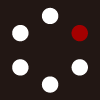
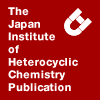
HETEROCYCLES
An International Journal for Reviews and Communications in Heterocyclic ChemistryWeb Edition ISSN: 1881-0942
Published online by The Japan Institute of Heterocyclic Chemistry
e-Journal
Full Text HTML
Received, 7th July, 2012, Accepted, 13th August, 2012, Published online, 20th August, 2012.
DOI: 10.3987/COM-12-S(N)93
■ CHIRAL PRIMARY AMINO SILYL ETHER ORGANOCATALYST FOR THE ENANTIOSELECTIVE DIELS-ALDER REACTION OF 1,2-DIHYDROPYRIDINES WITH AlDEHYDES
Yuki Sakuta, Yoshihito Kohari, N. D. M. Romauli Hutabarat, Koji Uwai, Eunsang Kwon, Yuko Okuyama, Chigusa Seki, Haruo Matsuyama, Nobuhiro Takano, Michio Tokiwa, Mitsuhiro Takeshita, and Hiroto Nakano*
Department of Applied Chemistry, Graduate School of Engineering, Muroran Institute of Technology, 27-1 Mizumoto, Muroran, Hokkaido 050-8585, Japan
Abstract
The enantioselective Diels-Alder reactions of 1,2-dihydropylidines with acroleins using a chiral primary amino silyl ether organocatalyst afforded chiral isoquinuclidines with a good chemical yield and excellent enantioselectivity (up to 70%, up to 99% ee).INTRODUCTION
Asymmetric organocatalysis has emerged as an important and rapidly growing area of synthetic organic Asymmetric organocatalysis has emerged as an important and rapidly growing area of synthetic organic chemistry, and excellent covalent and non-covalent organocatalysts have been developed for use in a wide range of reactions.1 Recently, we also developed a covalent-type oxazolidine and β-amino alcohol organocatalyst2 that works as an efficient catalyst in the asymmetric Diels-Alder (DA) reaction of 1,2-dihydropyridines3 with acroleins, which is an important reaction for the construction of chiral isoquinuclidines (2-azabicyclo[2.2.2]octanes). Isoquinuclidines are found widely in natural products such as iboga-type alkaloids, which have varied and interesting biological properties (Scheme 1).4 In particular, there are the anti-cancer drugs, vinblastine and vincristine, which possess isoquinuclidines, with the aspidosperma portion.5 Furthermore, it has recently been indicated that ibogaine reduces cravings for alcohol and other drugs by means of its ability to boost the levels of a growth factor known as glial cell line-derived neurotrophic factor (GDNF).6 Most recently it has also been shown that the isoquinuclidines can be used as the synthetic intermediates for the synthesis of oseltamivir phosphate (Tamiflu) which is an important anti-influenza drug.7 Therefore, it is meaningful to establish an effective catalytic asymmetric synthetic methodology for chiral isoquinuclidines using an organocatalyst.
We report herein that primary amino silyl ether with CF3CO2H is an efficient organocatalyst for the DA reaction of 1,2-dihydropyridines with acroleins affording chiral isoquinuclidines at good chemical yields (up to 65%) and excellent enantioselectivities (up to 99% ee).
Concerning the concept for catalyst design, it is suggested that the formation of the iminium ion intermediate from the reaction of an amino silyl ether catalyst with acroleins might be able to control the attack of a diene that was fixed by the hydrogen bonding interactions between the hydrogen on the iminium ion intermediate and the carbonyl group on 1,2-dihydropyridines. And therefore, the steric influence of the bulky diphenyl silyloxy group at the 1-position and a substituent at the 2-position in the iminium ion intermediate might also effectively to afford high enantioselectivity in the reaction (Scheme 2).
RESULTS AND DISCUSSION
Catalysts 3a-i, respectively, were easily prepared by the reactions of the corresponding β-amino alcohols with XOTf (X= TMS, TES, and TIPS) followed by treatment with an organic acid (Scheme 3). Thus, the silylations of the β-amino alcohols 1a-g, respectively, afforded the corresponding precursor amino silyl ethers 2a-g in good to moderate chemical yields. The treatments of 2a-g with YCO2H (Y= CF3, CCl3, CH3) afforded the desired amino silyl ether salt catalysts 3a-i in quantitative yields. Proline-based amino silyl ether8-CF3CO2H salt catalyst 4 was also obtained by the treatment of the precursor proline-based amino silyl ether with CF3CO2H under the same reaction conditions.
