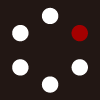
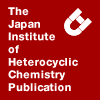
HETEROCYCLES
An International Journal for Reviews and Communications in Heterocyclic ChemistryWeb Edition ISSN: 1881-0942
Published online by The Japan Institute of Heterocyclic Chemistry
e-Journal
Full Text HTML
Received, 9th July, 2012, Accepted, 31st July, 2012, Published online, 14th August, 2012.
DOI: 10.3987/COM-12-12543
■ Synthesis, Reactions, and Rearrangements of 2,4-Diazabicyclo[3.1.0]hexan-3-ones
Helmut Quast,* Josef Walter Stawitz, and Bodo Mueller
Institute of Organic Chemistry, University of Würzburg, Am Hubland, D-97074 Würzburg, Germany
Abstract
Copper-mediated cyclopropanation of 1,3-dialkyl-1,3-dihydroimidazol-2-ones with diazomethane and methyl diazoacetate yields 2,4-diazabicyclo[3.1.0]hexan-3-ones and the first diaza[4.3.1]propellane. The bicyclic system survives elevated temperatures and in strongly basic and acidic solutions, but drastic conditions cleave the (C-1)–(C-6) bond affording 1,3-dihydroimidazol-2-ones. Catalytic hydrogenation removes N-benzyl groups and opens the (C-1)–(C-6) bond. Lithium aluminium hydride reduces 2,4-dibenzyl-2,4-diazabicyclo[3.1.0]hexan-3-one to a bicyclic aminal. Acid-induced cleavage of its imidazolidine ring is followed by unexpected transformations that probably procede via an acyclic intermediate, which comprises an azomethine ylide and an iminium moiety. 1,3-Cyclization involving both functional groups leads to N,N’-dibenzyl-trans-1,2-cyclopropanediamine, while 1,5-cyclization gives rise to the formation of N-benzylpyrrol along with N-benzylamine.INTRODUCTION
Since the Sixties, an increasing number of heterobicyclo[3.1.0]hexanes have been synthesized by [2+1] cycloaddition reactions of carbenes and carbenoids at CC double bonds that are part of five-membered heterocycles.1–6 In search of a novel route to cis-1,2-cyclopropanediamines, we employed the [2+1] cycloaddition strategy and synthesized the first 2,4-diazabicyclo[3.1.0]hexan-3-ones by copper-mediated cyclopropanation of 1,3-dihydroimidazol-2-ones with diazo compounds.7 Thereafter, several examples appeared in the literature: 2,4-Diazabicyclo[3.1.0]hexan-3-ones 1 were obtained as byproducts of the Curtius degradation of cis-1,2-cyclopropanedicarboxylic acids.8,9 Cyclopropanation of 1,3-diacetyl-1,3-dihydroimidazol-2-ones, either copper-catalyzed with diazomethane10 or by Simmons-Smith reaction,11 furnished low yields of 2a and 2b, respectively. The latter was converted into 2c and 2d.11 Recently, Schaumann and coworkers reported formation and X-ray diffraction analysis of the annelated 2,4-diazabicyclo-[3.1.0]hexan-3-one 3.12 Apart from being attractive in their own right, 2,4-diazabicyclo[3.1.0]hexan-3-ones promise to show interesting pharmacological properties as they are homologues of 1,3-dihydroimidazol-2-ones, which exhibit broad biological activities.13 Therefore, it comes as no surprise that the 2,4-diazabicyclo[3.1.0]hexane system emerged as integral part of bioactive molecules in the medicinal chemistry literature.14
Here we give a full account of our earlier7 and subsequent experiments with the view of (i) exploring scope and limitations of the copper-mediated cyclopropanation of 1,3-dihydroimidazol-2-ones with diazo compounds and (ii) probing stability and some aspects of the reactivity of 2,4-diazabicyclo[3.1.0]hexanones.
RESULTS AND DISCUSSION
Cyclopropanation of cis-1,2-ethenediamines whose configuration is fixed by a ring may be imagined askey step of a novel access to cis-1,2-cyclopropanediamines.7 In principle, this strategy for the synthesis of cis-1,2-substituted cyclopropanes is not new: For example, Maier and Sayrac employed it as early as 1966 to synthesize cis-1,2-cyclopropanedicarboxaldehyde.2 In pursuit of our synthetic objective, we conceived 1,3-dihydroimidazol-2-ones (4, 6) as equivalents of cis-1,2-ethenediamines in the projected [2+1] cycloaddition reaction, which thus would lead to 2,4-diazabicylo[3.1.0]hexan-3-ones.7 N-Benzyl compounds 4 were preferred over the corresponding N-methyl analogs, because these tend to be hygroscopic.15 More important, N-benzyl substituents provide a convenient criterion for the distinction between planar starting materials and 2,4-diazabicylo[3.1.0]hexanes: N-Benzyl protons of the former are enantiotopic (i. e., they appear equivalent in normal 1H NMR spectra), whereas those of the latter are diastereotopic.
While 1,3-dihydroimidazol-2-ones undergo sensitized [2+2] and [4+2] photocycloaddition reactions,16 they are reluctant toward thermal cycloaddition. Therefore, we tried in exploratory experiments one of the most efficient cyclopropanation procedures, viz. the Gaspar-Roth method,17 which involves passing gaseous diazomethane carried in a stream of dry nitrogen18 into a suspension of cuprous chloride in the liquid, olefinic substrate or concentrated solutions thereof. Even benzene is attacked by the intermediate in the copper-mediated decomposition of diazomethane.17 Likewise, the Gaspar-Roth method cyclopropanated N-carbomethoxypyrrol to afford the first example of the 2-azabicyclo[3.1.0]hexane ring system.3
It was gratifying to observe a single product (5a) when the Gaspar-Roth protocol was applied to 4a. Unfortunately, numerous attempts to achieve more than ca. 15 – 20 % conversion in this way met with failure. The conversion increased to 50 %, however, when a very large excess of diazomethane in diethyl ether19 was allowed to distill into concentrated solutions of 4a in the presence of cuprous chloride (under nitrogen and strict exclusion of moisture). The mixture turned dark due to formation of colloidal copper(0)20 and was worked-up with the view to avoid the deleterious effect of further dilution and to renew the catalyst. The resulting oil was again subjected to the cyclopropanation procedure to improve the ratio 4a:5a to 18:82. Chromatography on silicagel readily separated 4a and 5a, which could be purified further by short-path distillation under high vacuum. A yield of almost 80% was realized in this way.
