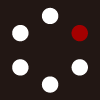
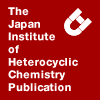
HETEROCYCLES
An International Journal for Reviews and Communications in Heterocyclic ChemistryWeb Edition ISSN: 1881-0942
Published online by The Japan Institute of Heterocyclic Chemistry
e-Journal
Full Text HTML
Received, 14th May, 2012, Accepted, 8th June, 2012, Published online, 15th June, 2012.
DOI: 10.3987/COM-12-12506
■ Synthesis and Evaluation of 4-Aryl-2(1H)-quinolinones as Potent Amyloid β Fibrillogenesis Inhibitors
Yoko Shimokawa, Masamichi Nakakoshi, Setsu Saito, Hideharu Suzuki, Yuusaku Yokoyama, Akihito Ishigami, Hideo Nishioka, and Masayoshi Tsubuki*
Institute of Medicinal Chemistry, Hoshi University, 2-4-41 Ebara, Shinagawa-ku, Tokyo 142-8501, Japan
Abstract
4-Aryl-2(1H)-quinolinones were synthesized and evaluated in vitro as inhibitors of Aβ1-42 fibrillogenesis using a thioflavin T fluorescence method. The most potent anti-aggregating molecules (4b and 5c) were found among the derivatives bearing OH and/or OMe groups at C-4’ (R4) and/or C-6 (R2) of the 4-aryl-2(1H)-quinolinone moiety. Furthermore, the derivative bearing 4’-F substituent (4f) proved to be a very active inhibitor.INTRODUCTION
Alzheimer’s disease (AD) is a progressive, degenerative disease of the brain and recognized as the leading cause of dementia in the aging population. AD is characterized by the aggregation of amyloid β peptides (Aβ) into amyloid plaques in selected areas of brain.1 Aβ is a 39- to 43-residue secretase cleavage product of the amyloid precursor protein (APP). The most common forms in extracellular fluids are a 40-residue fragment (Aβ1-40, ca 90%) and a more aggregation-prone and toxic 42-residue fragment (Aβ1-42, ca 10%). The accumulation of Aβ as amyloid in the brain is caused by an imbalance in the production and clearance of these peptides.2
A number of efforts in searching for inhibitors of Aβ aggregation has demonstrated that small molecules, containing aromatic rings often bearing hydroxy groups, can inhibit protein−protein interactions.3 Recently we have found two types of inhibitors of Aβ, which would be distyrylbenzene derivatives4 and 2-substituted benzofurans.5 In continuation of our focus on developing low-molecular weight inhibitors of Aβ, we were interested in the synthesis of 4-aryl-2(1H)-quinolinones, as structural analogs of recently reported 2-arylbenzofurans.5 Although the quinolinones have wide-ranging biological activities,6 such as anti-malarial6b and anti-bacterial6c activities, there is no report on inhibition of Aβ fibril formation by the quinolinones. We here report the synthesis of 4-aryl-2(1H)-quinolinones and their activity as inhibitors of Aβ1-42 fibirillogenesis using a thioflavin T (ThT) fluorescence assay.7
RESULTS AND DISCUSSION
There are many methods for the synthesis of the 4-aryl-2(1H)-quinolinones based on cyclization approaches,8-10 including Friedländer cyclization,8 Knorr cyclization,9 and transition metal-catalyzed cyclizations.10 We prepared 4-aryl-2(1H)-quinolinones employing the Knorr cyclization of the corresponding benzoylacetoanilides 3a-g as shown in Scheme 1. Amidation between anilines 1a-d and benzoylacetate 2a-c in pyridine gave benzoylacetoanilides 3a-g, which were treated with polyphosphoric acid (PPA) at 95 °C to afford 4-aryl-2(1H)-quinolinones 4a-g in moderate to good yields. Demethylation of 4b-g was achieved by heating with pyridine hydrochloride in either an oil bath or a microwave oven to give phenols 5b-g in 78-98% yields.