We first examined the DA reaction of common 1-phenoxycarbonyl-1,2-dihydropyridine 5a with acrolein 6. The reaction of 5a (1 equiv) with 6 (3 equiv) was carried out at 0 °C in CH3CN-H2O (19:1)2 in the presence of 10 mol% of catalysts 3a-i to give the DA adduct 7, and its chemical and optical yields were determined by conversion to the alcohol 8. The results are summarized in Table 1. The reaction catalyzed by 2-methyl-1-OTMS 3a gave the DA adduct 7 in low chemical yield and with moderate enantioselectivity (entry 1). The use of 2-isopropyl-1-OTMS 3b brought about an increase in both chemical yield (53%) and enantioselectivity (endo-7a: 91% ee) (entry 2). Bulkier 2-tert-butyl-1-OTMS catalyst 3c gave the endo-DA adduct 7a in good chemical yield (55%) and with excellent enantioselectivity (95% ee) (entry 3). In contrast, the catalytic activity of sterically crowded 2-phenyl-1-OTMS 3d was low and those reactions proceeded slightly with exo-DA adduct 3b (entry 4). On the other hand, more sterical larger 2-benzyl-1-OTMS 3e showed a high level of catalytic activity to afford the endo-DA adduct 7a at 56% and 85% ee (entry 5), although the reasons for this are unclear. Furthermore, the use of 2-tert-butyl-1-OTMS catalyst 3f with CH3CO2H did not show the catalytic activity in the reaction (entry 6). In addition, the activity of 2-tert-butyl-1-OTMS catalyst 3f with CCl3CO2H was also examined, but the chemical yield was not satisfactory, although the endo-DA adduct 7a was obtained with good enantioselectivity (entry 7). We next examined the efficiencies of the catalysts 3h,i having bulkier OTES and OTIPS at the 1-position on amino silyl ether (entries 8,9).
Although these catalysts 3h,i brought about fairly good asymmetric inductions, the chemical yields were poor. The catalytic activity of common proline-based organocatalyst 4 with CF3CO2H in this reaction was also tested. However the catalyst did not afford a better result than did primary amino silyl ether 3c (entry 10). The above results indicate that primary amino silyl ether was the efficient catalyst in this DA reaction. These results indicate that the existence of the OTMS group as a trisubstituted silyloxy group at the 1-position on amino silyl ether is important for obtaining a satisfactory enantioselectivity and chemical yield. To optimize the reaction conditions using superior catalysts 3c, we next examined the effect of reducing the molar ratio of 3c. Although the reaction mixtures containing 5 mol% of 3c afforded fairly good enantioselectivity (endo-7a: 92% ee), the chemical yield decreased to 40% (entry 11). Lower catalytic loading to 2.5 mol% greatly decreased the chemical yield (20%) (entry 12). A dramatic increase in chemical yield to 70% was achieved with excellent enantioselectivity (95% ee), when the reaction time was changed from 24 h to 48 h (entry 13). Similarly, synthetically useful 1,2-dihydropyridine 5b was also examined using superior catalyst 3c and dienophile 6 (Scheme 14). The reaction was carried out at 0 °C for 24 h in the presence of 10 mol% of the best catalyst 3c to give the corresponding endo-DA adduct 9a. The chemical and optical yields of the DA adduct 9 were determined by converting to the alcohol 10. As a result, good chemical yield and enantioselectivity (68%, 96% ee) were obtained using catalyst 3c. These results indicated that 5-tert-butyl-1-OTMS catalyst 3c with CF3CO2H were the most effective in the DA reactions of dienes 5a,b with 6 to afford the DA adducts 7a, 9a, respectively, with good chemical yields and excellent enantioselectivities (7a: 70%, 95% ee, 9a: 68%, 96% ee). However, the best catalyst 3c did not afford a better result than our corresponding previously reported β-amino alcohol organocatalyst 1c with CF3CO2H2a in the same DA reaction. It might be reason that bulkier trimethylsilyloxy group on 3c was act negatively for obtaining the DA adducts 7a or 9a with an excellent chemical yield and enantioselectivity.