Already a single treatment of 4,5-dimethylimidazolone 4b with diazomethane/CuCl furnished a reasonable conversion to 5b, whereas this procedure had to be repeated several times to achieve an acceptable conversion of the annelated imidazolone 6 to diaza[4.3.1]propellane 7 (Scheme 1).21
A number of unsaturated five-membered rings have been cyclopropanated by methyl and ethyl diazoacetate in the presence of copper bronze, copper salts, and copper complexes. The 6-alkoxycarbonyl groups of the resulting (hetero)bicyclo[3.1.0]hexane derivatives adopted preferentially the exo position, corresponding to a trans-selective [2+1] cycloaddition.1,3–5,22
Mixtures of 4a and methyl diazoacetate were heated in boiling diethyl ether in the presence of copper bronze, the oldest copper catalyst23 (Scheme 2). Two isomeric products arose in almost quantitative combined yield, along with some dimethyl fumarate and maleate. An excess of copper bronze (1.4 mol/mol 4a) was required for a smooth reaction since smaller amounts (0.9 mol/mol 4a) resulted in recovery of substantial fractions of starting material, even after extended periods of time. The crystalline major product 8 was isolated conveniently in good yield, while the minor, oily product had to be separated from small amounts of the former by chromatography. Analytical and spectroscopic evidence suggested structure 9 for the minor product, which corresponds to an insertion of methoxycarbonylcarbene into a ring C–H bond of the starting material 4a. Copper-mediated insertion of methoxycarbonylcarbene into sp2 C–H bonds has been observed.6 However, 9 is also formed by rearrangement of the major product 8 at elevated temperatures (see below). Consequently, speculation as to the way of formation of 9 is not warranted in the absence of experimental evidence.
Ring-methylated imidazolone 4b reacted slower than 4a. Therefore, a higher excess of both methyl diazoacetate and copper bronze and THF instead of diethyl ether were employed. The product consisted of at least five components. Chromatography allowed to isolate a mixture of the isomers 10 and 11. High-vacuum distillation of this fraction gave a mixture of 10 and 11 (2:3) and left 11 as pure oil. Apparently, 10 is thermally labile and rearranges in a homo-[1.5]H shift to 11. This rearrangement is well-kown for alkyl cis-2-methylcyclopropanecarboxylates.24 Structure 11 of the major product is suggested by NMR spectroscopic comparison with the similar compound 12, which arises besides 4b by benzylation of 4,5-dimethyl-1,3-dihydroimidazol-2-one (see Supporting Information). The 1H NMR spectra of both compounds exhibit the expected doublet pattern for the diastereotopic protons of methylene groups at C-4 and C-5. Other 1,3,5,5-tetraalkyl-4-methyleneimidazolidin-2-ones of type 12 are known for long.25
A number of attempts to employ dimethyl diazomalonate in the copper-catalyzed cyclopropanation of 4a met with failure. Heating of solutions of 4a and dimethyl diazomalonate in the presence of copper bronze gave complex mixtures. None of the fractions separated by chromatography showed the typical AB pattern of diastereotopic N-benzyl protons in the NMR spectrum.
The 2,4-diazabicyclo[3.1.0]hexan-3-one structures 5, 7, 8, and 10 of the cyclopropanation products were immediately evident from NMR spectra: These indicate the presence of a symmetry plane passing through C-3 and C-6 and bisecting the bond between the bridgehead carbon atoms; protons of N-benzyl groups are diastereotopic due to the presence of centres of chirality (C-1 and C-5); and signals of cyclopropane protons appear at high field. They were assigned for 5a to 6-Hexo and 6-Hendo on the basis of the well-established rule for vicinal coupling between cyclopropane protons, viz., 3Jtrans< 3Jcis.26,27 The 6-methoxycarbonyl group of 8 was attributed the exo configuration as suggested by the small size of the vicinal coupling constant 3J1-H,6-H = 1.8 Hz = 3Jtrans.
General chemical properties and stability of 2,4-diazabicyclo[3.1.0]hexan-3-ones have not been reported so far. An exploratory study of these aspects employed those of the present work. Reaction conditions and results are summarized in Scheme 3.
The 2,4-diazabicyclo[3.1.0]hexan-3-ones 5a and 5b can be recovered unchanged after dry heating at 150 °C or heating for several hours in boiling acetic acid. Under these conditions, 8 opens the (C-1)–(C-6) bond to afford 1,3-dihydroimidazol-2-one 9. In strongly basic media, 5a and 8 differ in the same way: While 5a survives unchanged in an ethanolic solution of 10% potassium hydroxide at elevated temperatures, 8 undergoes (C-1)–(C-6) ring opening with concomitant hydrolysis of the ester group under similar conditions (→16). However, the bicyclic system remains intact and only hydrolysis is observed when 8 is heated in aqueous sodium carbonate (→13). Decarboxylation and (C-1)–(C-6) ring cleavage occur on heating of the acid 13 at 150 °C to yield 15. The same compound is obtained on brief heating of both 5a and 8 in dilute aqueous sulfuric acid. Catalysis by both strong acid and strong base of these rearrangements indicate operation of polar mechanisms.
As expected, catalytic hydrogenation removes the N-benzyl groups of 8 and selectively cleaves one of the cyclopropane bonds adjacent to the methoxycarbonyl group to furnish 14.
The results of the preceding section suggest that selective cleavage of the five-membered ring of 2,4-diazabicyclo[3.1.0]hexan-3-ones is not feasible. In pursuit of our original objective of developing a novel route to cis-1,2-cyclopropanediamines, we reduced of 5a using lithium aluminium hydride.28 An almost quantitative yield of the corresponding bicyclic aminal 17 was realized in this way. Aminal 17 can be distilled under high vacuum and is stable in methanol in the presence of sodium hydroxide but decomposes slowly, even under nitrogen, yielding red-brown polymeric products.