The inhibitory effects on the Aβ1-42 fibrillogenesis of 4-aryl-2(1H)-quinolinones 4a-g and 5b-g were evaluated by ThT fluorescence assay. For all the synthesized compounds we measured the percent of fibril formation inhibition. The inhibition data are summarized in Table 1. The core structure, 4-phenyl-2(1H)-quinolinone 4a lacking hydroxyl or methoxy groups, is inactive. With regard to monosubstituted derivatives, the derivatives bearing 4’-OMe (4b), 4’-OH (5b) and 6-OH (5c) groups showed the most potent activities. In general, the derivatives bearing hydroxyl group (5c and 5d) showed higher activities compared to the corresponding methoxy congeners (4c and 4d). It is worth noting that the introduction of 4’-F substituent (5e and 5f) on the phenyl ring of the potent inhibitors (5c and 5d) lost their activities, whereas the respective methoxy derivatives (4e and 4f) improved their activities. The introduction of 6-Cl substituent (4g and 5g) on the phenyl ring of the quinolinone did not exhibit any effect of interest.
The inhibitory behavior of compound 5c was then characterized with transmission electron microscopy (TEM) to assess quantity and shape of fibril. TEM images confirmed the conformational changes observed in ThT fluorescence spectroscopy studies above described (Figure 1).
TEM images provide strong support for the ThT data shown above. They indicate significant difference between inhibitor-free Aβ control sample (Figure-1a) and sample incubated with inhibitor 5c (Figure-1b). While inhibitor-free peptide formed the expected network of long fibers, drastic lack of such aggregates can be observed in the presence of 5c. Thus, we confirmed the antifibrillogenic activity for Aβ fibrillogenesis inhibitors, 4-aryl-2(1H)-quinolinones 4b, 4e, 4f, 5b-d.
CONCLUSION
4-Aryl-2(1H)-quinolinones were synthesized and evaluated in vitro as inhibitors of Aβ1-42 fibrillogenesis using a ThT fluorescence method. The most potent anti-aggregating molecules (4b and 5c) were found among the derivatives bearing OH and/or OMe groups at C-4’ (R4) and/or C-6 (R2) of the 4-aryl-2(1H)-quinolinone moiety. Furthermore, the derivative bearing 4’-F substituent (4f) proved to be a very active inhibitor. Thus, the 4-aryl-2(1H)-quinolinone would be a scaffold for a new class of low-molecular weight inhibitors of Aβ1-42 aggregation. Efforts to understand inhibition mechanism of this class of compounds will be the focus of future efforts.
EXPERIMENTAL
Chemistry
IR spectra were obtained using a Shimadzu FT/IR Prestige-21 spectrophotometer. 1H- and 13C-NMR spectra were obtained on a Bruker AV 400 spectrometer, and chemical shifts were referenced to the residual solvent peaks (δ H 7.26 and δ C 77.0 for CDCl3, and δ H 2.49 and δ C 39.7 for DMSO-d6). MS spectra were measured with a JEOL JMS-T100LP spectrometer. Melting points were determined on a Yanaco micro melting apparatus MP-J3 and are uncorrected.
Known 4-aryl-2(1H)-quinolinones (4a,11 4b,9b 4c12) were prepared from 3a-c according to the literature.9
General procedure for amidation between anilines 1a-d and 3-aryl-oxopropanoates 2a-c
A mixture of aniline 1 (1.0 mmol) and ethyl 3-aryl-3-oxopropanoate 2 (1.0-1.8 mmol) in pyridine (0.25 mmol) was refluxed for 4-24 h. Evaporation of solvent gave a residue, which was purified by silica gel column chromatography (hexane/EtOAc) to afford solid. The solid was recrystallized from EtOAc.