Based on both the high enantiopurity (95% ee) of the chiral DA adduct (7S)-7a that was obtained from the reaction of diene 5a with dienophile 6 and Isihara’s detail study9 for an amino organocatalized DA reaction mechanism, a model of the enantioselective reaction was proposed as follows (Scheme 4). Thus, the reaction might be through the transition state A that was fixed by the hydrogen bonding interactions between the hydrogen on the iminium ion intermediate and the carbonyl group on 1,2-dihydropyridines. Then the diene might attack from the re-face of the olefin part on the iminium ion intermediate rather than the sterically crowded si-face that was masked by the combination of the bulky diphenyl trimethylsilyloxy group at the 1-position and the tert-butyl group at the 2-position in the iminium ion intermediate (transition state B).
The effectiveness of superior 2-tert-butyl-1-OTMS catalyst 3c was then evaluated in the DA reaction using acrolein derivative 11 (Scheme 5). The reactions of dienes 5a or 5b with dienophile 11 were carried out at 0 °C in the presence of 10 mol% of superior catalyst 3c to give the DA adducts 12 or 13. The chemical and optical yields of 12 or 13 were determined by conversion to alcohols 14 or 15, respectively. In both reactions, catalyst 3c showed satisfactory asymmetric catalytic activity and the desired DA adducts 12 or 13 were obtained in good chemical yields and with excellent enantioselectivities (12: 65%, 99% ee, 13: 60%, 97% ee).
In conclusion, new amino silyl ether organocatalyst 3 was explored. Catalyst 3 was easily prepared in two steps. Organocatalyst 3c, in particular, showed efficient asymmetric catalytic activity in the Diels-Alder reactions of 1,2-dihydropyridines 5a,b with acroleins 6, 11 to afford the chiral isoquinuclidines 7, 9, 12, 23, respectively. Further studies are in progress to examine the scope and limitations of this organocatalyst for the catalytic asymmetric version of the DA reactions of 1,2-dihydropyridines.
EXPERIMENTAL
IR spectra were measured with a PERKIN ELMER 1725X spectrophotometer. 1H-NMR spectra were recorded on a JEOL JNM-GSX 400 and a JEOL JNM-LA 600 spectrometers with TMS as an internal standard. MS were taken on a Hitachi RMG-6MG and a JEOL-JNM-DX 303 spectrometers. Optical rotations was measured with a JASCO-DIP-370 digital polarimeter.
General procedure for the preparation of amino silyl ethers 2b,c,f,g
To a solution of amino alcohols 1a-e (2.0 mmol) in CH2Cl2 (30 mL) were added trisubstituted silyl trifluoromethanesulfonates (2.4 mmol) and Et3N (2.4 mmol) at -30 °C. The mixture was stirred for 24 min at room temperature. Water (10 mL) was added, the aqueous layer was extracted with CHCl3 (2 x 30 mL) and organic phases were dried over MgSO4 and evaporated to give a crude products 2a-g. The residue was purified by column chromatography (SiO2, CHCl3) to give a pure products 2a-g (a: 299 mg, 50%, b: 498 mg, 76%, c: 546 mg, 80%, d: 333 mg, 46%, e: 511 mg, 68%, f: 223 mg, 29%, g: 204 mg, 24%).
(S)-3-Methyl-1,1-diphenyl-1-(trimethylsilyloxy)butan-2-amine (2b)
Colorless oil. [α]D25 129.99 (c 0.30, CHCl3); IR (KBr) cm-1: 699, 836, 1069, 1250, 1493, 2954; 1H-NMR (CDCl3) δ: 7.44-7.26 (m, 10H, Ph), 3.57 (s, 1H, NCH), 1.92 (s, 1H, CH(CH3)2), 1.03 (d, J = 6.9 Hz, 6H, CH(CH3)2), 0.17 (d, J = 6.9 Hz, 6H, CH(CH3)2), -0.18 (s, 9H, Si(CH3)3); 13C-NMR (CDCl3) δ:128.7, 128.6, 127.7, 127.5, 127.1, 84.5, 61.5, 27.0, 23.6, 15.8, 1.8 ; Anal. Calcd for C20H29NOSi: C, 73.34; H, 8.92; N, 4.28. Found: C, 73.15; H, 9.10; N, 4.16. EI-MS m/z: 327 (M+).