While N,N’-dibenzyltetrahydroimidazol was rapidly hydrolyzed by dilute hydrochloric acid to afford a quantitative yield of N,N’-dibenzyl-1,2-ethanediamine dihydrochloride,7 17 reacted in acidic media in puzzling ways. It dissolved in acetic acid with dark red color. An intense red color was also observed on addition of strong acids to solutions of 17 in ethanol. Work-up yielded resin-like products that lacked cyclopropane rings. Introduction of dry hydrogen chloride into a solution of 17 in dry diethyl ether gave a colorless precipitate, which decomposed above 68 °C and deliquesced on exposure to air. The 1H NMR spectrum recorded for a solution in trifluoracetic acid showed an ABX2 spectrum attributable to protons of a cis-1,2-disubstituted cyclopropane, which disappeared overnight, however.
Treatment of 17 with 0.1–1 M hydrochloric acid and separation of neutral, unknown products furnished, surprisingly, trans-19 rather than expected cis-19. The trans configuration of this product was evident from an AA’XX’ spin system, present according to the 1H NMR spectrum, and established by comparison with the authentic dihydrobromide.9 Experiments using higher concentrations of hydrochloric acid (10–35 %) afforded substantial amounts of colored decomposition products and, after basic work-up and distillation at 10-3 Torr, oily mixtures that exhibited ABX2 systems at high field, corresponding to cis-1,2-substituted cyclopropanes. Weakly acidic media such as 2 M aqueous acetic acid or a two-phase system consisting of chloroform and a saturated aqueous solution of copper(II) sulfate converted 17 into N-benzylpyrrole (22)29 and N-benzylammonium chloride (23). Signals of cyclopropane protons were not observed in these experiments. The appearance of acid-sensitive 22 would explain the deep red-brown colors developed by 17 in acidic media.
The results may be rationalized in terms of Scheme 4, which is based on extensive studies of the reactions of 1,2-cyclopropanediamines with aldehydes and ketones.30 Opening of the five-membered ring of protonated 17 leads to the iminium ion cis-18. In strongly acidic solution, this can be hydrolyzed in part to yield cis-19, because ring cleavage to azomethine ylide 20 is disfavored due to protonation of the amino group of cis-18. Lower concentrations of hydrochloric acid give rise to cis/trans isomerization cis-18→20→trans-18 followed by hydrolysis of the latter (→trans-19). Weakly acidic media favor ring-expansion cis-18→20→21, which finally leads to 22. Azomethine ylide 20 is invoked as common intermediate that may explain the cis/trans rearrangement to trans-1,2-cyclopropanediamine trans-19 as well as the cyclization to N-benzylpyrrol (22). Clearly, these unexpected transformations prevented realization of our original objective. However, they provided the impetus for productive studies of 1,2-cyclopropanediamines.30
The present work delineates a straightforward route to and some reactions of 2,4-diazabicyclo[3.1.0]hexan-3-ones, as yet a somewhat neglected heterobicyclic system. It is quite robust under a variety of conditions due to the inherent urea partial structure. Owing to the stability, 2,4-diazabicyclo[3.1.0]hexan-3-ones may replace cyclic urea moieties in many instances and thus lead to novel systems and properties. Reduction yields an aminal (2,4-diazabicyclo[3.1.0]hexane). This undergoes surprising reactions, probably involving an acyclic intermediate, which comprises an azomethine ylide and an iminium moiety. The results of this study may be expected to foster investigations of heterobicyclo[3.1.0]hexanes with the view of advanced applications. Extensions could include cyclopropanation of differently substituted 1,3-dihydroimidazol-2-ones and heteroanalogs thereof using modern transition metal catalysts.
EXPERIMENTAL
General Experimental and preparation of the 1,3-dialkyl-1,3-dihydroimidazol-2-ones 4a, 4b, and 6 used as starting materials are detailed in the Supporting Information. 1H1H coupling constants given are absolute values.
Copper-mediated cyclopropanation of 1,3-dihydroimidazol-2-ones with diazomethane, General Procedure. Diazomethane was generated from N-nitroso-N-methylurea according to the method of Arndt.19 Caution! N-nitroso-N-methylurea is suspected of being a carcinogen and diazomethane is highly toxic and explosive. It must be handled in an efficient fume hood behind a protection shield. In a brand-new 1L-Erlenmeyer flask (not etched or scratched), a solution of diazomethane was prepared from N-nitroso-N-methylurea (51.5 g, 0.50 mol), aq. KOH (40%, 175 mL) and Et2O (0.3–0.5 L), cooled at 5 °C. The ethereal solution was decanted, carefully dried with KOH pellets, and distilled under N2. To avoid ground-glass joints, we used a distillation apparatus made in a single piece from a 1L-flask, attached to a N2 supply, and a Liebig condensor, which was cooled at –30 °C. The adapter at the lower end of the condensor carried a fire-polished glass tube. The tube passed through a two-holed rubber stopper and reached almost the surface of a stirred, cooled (–15 to –10 °C) suspension in a 0.5-L-Erlenmeyer flask protected by a drying tube. The suspension consisted of CuCl (dried over P2O5), the 1,3-dihydroimidazol-2-one and dry Et2O (4a, 7) or dry CH2Cl2 (4b). Distillation was interrupted as soon as the distillate became colorless. The dark suspension was filtered (Celite) and the solvent distilled i. vac. to afford a pale yellow oily residue. The ratio 2,4-diazabicyclo[3.1.0]hexan-3-one vs. 1,3-dihydroimidazol-2-one was determined by 1H NMR. Treatment with diazomethane/CuCl was repeated with the residue until a satisfactory ratio was obtained. Starting material and product were separated by preparative layer or flash chromatography on silicagel with Et2O/hexane. The cyclopropanation products were collected in the first fractions. Further purification by recrystallization (5b) or short-path distillation in a sublimation apparatus (cold finger kept at –30 °C) under high vacuum (5a, 7) afforded the pure cyclopropanation products (TLC, 1H NMR).