[N-(3,4,5-Trimethoxyphenyl)-β-(4-methoxyphenyl)-β-oxo]propanamide 3d
Reaction of 1c and 2b gave 3d in 53% yield; mp 128-129 °C (EtOAc); 1H NMR (400 MHz, CDCl3) δ (ppm): 3.81 (s, 3H), 3.86 (s, 6H), 3.90 (s, 3H), 4.05 (s, 2H), 6.89 (s, 2H), 6.99 (ddd, 2H, J = 9.0, 2.8, 2.0 Hz), 8.02 (ddd, 2H, J = 9.0, 2.8, 2.0 Hz), 9.38 (s, 1H). 13C NMR (100 MHz, CDCl3) δ (ppm): 45.1, 55.6, 56.0, 60.9, 97.6, 114.1, 128.9, 131.1, 133.8, 134.6, 153.2, 164.0, 164.6, 194.8. IR (thin film) νmax 3327, 2939, 1602, 1508, and 1129 cm–1; HRESITOFMS m/z 360.1489 (M++H; calcd for C19H22NO6, 360.1447).
[N-(p-Methoxyphenyl)-β-(p-fluorophenyl)-β-oxo]propanamide 3e
Reaction of 1b and 2c gave 3e in 53% yield; mp 141 °C (EtOAc); 1H NMR (400 MHz, CDCl3) δ (ppm): 3.79 (s, 3H), 4.07 (s, 2H), 6.86 (ddd, 2H, J = 8.8, 3.6, 2.0 Hz), 7.18 (dddd, 2H, J = 9.0, 8.4, 2.8, 2.0 Hz), 7.47 (ddd, 2H, J = 8.8, 3.6, 2.0 Hz), 8.08 (dddd, 2H, J = 9.0, 5.4, 2.8, 2.0 Hz), 9.04 (s, 1H). 13C NMR (100 MHz, CDCl3) δ (ppm): 45.6, 55.5, 114.1, 116.2 (d, J = 87.6 Hz), 121.9, 130.6, 131.5 (d, J = 38.4 Hz), 132.5 (d, J = 11.6 Hz), 156.6, 167.7, 194.8. IR (thin film) νmax 3311 and 1509 cm–1; HRESITOFMS m/z 288.1053 (M++H; calcd for C16H15FNO3, 288.1036).
[N-(3,4,5-Trimethoxyphenyl)-β-(p-fluorophenyl)-β-oxo]propanamide 3f
Reaction of 1c and 2c gave 3f in 78% yield; mp 160 °C (EtOAc); 1H NMR (400 MHz, CDCl3) δ (ppm): 3.81 (s, 3H), 3.86 (s, 6H), 4.08 (s, 2H), 6.87 (s, 2H), 7.20 (dddd, 2H, J = 9.0, 8.4, 2.8, 2.0 Hz), 8.08 (dddd, 2H, J = 9.0, 5.4, 2.8, 2.0 Hz), 9.12 (s, 1H). 13C NMR (100 MHz, CDCl3) δ (ppm): 45.7, 56.1, 60.9, 97.6, 116.2 (d, J = 87.6 Hz), 131.4 (d, J = 38.4 Hz), 132.4 (d, J = 11.2 Hz), 133.6, 134.7, 153.2, 163.5, 165.2, 167.7, 194.7. IR (KBr) νmax 3352, 1669, 1510, and 1131 cm–1; HRESITOFMS m/z 348.1251 (M++H; calcd for C18H19FNO5, 348.1247).
[N-(4-Chloro-3-methoxyphenyl)-β-(p-methoxyphenyl)-β-oxo]propanamide 3g
Reaction of 1d and 2b gave 3g in 73% yield; mp 134-136 °C (EtOAc); 1H NMR (400 MHz, CDCl3) δ (ppm): 3.90 (s, 3H), 3.91 (s, 3H), 4.05 (s, 2H), 6.98 (d, 2H, J = 8.8 Hz), 7.00 (dd, 8.8, 2.4 Hz), 7.28 (d, 8.8 Hz), 7.48 (d, 2.4 Hz), 8.01 (d, 8.8 Hz), 9.58 (s, 1H). 13C NMR (100 MHz, CDCl3) δ (ppm): 44.7, 55.6, 56.2, 104.5, 112.4, 114.2, 117.5, 128.9, 130.0, 131.1, 137.5, 155.1, 164.1, 164.7, 194.9. IR (KBr) νmax 3339, 2926, 1695, and 1604 cm–1; HRESITOFMS m/z 334.0880 (M++H; calcd for C17H17ClNO4, 334.0846).