(S)-3,3-Dimethyl-1,1-diphenyl-1-(trimethylsilyloxy)butan-2-amine (2c)
Colorless oil. [α]D26 106.66 (c 0.30, CHCl3); IR (KBr) cm-1: 700, 835, 1072, 1250, 1494, 1738, 2952; 1H-NMR (CDCl3) δ: 7.58-7.26 (m, 10H, Ph), 3.61 (s, 1H, NCH), 0.72 (s, 9H, C(CH3)3), -0.21 (s, 9H, Si(CH3)3); 13C-NMR (CDCl3) δ:128.9, 128.6, 128.1, 127.5, 127.1, 126.8, 85.1, 65.1, 35.5, 28.8, 1.8; Anal. Calcd for C21H31NOSi: C, 73.84; H, 9.15; N, 4.10. Found: C, 73.75; H, 9.21; N, 4.02. EI-MS m/z: 342 (M+).
(S)-3,3-Dimethyl-1,1-diphenyl-1-(triethylsilyloxy)butan-2-amine (2f)
Colorless oil. [α]D28 109.99 (c 0.30, CHCl3); IR (KBr) cm-1: 701, 850, 1069, 1230, 1494, 1738 2952; 1H-NMR (CDCl3) δ: 7.62-7.26 (m, 10H, Ph), 3.64 (s, 1H, NCH), 0.83 (t, J = 8.0 Hz, 9H, Si(CH2CH3)3), 0.72 (s, 9H, C(CH3)3), 0.24 (qq, J = 7.4 Hz, J = 8.3 Hz, 6H, Si(CH2CH3)3); 13C-NMR (CDCl3) δ:128.9, 128.7, 128.0, 127.7, 127.0, 126.8, 85.0, 65.5, 35.5, 28.8, 7.3, 6.0; Anal. Calcd for C24H37NOSi: C, 75.14; H, 9.72; N, 3.65. Found: C, 75.02; H, 9.81; N, 3.54. EI-MS m/z: 383 (M+).
(S)-3,3-Dimethyl-1,1-diphenyl-1-(triisopropylsilyloxy)butan-2-amine (2g)
Colorless oil. [α]D28 116.66 (c 0.30, CHCl3); IR (KBr) cm-1: 704, 880, 1052, 1229, 1464, 1738, 2945; 1H-NMR (CDCl3) δ: 7.72-7.26 (m, 10H, Ph), 3.74 (s, 1H, NCH), 1.05 (s, 18H, Si(CH(CH3)2)3), 0.99 (d, J = 7.4 Hz, 18H, Si(CH(CH3)2)3), 0.90 (d, J = 7.4 Hz, 18H, Si(CH(CH3)2)3), 0.72 (s, 9H, C(CH3)3), 0.66 (dd, J = 7.4 Hz, J = 7.7Hz, 3H, Si(CH(CH3)2)3); 13C-NMR (CDCl3) δ:129.4, 129.1, 127.9, 126.8, 85.9, 66.2, 35.4, 28.9, 19.0, 14.2; Anal. Calcd for C27H43NOSi: C, 76.17; H, 10.18; N, 3.29. Found: C, 76.01; H, 10.29; N, 3.14. EI-MS m/z: 425 (M+).
General procedure for the preparation of amino silyl ether・CF3CO2H organocatalysts 3a-i
To a CH2Cl2 (1.8 mL) solution of amino silyl ether 2a-g (0.03 mmol), trifluoroacetic acid (0.036 mmol) was added at 0 °C. The resulting mixture was stirred at that temperature for 5 min. Solvent was evaporated under a reduced pressure to afford the corresponding catalyst 3a-i as a colorless crystal in quantitative yield.
(S)-1,1-Diphenyl-1-(trimethylsilyloxy)propan-2-amine・CF3CO2H (3a)
Colorless oil. [α]D28 73.33 (c 0.30, CHCl3); IR (KBr) cm-1: 701, 838, 1130, 1254, 1541, 1684; 1H-NMR (CDCl3) δ: 7.51-7.26 (m, 10H, Ph), 4.37 (d, J = 6.9 Hz, 1H, NCH), 1.24 (d, J = 6.9 Hz, 3H, CH3), -0.18 (s, 9H, Si(CH3)3); 13C-NMR (CDCl3) δ:129.0, 128.6, 128.0, 81.9, 54.9, 14.9, 1.4; Anal. Calcd for C20H26F3NO3Si: C, 58.09; H, 6.34; N, 3.39. Found: C, 57.91; H, 6.48; N, 3.22.