2,4-Dibenzyl-2,4-diazabicyclo[3.1.0]hexan-3-one (5a): The first treatment of 4a (7.92 g, 30 mmol, solution in Et2O, 50 mL) with diazomethane in the presence of CuCl (5.0 g, 50 mmol) resulted in a pale yellow oil (8.67 g) with a ratio 4a:5a = 52:48, the second gave a pale yellow oil (9.41 g) with a ratio 4a:5a = 18:82. Chromatography followed by distillation at 70–90 °C bath temp./10-5 Torr afforded a pale yellow oil (6.80 g, 79%). IR (neat oil) ν [cm-1] = 1690 (s). MS (EI, 70 eV) m/z = 278 (M+). 1H NMR (600.1 MHz, CDCl3): δ [ppm] = –0.130 (dt, 2J = 6.3 Hz, 3Jtrans = 2.7 Hz, 1 H, 6-Hendo), 0.296 (dt, 2J = 6.3 Hz, 3Jcis = 5.6 Hz, 1 H, 6-Hexo), 2.837 (dd, 3Jcis = 5.6 H, 3Jtrans = 2.7 Hz, 2 H, 1-H, 5-H), 4.265 (d, 2J = 14.8 Hz, 1 H, ½ CH2), 4.517 (d, 2J = 14.8 Hz, 1 H, ½ CH2), 7.17–7.27 (m, 10 H, 2 Ph). IR (neat oil) ν [cm-1] = 1705 (s). MS (EI, 70 eV) m/z = 180 (M+). 13C NMR (150.9 MHz, CDCl3): δ [ppm] = 9.74 (CH2), 29.29 (CH), 47.82 (CH2), 127.21 (p-CH), 128.04, 128.33 (o, m-CH), 137.15 (ipso-C), 158.92 (C=O). Anal. Calcd for C18H18N2O: C, 77.67; H, 6.52. Found: C, 77.49; H, 6.86.
2,4-Dibenzyl-1,5-dimethyl-2,4-diazabicyclo[3.1.0]hexan-3-one (5b): According to the General Procedure, diazomethane was distilled into a mixture of 4b (8.77 g, 30 mmol), CuCl (5.0 g, 50 mmol) and dry CH2Cl2 (35 mL). Filtration, distillation of the solvent i. vac., and treatment of the residue with cold pentane yielded colorless crystals (9.17 g), which consisted of 4b and 5b with a ratio 38:62 (TLC, 1H NMR). Chromatography and recrystallization from pentane yielded colorless crystals (3.35 g, 36 %), mp 88–90 °C. IR (KBr) ν [cm-1] = 1695 (s), 1680 (m). MS (EI, 70 eV) m/z = 306 (M+). 1H NMR (60 MHz, CDCl3): δ [ppm] = –0.13, 0.01 (AB spectrum, 2J = 6.4 Hz, 2 H, 6-Hendo, 6-Hexo), 1.24 (s, 6 H, 2 CH3), 4.35, 4.69 (AB spectrum, 2J = 15.4 Hz, 4 H, 2 CH2), 7.34 (mc, 10 H, 2 Ph). Anal. Calcd for C20H22N2O: C, 78.40; H, 7.24; N, 9.14. Found: C, 78.34; H, 7.21; N, 9.16.
7,9-Dimethyl-7,9-diazatricyclo[4.3.1.01,6]decan-8-one (7): According to the General Procedure, diazomethane was distilled into a mixture of 6 (15.0 g, 90 mmol), CuCl (5.0 g, 50 mmol) and Et2O (30 mL). The resulting pale yellow oil was treated once again with diazomethane/CuCl. Short-path distillation of the oil at 50–75 °C bath temp./3×10-5 Torr yielded a colorless oil (14.0 g) consisting of polymethylene (δH = 1.26 ppm), 6 and 7 (45:55, 1H NMR). Two further treatments of the oil with diazomethane/CuCl and short-path distillation of the product at 50–75 °C bath temp./3×10-5 Torr gave a colorless oil (12.9 g, 6:7 = 27:73, 1H NMR). Flash chromatography on silicagel with CHCl3 and short-path distillation at 3×10-5 Torr yielded a colorless, hygroscopic oil (7.61 g, 47%). IR (neat oil) ν [cm-1] = 1705 (s). MS (EI, 70 eV) m/z = 180 (M+). 1H NMR (600.1 MHz, CDCl3): δ [ppm] = –0.020 (d, 2J = 5.9 Hz, 1 H), 0.195 (dd, 2J = 5.9 Hz, J = 0.8 Hz, 1 H), 0.97–1.05 (m, 2 H), 1.22–1.30 (m, 2 H), 1.65–1.77 (m, 4 H), 2.587 (dd, J = 1.7 Hz, J = 0.6 Hz, 6 H, 2 CH3). 13C NMR (150.9 MHz, CDCl3): δ [ppm] = 16.11 (CH2), 20.38 (2 CH2), 25.09 (2 CH2), 27.56 (2 CH3), 160.21 (C=O).