General procedure for cyclization of propanamides 3d-g
A mixture of propanamide 3 (1.0 mmol) and polyphosphoric acid (4.0 g) was heated at 95 °C for 1.5-24 h. The mixture was poured into ice water and the precipitated solid was filtered and washed with ice water. The solid was recrystallized from EtOH.
5,6,7-Trimethoxy-4-(p-methoxyphenyl)-2(1H)-quinolinone 4d
Cyclization of 3d gave quinolinone 4d in a quantitative yield; mp 278 °C (EtOH); 1H NMR (400 MHz, CDCl3) δ (ppm): 3.27 (s, 3H), 3.81 (s, 3H), 3.87 (s, 3H), 3.98 (s, 3H), 6.38 (s, 1H), 6.73 (s, 1H), 6.93 (ddd, 2H, J = 8.8, 2.8, 2.0 Hz), 7.29 (ddd, 2H, J = 8.8, 2.8, 2.0 Hz), 12.59 (s, 1H). 13C NMR (100 MHz, CDCl3) δ (ppm): 55.3, 56.2, 60.9, 61.1, 94.4, 108.6, 112.6, 120.0, 129.0, 133.2, 136.8, 138.7, 151.0, 152.5, 156.6, 159.0, 163.7. IR (KBr) νmax 2961, 1654, 1613, 1360, 1244, and 1118 cm–1; HRESITOFMS m/z 342.1377 (M++H; calcd for C19H20NO5, 342.1342).
6-Methoxy-4-(p-fluorophenyl)-2(1H)-quinolinone 4e
Cyclization of 3e gave quinolinone 4e in 67% yield; mp 289 °C (EtOH); 1H NMR (400 MHz, DMSO-d6) δ (ppm): 3.64 (s, 3H), 6.39 (s, 1H), 6.78 (d, 1H, J = 2.4 Hz), 7.22 (dd, 1H, J = 8.8, 2.4 Hz), 7.34 (d, 1H, J = 8.8 Hz), 7.36 (dd, 2H, J = 9.2, 8.8 Hz), 7.53 (dd, 2H, J = 8.8, 5.0 Hz), 11.80 (s, 1H). 13C NMR (100 MHz, DMSO-d6) δ (ppm): 55.5, 108.2, 115.9 (d, J = 86.0 Hz), 117.3, 119.0, 119.5, 122.2, 131.1 (d, J = 32.8 Hz), 133.3 (d, J = 12.4 Hz), 134.0, 150.1, 154.3, 161.0, 161.3, 163.8. IR (KBr) νmax 2837, 1655, 1498, 1422 and 1226 cm–1; HRESITOFMS m/z 270.0929 (M++H; calcd for C16H13FNO2, 270.0930).
4-(p-Fluorophenyl)- 5,6,7-trimethoxy-2(1H)-quinolinone 4f
Cyclization of 3f gave quinolinone 4f in 79% yield; mp 282-283 °C (EtOH); 1H NMR (400 MHz, CDCl3) δ (ppm): 3.26 (s, 3H), 3.78 (s, 1H), 3.95 (s, 3H), 6.37 (s, 1H), 6.81 (s, 1H), 7.07 (s, 2H), 7.30 (s, 2H), 13.22 (brs, 1H). 13C NMR (100 MHz, CDCl3) δ (ppm): 56.3, 60.7, 61.0, 94.6, 108.5, 114.2 (d, J = 86.0 Hz), 119.5, 129.3 (d, J = 31.6 Hz), 136.7 (d, J = 12.4 Hz), 136.8, 138.8, 150.7, 151.8, 156.8, 160.9, 163.4, 163.6. IR (KBr) νmax 3432, 2931, and 1667 cm–1; HRESITOFMS m/z 330.1137 (M++H; calcd for C18H17FNO4, 330.1142).