(S)-3-Methyl-1,1-diphenyl-1-(trimethylsilyloxy)butan-2-amine・CF3CO2H (3b)
White crystal (Et2O). Mp 220-221 °C; [α]D27 113.33 (c 0.30, CHCl3); IR (KBr) cm-1: 702, 833, 1051, 1253, 1510, 1667; 1H-NMR (CDCl3) δ: 7.77-7.26 (m, 10H, Ph), 4.10 (d, J = 3.2 Hz, 1H, NCH), 1.97 (s, 1H, CH(CH3)2), 1.05 (d, J = 6.9Hz, 6H, CH(CH3)2), 0.45 (d, J = 6.9 Hz, 6H, CH(CH3)2), -0.20 (s, 9H, Si(CH3)3); 13C-NMR (CDCl3) δ:129.2, 128.9, 128.5, 82.1, 63.4, 26.6, 22.6, 17.0, 1.4; Anal. Calcd for C22H30 F3NO3Si: C, 59.84; H, 6.85; N, 3.17. Found: C, 59.73; H, 6.98; N, 3.05.
(S)-3,3-Dimethyl-1,1-diphenyl-1-(trimethylsilyloxy)butan-2-amine・CF3CO2H (3c)
White crystal (Et2O). Mp 222-223 °C; [α]D24 83.33 (c 0.30, CHCl3); IR (KBr) cm-1: 702, 836, 1133, 1254, 1495, 1673, 2958; 1H-NMR (CDCl3) δ: 7.58-7.09 (m, 10H, Ph), 4.19 (s, 1H, NCH), 0.75 (s, 9H, C(CH3)3), -0.14 (s, 9H, Si(CH3)3); 13C-NMR (CDCl3) δ:129.3, 128.9, 128.7, 128.5, 128.0, 83.3, 65.2, 34.7, 28.1, 1.6; Anal. Calcd for C23H32F3NO3Si: C, 60.64; H, 7.08; N, 3.07. Found: C, 60.48; H, 7.20; N, 2.95.
(S)-1,2,2-Triphenyl-2-(trimethylsilyloxy)ethan-2-amine・CF3CO2H (3d)
White crystal (Et2O). Mp 189-190 °C; [α]D27 43.33 (c 0.30, CHCl3); IR (KBr) cm-1: 697, 837, 1131, 1254, 1507, 1684; 1H-NMR (CDCl3) δ: 7.64-6.79 (m, 10H, Ph), 5.29 (s, 1H, NCH), -0.16 (s, 9H, Si(CH3)3); 13C-NMR (CDCl3) δ:129.5, 129.4, 129.2, 128.7, 128.4, 127.6, 127.5, 83.5, 62.7, 1.6; Anal. Calcd for C25H28 F3NO3Si: C, 63.14; H, 5.93; N, 2.95. Found: C, 62.98; H, 6.02; N, 2.82.
(S)-1,1,3-Triphenyl-1-(trimethylsilyloxy)propan-2-amine・CF3CO2H (3e)
White crystal (Et2O). Mp 173-174 °C; [α]D28 246.66 (c 0.30, CHCl3); IR (KBr) cm-1: 699, 839, 1131, 1254, 1507, 1684; 1H-NMR (CDCl3) δ: 7.66-7.08 (m, 10H, Ph), 4.45 (d, J = 10.9 Hz, 1H, NCH), 3.08 (d, J = 14.3 Hz, 2H, CH2Ph), 2.45 (t, J = 14.0 Hz, 2H, CH2Ph), -0.10 (s, 9H, Si(CH3)3); 13C-NMR (CDCl3) δ:129.6, 129.2, 129.0, 128.7, 128.2, 127.1, 125.6, 125.0, 81.9, 60.6, 35.1, 1.5; Anal. Calcd for C26H30F3NO3Si: C, 63.78; H, 6.18; N, 2.86. Found: C, 63.65; H, 6.29; N, 2.75.
(S)-3,3-Dimethyl-1,1-diphenyl-1-(trimethylsilyloxy)butan-2-amine・CH3CO2H (3f)
White crystal (Et2O). Mp 167-168 °C; [α]D28 29.99 (c 0.30, CHCl3); IR (KBr) cm-1: 695, 837, 1074, 1179, 1541, 1698; 1H-NMR (CDCl3) δ: 7.67-7.11 (m, 10H, Ph), 3.67 (s, 1H, NCH), 0.72 (s, 9H, C(CH3)3), -0.32 (s, 9H, Si(CH3)3); 13C-NMR (CDCl3) δ:129.0, 128.5, 128.2, 127.7, 127.3, 85.0, 64.9, 35.4, 28.8, 1.8; Anal. Calcd for C23H35NO3Si: C, 68.78; H, 8.78; N, 3.49. Found: C, 68.61; H, 8.92; N, 3.35.