exo-2,4-Dibenzyl-6-carbomethoxy-2,4-diazabicyclo[3.1.0]hexan-3-one (8): A solution of methyl diazoacetate (2.20 g, 22 mmol) in dry Et2O (25 mL) was added dropwise during 30 min to a stirred, boiling mixture of 4a (2.64 g, 10 mmol), copper bronze (0.89 g, 14 mmol) and dry Et2O (25 mL). Heating under reflux was continued for 5 h followed by filtration and distillation of the solvent i. vac. Short-path distillation at rt/10-3 Torr removed decomposition products of methyl diazoacetate (dimethyl fumarate and maleate, 1H NMR). The solid, pale yellow residue was treated with cold Et2O (0 °C, 2 × 10 mL) and the insoluble material recrystallized from cyclohexane to afford colorless crystals (2.06 g, 62%) mp 105–106 °C. The solvent from the combined washings and mother liquor was distilled i. vac. and the residue separated by chromatography to yield a second crop of 8 (1st fraction, 0.52 g, 15%) mp 103–105 °C, and 9 as pale yellow oil (2nd fraction, 0.71 g, 20%), which was identical with the isomerization product of 8 (IR, 1H NMR, see below). 8: IR (KBr) ν [cm-1] = 1723 (s), 1688 (s). MS (EI, 70 eV) m/z = 336 (M+). 1H NMR (600.1 MHz, CDCl3): δ [ppm] = 1.010 (t, 3Jtrans = 1.8 Hz, 1 H, 6-Hendo), 3.477 (d, 3Jtrans = 1.8 Hz, 2 H, 1-H, 5-H), 3.574 (s, 3 H, OCH3), 4.324 (d, 2J = 14.8 Hz, 1 H, ½ CH2), 4.666 (d, 2J = 14.9 Hz, 1 H, ½ CH2), 7.28–7.32 (m, 6 H, Ph), 7.33–7.38 (m, 4 H, Ph). 13C NMR (150.9 MHz, CDCl3): δ [ppm] = 23.88 (CH-6), 39.08 (CH-1, CH-5), 47.77 (CH2), 51.81 (OCH3), 127.81 (p-CH), 128.16, 128.77 (o-, m-CH), 136.30 (ipso-C), 158.57 (C=O), 170.68 (CO2Me). Anal. Calcd for C20H20N2O3: C, 71.41; H, 5.99; N, 8.32. Found: C, 71.80; H, 6.02; N, 8.36.
1,3-Dibenzyl-4-carbomethoxymethyl-1,3-dihydroimidazol-2-one (9): (a) 8 (1.35 g, 4.0 mmol) was heated at 140–150 °C for 1 h to afford a pale yellow, very viscous oil (1.35 g, quant.). Attempts at crystallization met with failure. IR (neat oil) ν [cm-1] = 1735 (CO2Me), 1678 (C=O). MS (EI, 70 eV) m/z = 336 (M+). 1H NMR (60 MHz, CDCl3): δ [ppm] = 3.08 (s, 2 H, CH2), 3.47 (s, 3 H, OCH3), 4.69 (s, 2 H, CH2), 4.80 (s, 2 H, CH2), 6.00 (s, 1 H, 5-H), 7.13, 7.19 (mc, 10 H, 2 Ph). Anal. Calcd for C20H20N2O3: N, 8.32. Found: N, 8.42.
(b) A solution of 8 (1.35 g, 4.0 mmol) in acetic acid (15 mL) was heated at 80 °C for 3 h followed by evaporation of the solvent at rt/10-2 Torr to afford 9 (1.36 g, quant.) as a pale yellow viscous oil (TLC, IR, 1H NMR).
exo-2,4-Dibenzyl-6-carbomethoxy-2,4-diazabicyclo[3.1.0.]hexan-3-one (10) and 1,3-Dibenzyl-5- carbomethoxymethyl-5-methyl-4-methylene-1,3-dihydroimidazol-2-one (11): A solution of methyl diazoacetate (4.30 g, 43 mmol) in dry THF (30 mL) was added dropwise during 30 min to a stirred, boiling mixture of 4b (1.82 g, 6.2 mmol), copper bronze (2.0 g, 31 mmol) and dry THF (20 mL). Heating under reflux was continued for 6 h followed by filtration of the brown suspension and distillation of the solvent at 15 Torr and finally at 70 °C bath temp./10-2 Torr to afford a brown resin (2.96 g), which consisted of at least 5 components (TLC). Chromatography and drying of the main fraction for 1 h at 40–50 °C/10-5 Torr yielded a yellow oil (1.79 g). Short-path distillation at 100–120 °C bath temp./10-5 Torr furnished a colorless oil (0.69 g, 30%) consisting of 10 and 11 (2:3, TLC, 1H NMR). The residue was pure 11 as yellow oil (1.04 g, 46%), (TLC, 1H NMR).
10: 1H NMR (60 MHz, CDCl3): δ [ppm] = 0.80 (s, 1 H, 6-Hendo), 1.44 (s, 6 H, 2 CH3), 3.46 (s, 3 H, OCH3), 4.37, 4.61 (AB spectrum, 2J = 15.4 Hz, 4 H, 2 CH2), 7.29 (m, Ph).
10, 11 (2:3): Anal. Calc. for C22H24N2O: C, 72.51; H, 6.64; N, 7.69. Found: C, 72.38; H, 6.59; N, 7.65.
11: 1H NMR (60 MHz, CDCl3): δ [ppm] = 1.25 (s, 3 H, CH3), 2.47, 2.66 (AB spectrum, 2J = 16 Hz, 2 H, CH2), 3.39 (s, 3 H, OCH3), 3.85, 3.95 (AB spectrum, 2J = 2.8 Hz, 2 H, = CH2), 4.45, 4.62 (AB spectrum, 2J = 16 Hz, 2 H, CH2), 4.69 (s, 2 H, CH2), 7.29 (mc, 10 H, 2 Ph).
exo-2,4-Dibenzyl-6-carboxy-2,4-diazabicyclo[3.1.0]hexan-3-one (13): A suspension of 8 (1.35 g, 4.00 mmol) in aq. Na2CO3 (1 M, 25 mL) was heated under reflux for 4 h. Filtration and acidification of the filtrate with aq. HCl (2 M) yielded a preciptate, which was isolated by filtration and washed with water to yield colorless crystals (1.16 g, 90 %) mp 119–120 °C (dec.). IR (KBr) ν [cm-1] = 1710 (s, br.), 1670 (s, br.). 1H NMR (60 MHz, CDCl3): δ [ppm] = 0.88 (br., 1 H, 6-Hendo), 3.45 (d, 3Jtrans = 1.6 Hz, 1-H, 5-H), 4.37, 4.62 (AB spectrum, 2J = 15 Hz, 4 H, 2 CH2), 7.30 (mc, 10 H, 2 Ph), 9.74 (br., 1 H, CO2H). Anal. Calcd for C19H18N2O3: N, 8.69. Found: N, 8.62.
