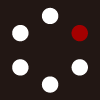
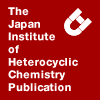
HETEROCYCLES
An International Journal for Reviews and Communications in Heterocyclic ChemistryWeb Edition ISSN: 1881-0942
Published online by The Japan Institute of Heterocyclic Chemistry
e-Journal
Full Text HTML
Received, 20th June, 2012, Accepted, 27th July, 2012, Published online, 7th August, 2012.
DOI: 10.3987/COM-12-S(N)46
■ STEREOSELECTIVE SYNTHESIS OF THE FUSED γ-LACTONE/δ-LACTONE CORE OF OPHIODILACTONES
Takaaki Matsubara, Keisuke Takahashi, Jun Ishihara, and Susumi Hatakeyama*
Graduate School of Biomedical Sciences, Nagasaki University, 1-14, Bunkyo-machi, Nagasaki 852-8521, Japan
Abstract
A promising precursor of ophiodilactones A and B, tetrameric phenyl propanoids isolated form the ophiuroid Ophiocoma scolopendrina, has been synthesized stereoselectively employing a halolactonization and an intramolecular epoxide-opening with a carboxylic acid as key reactions.Ophiodilactones A (1) and B (2), isolated form the ophiuroid Ophiocoma scolopendrina, exhibit moderate cytotoxic activity against P388 murine leukemia cells with IC50 values of 5.0 and 2.2 µg/mL, respectively.1 These compounds possess characteristic structures consisting of a fused γ-lactone/δ-lactone skeleton with four phenyl groups and four or five contiguous stereogenic centers containing three quaternary centers. The absolute configuration of 1 was tentatively determined by its CD spectrum; however that of 2 has not been elucidated yet.1 Their unique highly substituted dilactone structures and intriguing biological activities prompted us to investigate the synthesis of ophiodiactones. We report here the highly stereoselective synthesis of the fused γ-lactone/δ-lactone core 3, a promising precursor of ophiodilactones.
Since ophiodilactone B (2) could be accessible from ophiodilactone A (1) by, for example, Oikawa’s method involving α-sulfinylation, Pummerer reaction accompanied by cyclization of a phenyl group, and desulfurization,2 we focused on the synthesis of 1. Scheme 1 illustrates our retrosynthetic analysis of 1.
We envisaged dilactone 3 as a precursor of 1, which could be accessed from 4 by intramolecular epoxide-opening with a carboxylic acid group. To access 4 we envisioned an approach starting with halolactonization of 6 via Z-selective Horner-Wadsworth-Emmons olefination of aldehyde 5. The key issue of this approach is the diastereoselectivity of the halolactonization step as well as the feasibility of the δ-lactone formation.
Our synthesis of the key dilactone 3 thus commenced with the enantio- and stereoselective preparation of epoxy carboxylic acid 6 (Scheme 2). Meldrum’s acid was converted to β-ketoester 73 in 81% yield by acylation with phenylacetyl chloride followed by methanolysis and benzylation. Upon treatment of 7 with triflic anhydride under alkaline conditions according to Frantz’s method,4 the enol triflation took place with complete Z-selectivity5 to afford triflate 8 as the sole product in 93% yield. Stille coupling6 of 8 with stannane 9,7-9 and subsequent DIBAH reduction gave alcohol 10 in 87% yield. Katsuki-Sharpless asymmetric epoxidation10 of 10 afforded epoxy alcohol 11 in 83% yield but the enantioselectivity was disappointingly low.11 Compound 11 thus obtained was then subjected to Dess-Martin oxidation and Lindgren-Kraus oxidation to provide carboxylic acid 6 which was used for the next reaction without purification.
The crucial iodolactonization of 6 was conducted under the conditions using iodine and aqueous NaHCO3 in CH2Cl2 at room temperature to give a diastereoisomeric mixture of 12 and 13, which was directly hydrolyzed to afford epoxy γ-lactones 14 and 15 as a 6:1 mixture in 84% yield from 11 (Scheme 3). The relative configurations of 14 and 15 are determined by their NOESY and HMBC spectra. The observed diastereoselectivity can be rationalized by considering intermediates 12’ and 13’. Thus, intermediate 13’ experiences a severe steric repulsion between the C-2 and C-3 benzyl groups. On the other hand, another intermediate 12’ does not undergo such a significant steric repulsion, so that 12’ becomes thermodynamically more stable than 13’. Since intermediates 12’ and 13’ exist under equilibration, compound 12 is produced as a major product.
