B. Sharpless, Chem. Rev., 1994, 94, 2483. CrossRef
28. P. N. Devine and T. Oh, Tetrahedron Lett., 1991, 32, 883; CrossRef M. A. Robbins, P. N. Devine, and T. Oh, Organic Syntheses; Wiley: New York, 1999; Collect. Vol. 10, 297.
29. J. J. Pappas, W. P. Keaveney, E. Gancher, and M. Berger, Tetrahedron Lett., 1966, 7, 4273. CrossRef
30. J. E. McMurry and W. J. Scott, Tetrahedron Lett., 1983, 24, 979; CrossRef M. W. Gilbert, A. Galkina, and J. Mulzer, Synlett, 2004, 2558. CrossRef
31. P. H. Mee, V. Lee, and J. E. Baldwin, Angew. Chem. Int. Ed., 2004, 43, 1132; CrossRef W. J. Scott and J. E. McMurry, Acc. Chem. Res., 1988, 21, 47; CrossRef L. S. Liebeskind and R. W. Fengl, J. Org. Chem., 1990, 55, 5359; CrossRef V. F. S. Kapadia, B. Krishnan, C. Wang, and L. S. Liebeskind, J. Org. Chem., 1994, 59, 1047; CrossRef L. A. Paquette, T. Wang, and M. R. Sivik, J. Am. Chem. Soc., 1994, 116, 11323; CrossRef A. M. Echavarren, O. de Frutos, N. Tamayo, P. Noheda, and P. Calle, J. Org. Chem., 1997, 62, 4524. CrossRef
32. B. Koo and F. E. McDonald, Org. Lett., 2005, 7, 3621. CrossRef
33. M. Schröder, Chem. Rev., 1980, 80, 187; CrossRef I. Fleming, N. J. Lawrence, A. K. Sarkar, and A. P. Thomas, J. Chem. Soc., Perkin Trans. 1, 1992, 3303. CrossRef
34. MS(FAB+) Calcd. for C32H55O1179Br123Na1 [(M+Na)+] 717.2820, found 717.2807.
35. Tributyltin triflate was freshly prepared by dropwise addition of triflic anhydride (0.03 mL, 0.18 mmol) to neat bistributyltin oxide (0.06 mL, 0.12 mmol). The neat solution was stirred for 1 h, then excess triflic anhydride was removed by vacuum. The white solid was used without purification.
36. Ultrapure CuI was further purified by Soxhlet extraction with THF.
37. Supporting Information is available on the Heterocycles website, http://www.heterocycles.jp/.