4-Carbomethoxymethyl-1,3-dihydroimidazol-2-one (14): A quantitative micro hydrogenation apparatus by NORMAG, O. Fritz, Hofheim am Taunus, Germany, was used. A vigorously stirred suspension of Pd/C (10%, 200 mg) in a solution of 8 (342 mg, 1.02 mmol) in EtOH (15 mL) was kept at 735 Torr H2 pressure and 54 °C. Consumption of H2 ceased after 24 h, when 85.3 mL of H2 (3.08 mmol) had been consumed. Filtration, washing of the catalyst with EtOH (50 mL), distillation of the solvent i. vac., and recrystallization of the residue from benzene yielded colorless crystals (121 mg, 75%), mp 108–109 °C. IR (KBr) ν [cm-1] = 1730 (s), 1695 (s). MS (EI, 70 eV) m/z = 158 (M+). 1H NMR (90 MHz, D2O, LAOCOON III simulation of the ABMX2 spectrum): δ [ppm] = 2.71 (d, X part of an ABMX2 spectrum, 3JMX = 6.4 Hz, 2 HX, 4-CH2), 3.25 (m, 2JAB = –9.3 Hz, 3JAM = 6.2 Hz, HA, 5-H), 3.72 (m, 2JAB = –9.3 Hz, 3JBM = 9.1 Hz, HB, 5-H), 4.21 (m, 3JAM = 6.2 Hz, 3JBM = 9.1 Hz, 3JMX = 6.4 Hz, HM, 4.H), 3.76 (s, 3 H, OCH3); 1H NMR in CDCl3: Ref.31 Anal. Calcd for C6H10N2O3: C, 45.56; H, 6.37. Found: C, 45.02; H, 6.36.
1,3-Dibenzyl-4-methyl-1,3-dihydroimidazol-2-one (15): (a) The acid 13 (645 mg, 2.00 mmol) was heated at 120–140 °C for 2 h. The product was dissolved in a small amount of Et2O. Filtration followed by cooling to –25 °C yielded pale yellow crystals (490 mg, 88 %), mp 68–70 °C.32 IR (KBr) ν [cm-1] = 1682 (s), 1673 (s). 1H NMR (60 MHz, CDCl3): δ [ppm] = 1.85 (d, 4J = 1.4 Hz, 3 H, CH3), 4.80 (s, 2 H, CH2), 4.87 (s, 2 H, CH2), 5.83 (q, 4J = 1.4 Hz, 1 H, 5-H), 7.25, 7.28 (mc, 10 H, 2 Ph). Anal. Calcd for C18H18N2O: N, 10.06. Found: N, 10.02.
(b) A stirred mixture of 8 (673 mg, 2.00 mmol), and aq. H2SO4 (1 M, 10 mL) was heated at 80 °C for 30 min followed by extraction with Et2O. The org. layer was dried with K2CO3, the solvent distilled i. vac., and the residue recrystallized from Et2O at –25 °C to yield pale yellow crystals (451 mg, 81%), mp 69–70 °C.
(c) A solution of 5a (279 mg, 1.00 mmol) in aq. H2SO4 (1 M, 5 mL) was heated at 90 °C for 1 h. The mixture was neutralized with aq. NaOH (2 M) and extracted with CH2Cl2. The org. layer was dried with K2CO3. Distillation of the solvent i. vac. gave a yellow-brown oil (237 mg, 85 %), which was a single compound (TLC) and identical with 15 (1H NMR).
1,3-Dibenzyl-4-carboxymethyl-1,3-dihydroimidazol-2-one (16): A stirred mixture of 8 (1.35 g, 4.00 mmol) and aq. KOH (20%, 20 mL) was heated at 70–80 °C for 30 min. The mixture was filtered and the filtrate neutralized with aq. conc. HCl to afford colorless crystals (1.10 g, 85 %), mp 147–149 °C (dec). 1H NMR (60 MHz, CDCl3): δ [ppm] = 3.17 (s, 2 H, CH2), 4.83 (s, 2 H, CH2), 4.92 (s, 2 H, CH2), 7.23, 7.27 (mc, 10 H, 2 Ph), 9.2 (br., 1 H, CO2H).
2,4-Dibenzyl-2,4-diazabicyclo[3.1.0]hexane (17): A solution of 5a (2.79 g, 10 mmol) in Et2O (10 mL) was added to a stirred solution of LiAlH4 (1.36 g, 35 mmol) in Et2O (100 mL). Stirring was continued for 2 d followed by decomposition of the excess of LiAlH4 with the calculated amount of ice-water, filtration, and distillation of the solvent i. vac. to afford a pale yellow liquid (2.64 g). Short-path distillation in a sublimation apparatus (cold finger at –30 °C) at 50–70 °C bath temp./10-5 Torr gave a colorless oil (2.49 g, 94 %). IR (neat oil) ν [cm-1] = 1600 (m), 1580 (w), 1490 (s). – MS (EI, 70 eV) m/z = 264 (M+). 1H NMR (60 MHz, CDCl3, LAOCOON III simulation of the ABX2 spectrum): δ [ppm] = –0.02 (dt, 2JAB = –6.8 Hz, 3Jcis = 6.2 Hz, 1 H, 6-Hexo), 1.25 (dt, 2JAB = –6.8 Hz, 3Jtrans = 3.0 Hz, 1 H, 6-Hendo), 2.67 (d, 2J = 5.6 Hz, 1 H, 3-H), 2.85 (dd, 3Jcis = 6.2 Hz, 3Jtrans = 3.0 Hz, 2 H, 1-H, 5-H), 3.72 (s, 4 H, 2 CH2), 3.87 (d, 2J = 5.6 Hz, 1 H, 3-H), 7.2–7.6 (m, 10 H, 2 Ph). Anal. Calcd for C18H20N2: C, 81.77; H, 7.62; N, 10.59. Found: C, 81.32; H, 7.63; N, 10.60.