6-Chloro-4-(p-methoxyphenyl)-7-methoxy-2(1H)-quinolinone 4g
Cyclization of 3g gave quinolinone 4g in 90%; mp > 300 °C (EtOH); 1H NMR (400 MHz, DMSO-d6) δ (ppm): 3.83 (s, 3H), 3.90 (s, 3H), 6.24 (s, 1H), 7.04 (s, 1H), 7.10 (d, 2H, J = 8.8 Hz), 7.34 (s, 1H), 7.40 (d, 2H, J = 8.8), 11.82 (s, 1H). 13C NMR (100 MHz, DMSO-d6) δ (ppm): 55.5, 56.5, 98.9, 113.0, 114.5, 115.8, 119.1, 126.8, 128.7, 130.1, 140.1, 150.4, 156.1, 160.0, 161.7. IR (KBr) νmax 3446, 2955, 1666, 1609, and 1252 cm–1; HRESITOFMS m/z 316.0781 (M++H; calcd for C17H15ClNO3, 316.0741).
Demethylation of aryl methyl ethers 4b-g
Method A (Heating in a microwave oven)
A mixture of 2(1H)-quinolinone (4b, 4c and 4f; 100 mg) and pyridine hydrochloride (1.0 g) was heated in a microwave at 210 °C for 20 min. The mixture was poured into water. The precipitates were filtered to afford a brown crude product. The solid was recrystallized from EtOH.
Method B (Heating in an oil bath)
A mixture of 2(1H)-quinolinone (4d, 4e and 4g; 100 mg) and pyridine hydrochloride (1.0 g) was heated in an oil bath at 225 °C for 15-50 min. The same workup as Method A was carried out.
4-p-Hydroxyphenyl-2(1H)-quinolinone 5b
98% yield; mp > 300 °C (EtOH); 1H NMR (400 MHz, DMSO-d6) δ (ppm): 6.31 (s, 1H), 6.90 (d, 2H, J = 8.4 Hz), 7.13 (dd, 1H, J = 7.6, 7.4 Hz), 7.29 (d, 2H, J = 8.4 Hz), 7.36 (d, 1H, J = 7.8 Hz), 7.47 (d, 1H, J = 8.4 Hz), 7.50 (1H, dd, J = 7.8, 7.6 Hz), 11.79 (s, 1H). 13C NMR (100 MHz, DMSO-d6) δ (ppm): 115.7, 116.0, 118.8, 120.8, 121.9, 126.5, 127.4, 130.3, 130.6, 139.5, 151.8, 158.3, 161.6. IR (KBr) νmax 3010 and 1658 cm–1; HRESITOFMS m/z 238.0837 (M++H; calcd for C15H12NO2, 238.0868).
6-Hydroxy-4-phenyl-2(1H)-quinolinone 5c
92% yield; mp 273 °C (decomp.) (EtOH); 1H NMR (400 MHz, DMSO-d6) δ (ppm): 6.32 (s, 1H), 6.75 (d, 1H, J = 2.8 Hz), 7.01 (dd, 1H, J = 8.8, 2.8 Hz), 7.24 (d, 1H, J = 8.8 Hz), 7.43-7.56 (m, 5H), 9.33 (s, 1H), 11.68 (s, 1H). 13C NMR (100 MHz, DMSO-d6) δ (ppm): 110.1, 117.1, 119.4, 120.2, 121.6, 128.8, 128.8, 128.9, 132.8, 137.3, 151.1, 152.3, 161.0. IR (KBr) νmax 3453, 3000, 1652, 1399, and 1275 cm–1; HRESITOFMS m/z 238.0849 (M++H; calcd for C15H12NO2, 238.0868).
5,6,7-Trihydroxy-4-(p-hydroxyphenyl)-2(1H)-quinolinone 5d
84% yield; mp > 300 °C (EtOH); 1H NMR (400 MHz, DMSO-d6) δ (ppm): 5.78 (s, 1H), 6.39 (s, 1H), 6.71 (d, 2H, J = 8.2 Hz), 7.08 (d, 2H, J = 8.2 Hz), 8.32 (s, 1H), 9.42 (s, 1H), 10.06 (s, 1H), 11.35 (s, 1H). 13C NMR (100 MHz, DMSO-d6) δ (ppm): 92.5, 102.6, 114.2, 118.1, 128.0, 129.3, 132.5, 134.8, 144.2, 150.3, 151.8, 156.9, 161.5. IR (KBr) νmax 3368, 3148, and 1648 cm–1; HRESITOFMS m/z 286.0710 (M++H; calcd for C15H12NO5, 286.0716).