With the desired epoxy γ-lactone 14 in hand, we next investigated the construction of the fused γ-lactone/δ-lactone core structure (Scheme 4). Swern oxidation of 14 gave aldehyde 5, which was then subjected to Horner-Wadsworth-Emmons reaction following Ando’s protocol12 to afford Z-α,β-unsaturated ester 16 quantitatively. Then, we examined the key δ-lactone formation under various conditions (Table 1). As a result, when 16 was heated at 80 °C in TFA using a sealed tube, the cleavage of the tert-butyl ester and the concomitant epoxide-opening took place cleanly to give dilactone 313 having a fused γ-lactone/δ-lactone skeleton in 94% yield (entry 1). This TFA-promoted reaction turned out to be very sluggish at refluxing temperature (entry 2). Among Lewis acids searched, ZnBr2 was found to effectively promote the cyclization (entries 3, 4, and 5) and dilactone 3 was obtained in 83% yield under the conditions listed in entry 5. The stereochemistry of 3 was confirmed by its NOESY spectra.
In conclusion, we have developed an effective method for the stereoselecive construction of the fused γ-lactone/δ-lactone core structure of ophiodilactones. The remaining task towards the total synthesis of ophiodilactone A (1) is the stereoselective introduction of a phenyl group to 3 which is currently under investigation.
ACKNOWLEDGEMENTS
This work was supported by the Grant-in-Aid for Scientific Research (A) (22249001) from JSPS and the Grant-in-Aid for Scientific Research on Innovative Areas “Advanced Molecular Transformations by Organocatalysis” (No. 2304) (24105526) from MEXT.
References
1. S. Matsunaga, R. Ueoka, and T. Fujita, J. Org. Chem., 2009, 74, 4396. CrossRef
2. Y. Oikawa and O. Yonemitsu, J. Org. Chem., 1976, 41, 1118. CrossRef
3. O. Yonemitsu, Y. Oikawa, and K. Sugano, Org. Synth., Coll. Vol. II, 1990, 359.
4. D. E. Frantz, D. Babinski, and O. Soltani, Org. Lett., 2008, 10, 2901. CrossRef
5. Triflation of 7 using Tf2O and aqueous 25 % Me4NOH in hexane at 5 °C gave the corresponding E-isomer exclusively in 93% yield. See ref 4.
6. E. J. Corey, X. Han, and B. M. Stoltz, J. Am. Chem. Soc., 1999, 121, 7600. CrossRef
7. J. B. Lambert, E. C. Chelius, W. J. Schulz, Jr., and N. E. Carpenter, J. Am. Chem. Soc., 1990, 112, 3156. CrossRef
8. M. S. Baird, A. V. Nizovtsev, and I. G. Bolesov, Tetrahedron, 2002, 58, 1581. CrossRef
9. Y. Yamamoto, V. Gevorgyan, and J.-X. Liu, J. Org. Chem., 1997, 62, 2963. CrossRef
10. Y. Gao, R. M. Hanson, J. M. Klunder, S. Y. Ko, H. Masamune, and K. B. Sharpless, J. Am. Chem. Soc., 1987, 109, 5765. CrossRef
11. The enantiomeric purity was determined by 1H NMR analysis of the corresponding R- and S-MTPA esters although the absolute configuration was not determined.
12. K. Ando, J. Org. Chem., 1999, 64, 8406. CrossRef
13. Dilactone 3: a yellow oil. 1H NMR (400 MHz, CDCl3) δ 7.44-7.24 (m, 15H), 6.56 (d, J = 10.1 Hz, 1H), 5.91 (d, J = 10.1 Hz, 1H), 4.07 (d, J = 14.2 Hz, 1H), 3.27 (d, J = 14.6 Hz, 1H), 3.24 (d, J = 14.7 Hz, 1H), 3.19 (d, J = 14.6 Hz, 1H), 3.05 (d, J = 14.2 Hz, 1H), 2.95 (d, J = 14.7 Hz, 1H), 2.90 (s, 1H); 13C NMR (100 MHz, CDCl3) δ 174.0, 160.0, 139.5, 132.9, 132.8, 132.5, 132.1, 131.4, 131.3, 128.7, 128.6, 127.9, 127.7, 127.6, 123.1, 91.0, 78.4, 77.1, 41.2, 37.7, 35.3, 29.7, 18.4; FTIR (neat) 3420, 3031, 1783, 1741, 1495, 1452, 1279, 1181, 1087, 1038 cm-1; MS (EI) m/z 91 (100), 185, 276, 305, 440 (M+); HRMS (EI) calcd for C28H24O5 (M+) 440.1622, found 440.1625.