N,N’-Dibenzyl-trans-1,2-cyclopropanediamine Dihydrochloride (trans-19): Under N2, a solution of 17 (2.64 g, 10 mmol) in Et2O (6 mL) was added to stirred aq. HCl (1 M, 50 mL). Stirring was continued for 2 h followed by extraction with CHCl3. Water was distilled i. vac. at 30–40 °C bath temp. from the colorless aq. layer. The solid residue was dried at 70 °C/0.001 Torr and triturated with a small amount of cold ethanol. The insoluble material was isolated by filtration and recrystallized from EtOH to afford pale pink crystals (0.80 g, 25%), mp 225–230 °C (dec.). The 1H NMR spectrum (D2O) was superimposable with the one recorded for the dihydrobromide.9 Anal. Calcd for C17H22Cl2N2: C, 62.77; H, 6.82; Cl, 21.80; N, 8.61. Found: C, 62.78; H, 7.01; Cl, 21.91; N, 8.77.
N-Benzylpyrrole (22): (a) A solution of 17 (132 mg, 0.50 mmol) in CHCl3 (10 mL) was vigorously stirred for 1 h in the presence of sat. aq. CuSO4 (5 mL). The org. layer was dried with K2CO3 followed by distillation of the solvent i. vac. to afford a pale brown oil (69 mg, 89%), which was pure and identical with an authentic sample (IR, 1H NMR) prepared by phase transfer catalysed N-benzylation of pyrrole.29
(b) A solution of 17 (132 mg, 0.50 mmol) in Et2O (10 mL) was stirred for 0.5 h in the presence of aq. acetic acid (2 M, 10 mL) followed by separation of the layers and extraction of the aq. layer with Et2O. Drying of the combined org. layers with K2CO3 and distillation of the solvent i. vac. yielded almost pure 22 as yellow oil (1H NMR). Aq. HCl (2 M, 20 mL) was added to the aq. layer followed by distillation of the solvent i. vac. and trituration of the residue with a small amount of EtOH to furnish N- benzylammonium chloride (23) as colorless powder (IR, 1H NMR); picrate (from ethanol): mp 193–195 °C; authentic sample: mp 194 °C.
ACKNOWLEDGEMENTS
We express our gratitude to Mrs. Elfriede Ruckdeschel and Dr. Matthias Gruene, University of Wuerzburg, for taking the high-field NMR spectra, and to Dr. Gerda Lange for recording the mass spectra. Financial Support by the Deutsche Forschungsgemeinschaft (DFG) and the Fonds der Chemischen Industrie, Frankfurt/Main, is gratefully acknowledged.
SUPPORTING INFORMATION AVAILABLE
General experimental, preparation of 1,3-dialkyl-1,3-dihydroimidazol-2-ones (4a, 4b, 6) (6 pages), and NMR spectra recorded for 5a, 7, 8, 14, and 17 (8 pages). This material is available free of charge via the Internet at http://www.heterocycles.com or from the author.
References
1. F. W. Breitbeil, III, J. J. McDonnell, T. A. Marolewski, and D. T. Dennerlein, Tetrahedron Lett., 1965, 6, 4627. CrossRef
2. G. Maier and T. Sayrac, Angew. Chem., Int. Ed. Engl., 1966, 5, 959. CrossRef
3. S. R. Tanny, J. Grossman, and F. W. Fowler, J. Am. Chem. Soc., 1972, 94, 6495. CrossRef
4. D. Seebach and G. Stucky, Angew. Chem. Int. Ed. Engl., 1988, 27. 1351; CrossRef D. Seebach, G. Stucky, and E. Pfammatter, Chem. Ber., 1989, 122, 2377; CrossRef M. L. Rosenberg, K. Vlašaná, N. S. Gupta, D. Wragg, and M. Tilset, J. Org. Chem., 2011, 76, 2465. CrossRef
5. Further examples may be found in reviews: H. E. Simmons, T. L. Cairns, S. A. Vladuchick, and C. M. Hoiness, Org. React., 1973, 20, 1; T. Ye and M. A. McKervey, ‘The Chemistry of the Cyclopropyl Group, Vol. 2: Metal Catalysed Cyclopropanations’, ed. by Z. Rappoport, Wiley, New York, 1995, pp. 657–706; G. R. Krow and K. C. Cannon, Org. Prep. Proced. Int., 2000, 32, 103; CrossRef A. Charette and A. Beauchemin, Org. React., 2001, 58, 1; H. M. L. Davies and E. G. Antoulinakis, Org. React., 2001, 57, 1; H. Lebel, J. F. Marcoux, C. Molinaro, and A. B. Charette, Chem. Rev., 2003, 103, 977; CrossRef M Fedoryński, Chem. Rev., 2003, 103, 1099; CrossRef H.-U. Reissig and R. Zimmer, Chem. Rev., 2003, 103, 1151; CrossRef F. Gnad and O. Reiser, Chem. Rev., 2003, 103, 1603; CrossRef and Ref.6.
6. H. M. L. Davies and S. J. Hedley, Chem. Soc. Rev., 2007, 36, 1109. CrossRef
7. Portions of this work have been published in preliminary form: H. Quast and J. W. Stawitz, Tetrahedron Lett., 1976, 17, 3803. CrossRef