6-Hydroxy-4-(p-fluorophenyl)-2(1H)-quinolinone 5e
87% yield; mp > 300 °C (EtOH); 1H NMR (400 MHz, DMSO-d6) δ (ppm): 6.33 (s, 1H), 6.72 (s, 1H), 7.02 (d, 1H, J = 8.6 Hz), 7.25 (d, 1H, J = 8.6 Hz), 7.37 (dd, 2H, J = 8.8, 8.0 Hz), 7.50 (dd, 2H, J = 8.0, 5.6 Hz), 11.69 (s, 1H). 13C NMR (100 MHz, DMSO-d6) δ (ppm): 110.0, 115.8 (d, J = 86.0 Hz), 117.1, 119.4, 120.3, 121.8, 131.0 (d, J = 33.2 Hz), 132.8, 133.6 (d, J = 11.6 Hz), 150.0, 152.3, 161.0, 161.3, 163.7. IR (KBr) νmax 3043, 1656, and 1505 cm–1; HRESITOFMS m/z 256.0774 (M++H; calcd for C15H11FNO2, 256.0774).
4-(p-Fluorophenyl)- 5,6,7-trihydroxy-2(1H)-quinolinone 5f
78% yield; mp > 300 °C (EtOH); 1H NMR (400 MHz, DMSO-d6) δ (ppm): 5.78 (s, 1H), 6.39 (s, 1H), 7.14 (dddd, 2H, J = 9.2, 8.8, 2.8, 2.4 Hz), 7.29 (dddd, 2H, J = 8.8, 5.6, 2.8, 2.4 Hz), 8.20 (brs, 1H), 8.42 (s, 1H), 10.08 (s, 1H), 11.38 (s, 1H). 13C NMR (100 MHz, DMSO-d6) δ (ppm): 92.5, 102.3, 114.1 (d, J = 84.8 Hz), 118.4, 128.1, 130.0 (d, J = 32.4 Hz), 134.7, 138.2 (d, J = 13.2 Hz), 143.9, 150.4, 150.5, 160.4, 161.1, 162.8. IR (KBr) νmax 3306, 2935, 1648, 1507, 1412, and 1312 cm–1; HRESITOFMS m/z 310.0491 (M++H; calcd for C15H10FNO4Na, 310.0492).
6-Chloro-4-(p-hydroxyphenyl)-7-hydroxy-2(1H)-quinolinone 5g
92% yield; mp > 300 °C (EtOH); 1H NMR (400 MHz, DMSO-d6) δ (ppm): 6.12 (s, 1H), 6.90 (ddd, 2H, J = 8.4, 2.8, 1.8 Hz), 6.98 (s, 1H), 7.26 (ddd, 2H, J = 8.4, 2.8, 1.8 Hz), 7.30 (s, 1H), 9.82 (s, 1H), 11.03 (s, 1H), 11.71 (s, 1H). 13C NMR (100 MHz, DMSO-d6) δ (ppm): 101.9, 112.8, 115.5, 115.8, 118.0, 127.2, 127.4, 130.2, 140.0, 151.1, 155.2, 158.4, 162.1. IR (KBr) νmax 3323, 3125, 1657, 1610, 1400, 1256, and 1245 cm–1; HRESITOFMS m/z 288.0435 (M++H; calcd for C15H11ClNO3, 288.0428).
Biological assay
Inhibition of Aβ1-42 fibrillogenesis by synthetic compounds 4a-g and 5b-g
Aβ1-42 (Peptide Institute, Japan) 0.5 mg was dissolved in HIPF (0.5 mL). This solution was treated by the supersonic wave for 10 min and concentrated at 60 °C in vacuo. The Aβ1-42 (0.5 mg) was dissolved in DMSO (0.5 mL) and this solution was stocked at -20 °C. Aβ1-42 (5 µM) was incubated (37 °C, 24 h) with quinolinone (20 µM) in Tris–HCl (pH 7.6, 50 mM). Control was incubated without quinolinone under the same conditions. Incubations were run in quintuplicate.