(S)-3,3-Dimethyl-1,1-diphenyl-1-(trimethylsilyloxy)butan-2-amine・CCl3CO2H (3g)
White crystal (Et2O). Mp 177-178 °C; [α]D29 119.99 (c 0.30, CHCl3); IR (KBr) cm-1: 676, 827, 1079, 1173, 1507, 1684; 1H-NMR (CDCl3) δ: 7.65-7.14 (m, 10H, Ph), 4.30 (s, 1H, NCH), 0.78 (s, 9H, C(CH3)3), -0.12 (s, 9H, Si(CH3)3); 13C-NMR (CDCl3) δ:128.7, 128.1, 83.4, 65.3, 34.8, 28.2, 1.8; Anal. Calcd for C23H32Cl3NO3Si: C, 54.71; H, 6.39; N, 2.77. Found: C, 54.61; H, 6.47; N, 2.63.
(S)-3,3-Dimethyl-1,1-diphenyl-1-(triethylsilyloxy)butan-2-amine・CF3CO2H (3h)
Colorless oil. [α]D28 123.33 (c 0.30, CHCl3); IR (KBr) cm-1: 704, 832, 1133, 1243, 1507, 1684; 1H-NMR (CDCl3) δ: 7.51-7.26 (m, 10H, Ph), 4.23 (s, 1H, NCH), 0.77 (t, J = 7.7 Hz, 9H, Si(CH2CH3)3), 0.75 (s, 9H, C(CH3)3), 0.26 (dt, J =7.5 Hz, J = 7.7 Hz, 6H, Si(CH2CH3)3); 13C-NMR (CDCl3) δ:128.9, 128.7, 128.0, 127.7, 127.0, 126.8, 85.0, 65.5, 35.5, 28.8, 7.3, 6.0; Anal. Calcd for C26H38F3NO3Si: C, 62.75; H, 7.70; N, 2.81. Found: C, 62.63; H, 7.85; N, 2.71.
(S)-3,3-Dimethyl-1,1-diphenyl-1-(triisopropylsilyloxy)butan-2-amine・CF3CO2H (3i)
Colorless oil. [α]D28 143.33 (c 0.30, CHCl3); IR (KBr) cm-1: 706, 825, 1136, 1247, 1507, 1684; 1H-NMR (CDCl3) δ: 7.65-7.15 (m, 10H, Ph), 4.42 (s, 1H, NCH), 1.05 (s, 18H, Si(CH(CH3)2)3), 0.89 (dd, J = 8.9 Hz, J = 8.0 Hz, 18H, Si(CH(CH3)2)3), 0.73 (s, 9H, C(CH3)3), 0.60 (q, J = 7.5 Hz, 3H, Si(CH(CH3)2)3); 13C-NMR (CDCl3) δ:129.4, 129.1, 128.7, 128.1, 82.7, 65.5, 34.3, 28.1, 18.7, 17.7, 13.7, 12.2; Anal. Calcd for C29H44F3NO3Si: C, 64.53; H, 8.22; N, 2.60. Found: C, 64.41; H, 8.38; N, 2.49.
General procedure for the DA reaction of 1, 2-dihydropyridine 5a,b with acrolein 6, 11 using catalyst 3c.
To a CH3CN:H2O (19:9, 0.5 mL) solution of catalyst 3c (0.01 mmol) and 1,2-dihydropyridines 5a,b (0.1 mmol), distillated acroleins 6, 11 (0.3 mmol) was added at 0 °C and the solution was stirred at 0 °C for 24 h. The reaction was quenched by water. The reaction mixture was diluted with water and extracted with Et2O. The combined organic extracts were washed with brine, dried over Na2SO4, and removed under reduced pressure to give crude DA adducts 7a, 9a, 12, 13 which was used to the next reaction without purification.