8. D. T. Witiak, H. J. Lee, H. D. Goldman, and B. S. Zwilling, J. Med. Chem., 1978, 21, 1194. CrossRef
9. W. von der Saal, R. Reinhardt, H.-M. Seidenspinner, J. Stawitz, and H. Quast, Liebigs Ann. Chem., 1994, 569. CrossRef
10. K.-H. Scholz, W. Hartmann, and H.-G. Heine, Ger. Offen. (1978), DE 2713431 (A1) 1978-09-28.
11. J. L. Gagnon and W. W. Zajac, Jr., Synth. Commun., 1996, 26, 837. CrossRef
12. H. Nimmesgern, E. Schaumann, and G. Adiwidjaja, Heterocycles, 2012, 84, 945. CrossRef
13. See, for example: W. B. Wright, Jr., H. J. Brabander, and R. A. Hardy, Jr., J. Med. Chem., 1966, 9, 858; CrossRef R. A. Schnettler, R. C. Dage, and J. M. Grisar, J. Med. Chem., 1982, 25, 1477; CrossRef S. Cortes, Z. K. Liao, D. Watson, and H. Kohn, J. Med. Chem., 1985, 28, 601; CrossRef M. W. Moon, C. G. Chidester, R. F. Heier, J. K. Morris, R. J. Collins, R. R. Russell, J. W. Francis, G. P. Sage, V. H. Sethy, J. Med. Chem. 1991, 34, 2314; CrossRef E. M. Naylor, E. R. Parmee, V. J. Colandrea, L. Perkins, L. Brockunier, M. R. Candelore, M. A. Cascieri, L. F. Colwell, Jr., L. Deng, W. P. Feeney, M. J. Forrest, G. J. Hom, D. E. MacIntyre, C. D. Strader, L. Tota, P.-R. Wang, M. J. Wyvratt, M. H. Fisher, and A. E. Weber, Bioorg. Med. Chem. Lett., 1999, 9, 755; CrossRef H. S. Lozanova and G. N. Vassilev, Bulgar. Chem. Ind., 2004, 75, 24.
14. F. H. Jung, Eur. Pat. Appl. (1981), EP 0031708 (A2) 1981-07-08; F. Jung, C. Delvare, D. Boucherot, A. Hamon, N. Ackerley, and M. J. Betts, J. Med. Chem., 1991, 34, 1110; CrossRef X. Zhou, PCT Int. Appl. (2007), WO 2007085357 (A1) 2007-08-02; S. Chowdhury, N. Dales, J. Fonarev, J. Fu, D. Hou, Q. Jia, V. Kodumuru, N. Pokrovskaia, S. Sun, and Z. Zhang, PCT Int. Appl. (2009), WO 2009103739 (A1) 2009-08-27; C. J. Woolf and B. P. Bean, PCT Int. Appl. (2011), WO 2011006073 (A1) 2011-01-13.
15. G. E. Hilbert, J. Am. Chem. Soc., 1932, 54, 3413. CrossRef
16. G. Steffan and G. O. Schenck, Chem. Ber., 1967, 100, 3961; CrossRef G. Steffan, Chem. Ber., 1968, 101, 3688; CrossRef K.-H. Scholz, J. Hinz, H.-G. Heine, and W. Hartmann, Liebigs Ann. Chem., 1981, 248. CrossRef
17. W. von E. Doering and W. R. Roth, Tetrahedron, 1963, 19, 715; and references cited therein. CrossRef
18. B. Eistert, M. Regitz, G. Heck, and H. Schwall, in Methoden der Organischen Chemie (Houben-Weyl-Mueller), 4th ed., vol. 10/4, Thieme, Stuttgart, Germany, 1968, p. 552 and 538.
19. F. Arndt, Org. Synth., Coll. Vol., 1943, 2, 165; See also J. A. Moore and D. E. Reed, Org. Synth., Coll. Vol., 1973, 5, 351.
20. R. G. Salomon and J. K. Kochi, J. Am. Chem. Soc., 1973, 95, 3300. CrossRef
21. For reviews on propellanes, see: D. Ginsburg, Acc. Chem. Res., 1969, 2, 121; CrossRef D. Ginsburg, Top. Curr. Chem., 1987, 137, 1. CrossRef
22. J. Meinwald, S. S. Labana, and M. S. Chadha, J. Am. Chem. Soc., 1963, 85, 582; CrossRef M. Hagen and U. Luening, Chem. Ber./Recueil, 1997, 130, 231; F. Loeffler, M. Hagen, and U. Luening, Synlett., 1999, 1826; CrossRef R. K. Das, M. Sarkar, S. M. Wahidur Rahaman, H. Doucet, and J. K. Bera, Eur. J. Inorg. Chem., 2012, 1680. CrossRef
23. W. Kirmse, Angew. Chem. Int. Ed., 2003, 42, 1088. CrossRef
24. D. E. McGreer and N. W. K. Chiu, Can. J. Chem., 1968, 46, 2217; CrossRef P. H. Mazzocchi and H. J. Tamburin, J. Org. Chem., 1973, 38, 2221; CrossRef J. J. Gajewski, R. J. Weber, R. Braun, M. L. Manion, and B. Hymen, J. Am. Chem. Soc., 1977, 99, 816. CrossRef
25. N. R. Easton, D. R. Cassady, and R. D. Dillard, J. Org. Chem., 1964, 29, 1851; CrossRef S. K. Chiu, M. Dube, L. Keifer, S. Szilagyi, and J. W. Timberlake, J. Org. Chem., 1978, 43, 61. CrossRef
26. For vicinal cyclopropane coupling constants in bicyclo[3.1.0]hexanes, see: W. G. Dauben and W. T. Wipke, J. Org. Chem., 1967, 32, 2976. CrossRef
27. For vicinal coupling constants in cis-1,2-cyclopropanediamines, see Ref.9.
28. H. A. Bates, N. Condulis, and N. L. Stein, J. Org. Chem., 1986, 51, 2228. CrossRef
29. A. Jonczyk and M. Mąkosza, Rocz. Chem., 1975, 49, 1203; N.-C. Wang, K.-E. Teo, and H. J. Anderson, Can. J. Chem., 1977, 55, 4112; CrossRef E. Santaniello, C. Farachi, and F. Ponti, Synthesis, 1979, 617. CrossRef
30. H. Quast and W. von der Saal, Chem. Ber., 1984, 117, 1591; CrossRef W. von der Saal and H. Quast, J. Org. Chem., 1995, 60, 4024; CrossRef W. von der Saal and H. Quast, J. Org. Chem., 1996, 61, 4920; CrossRef W. von der Saal, R. Reinhardt, J. Stawitz, and H. Quast, Eur. J. Org. Chem., 1998, 1645. CrossRef
31. D. Misiti, M. Santaniello, and G. Zappia, Synth. Commun., 1992, 22, 883. CrossRef
32. I. Isaka, K. Kubo, M. Takashima, and M. Murakami, Yakugaku Zasshi, 1968, 88, 1062.