Thioflavin T (ThT) fluorescence assay
To perform ThT fluorescence assay on our samples, we followed the well-established protocol of H. Levine III.7 The solution of ThT (1 µM) used for fluorimetric measures was prepared in water. For each measurement, ThT solution (0.2 µmol) was added. Fluorescent measurements were made using JASCO FP-6500 spectrofluorimeter at 25 °C. The measurement condition was the emission wavelength: 480 nm and the excitation wavelength: 450 nm.
Observation of Transmission Electron Microscope (TEM)
Samples of inhibition of Aβ1-42 aggregation and fibril formation of Aβ1-42 fibril were observed by a JEOL JEM-1400F transmission electron microscope at an accelerating voltage of 80 kV. The negative staining used the 2% ytterbium triacetate solution on carbon-coated copper grids (400 mesh).
ACKNOWLEDGEMENTS
This work was supported in part by the Science Research Promotion Fund from the Promotion and Mutual Aid Corporation for Private Schools of Japan and by a Grant-in-Aid for Scientific Research from the Ministry of Education, Culture, Sports, Science and Technology of Japan.
References
1. J. C. Rochet and P. T. Lansbury, Curr. Opin. Struct. Biol., 2000, 10, 60. CrossRef
2. J. Hardy and D. J. Selkoe, Science, 2002, 297, 353. CrossRef
3. H. Levine, III, Amyloid, 2007, 14, 185. CrossRef
4. H. Suzuki, A. Ishigami, A. Orimoto, A. Matsuyama, S. Handa, N. Maruyama, Y. Yokoyama, H. Okuno, and M. Nakakoshi, Chem. Pharm. Bull., accepted.
5. H. Suzuki, S. Yajima, M. Nakakoshi, M. Tsubuki, A. Ishigami, H. Hikawa, and Y. Yokoyama, The 132nd Annual Meeting of the Pharmaceutical Society of Japan; Abstract, 2012, 31E09-pm10S.
6. a) H. J. Roth and H. Fenner, in Arzneistoffe, 3rd ed., Deutscher Apotheker Verlag, Stuttgart, 2000, pp. 51-114; b) S. Krishna and N. J. White, Clin. Pharmacokinet., 1996, 30, 263; CrossRef c) S. E. Hagen, J. M. Domagala, C. L. Heifetz, J. P. Sanchez, and M. Solomon, J. Med. Chem., 1990, 33, 849; CrossRef d) P. Hewawasam, W. Fan, M. Ding, K. Flint, D. Cook, G. D. Goggins, R. A. Myers, V. K. Gribkoff, C. G. Boissard, S. I. Dworetrzky, J. E. Starrett, and N. J. Lodge, J. Med. Chem., 2003, 46, 2819. CrossRef
7. H. LeVine, III, Methods Enzymol., 1999, 309, 274. CrossRef
8. C.-C. Cheng and S.-J. Yan, Org. React., 1982, 28, 37.
9. a) S. Nakano, Yakugaku Zasshi, 1962, 82, 492; b) Y. Kitahara, S. Nakahara, M. Shimizu, T. Yonezawa, and A. Kubo, Heterocycles, 1993, 36, 1909. CrossRef
10. a) C. Jia, D. Piao, J. Oyamada, W. Lu, T. Kitamura, and Y. Fujiwara, Science, 2000, 287, 1992; CrossRef b) R. Bernini, S. Cacchi, I. D. Salve, and G. Fabrizi, Synlett, 2006, 2947; CrossRef c) K. Inamoto, T. Saito, K. Hiroya, and T. Doi, J. Org. Chem., 2010, 75, 3900. CrossRef
11. B. Staskun and S. S. Islaelstam, J. Org. Chem., 1964, 29, 1153. CrossRef
12. K. Hino, K. Kawashima, M. Oka, H. Uno, and J. Matsumoto, Chem. Pharm. Bull., 1989, 37, 190. CrossRef