To a stirred solution of products 7a, 9a, 12, 13 in EtOH (2.0 mL), NaBH4 (2.0 mg, 0.05 mmol) was added and the mixture was stirred at 0 °C for 1 h. Solvent was evaporated under a reduced pressure. The reaction mixture was diluted with water and extracted with AcOEt. The combined organic extracts were washed with brine, dried over Na2SO4, removed under reduced pressure to give a crude DA adducts. The residue was purified by preparative TLC (SiO2, n-hexane : AcOEt = 1 : 1) to afford the DA adducts 8a, 10a, 14, 15 in quantitative yield.
References
1. (a) J. Seayad and B. List, Org. Biomol. Chem, 2005, 3, 719; CrossRef (b) P. I. Dalko and L. Moisan, Angew. Chem. Int. Ed., 2004, 43, 5138; CrossRef (c) W. Notz, F. Tanaka, and C. F. Barbas, III, Acc. Chem. Res., 2004, 37, 580; CrossRef (d) B. List, Synlett, 2001, 1675; CrossRef (d) L.-W. Xu, J. Luo, and Y. Lu, Chem. Commun., 2009, 1807; CrossRef (e) L.-W. Xu, L. Li, and Z.-H. Shi, Adv. Synth. Catal., 2010, 352, 243; CrossRef H. Pellissier, Tetrahedron, 2012, 68, 2197. CrossRef
2. (a) C. Suttibut, Y. Kohari, K. Igarashi, H. Nakano, M. Hirama, C. Seki, H. Matsuyama, K. Uwai, N. Takano, Y. Okuyama, K. Osone, M. Takeshita, and E. Kwon, Tetrahedron Lett., 2011, 52, 4745; CrossRef (b) H. Nakano, K. Osone, M. Takeshita, E. Kwon, C. Seki, H. Matsuyama, N. Takano, and Y. Kohari, Chem. Commun., 2010, 46, 4827. CrossRef
3. (a) N. Takenaka, Y. Huang, and V. H. Rawal, Tetrahedron, 2002, 58, 8299; CrossRef (b) H. Nakano, N. Tsugawa, and R. Fujita, Tetrahedron Lett., 2005, 46, 5677; CrossRef (c) H. Nakano, N. Tsugawa, K. Takahashi, Y. Okuyama, and R. Fujita, Tetrahedron, 2006, 62, 10879. CrossRef
4. (a) P. Popik and P. Skolnick, in Pharmacology of Ibogaine and Ibogaine-related Alkaloids. The Alkaloids. Chemistry and Biology, ed. by G. A. Cordell, Academic: San Diego, 1999, Vol. 52, 197; (b) S. D. Glick, I. M. Maisonneuve, and K. K. Szumlinski, in Mechanisms of Action of Ibogaine: Relevance to Putative Therapeutic Effects and Development of a Safer Iboga Alkaloid Congener. The Alkaloids, ed. by K. R. Alper, S. D. Glick, and G. A. Cordell, Academic: San Diego, 2001, Vol. 56, 39.
5. (a) G. Büchi, D. L. Coffen, K. Kocsis, P. E. Sonnet, and F. E. Ziegler, J. Am. Chem. Soc., 1966, 88, 3099; CrossRef (b)Stanley Raucher , Brian L. Bray, Ross F. Lawrence, J. Am. Chem. Soc., 1987, 109, 442; CrossRef (c) M. T. Reding and T. Fukuyama, Org. Lett., 1999, 1, 973. CrossRef
6. D. Y. He, N. N. McGough, A. Ravindranathan, J. Jeanblanc, M. L. Logrip, K. Phamluong, P. H. Janak, and D. Pon, J. Neurosci., 2005, 25, 619. CrossRef
7. N. Satoh, T. Akiba, S. Yokoshima, and T. Fukuyama, Tetrahedron, 2009, 65, 3239. CrossRef
8. Y. Hayashi, T. Itoh, and H. Ishikawa, Angew. Chem. Int. Ed., 2011, 50, 3920. CrossRef
9. (a) K. Ishihara and K. Nakano, J. Am. Chem. Soc., 2005, 127, 10504; CrossRef (b) A. Sakakura, K. Suzuki, K Nakano, and K. Ishihara, Org. Lett., 2006, 8, 2229; CrossRef (c) K. Ishihara, K. Nakano, and M. Akakura, Org. Lett., 2008, 10, 2893; CrossRef (d) A. Sakakura, H. Yamada, and K. Ishihara, Org. Lett., 2012, 14, 2972. CrossRef