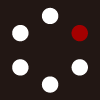
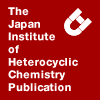
HETEROCYCLES
An International Journal for Reviews and Communications in Heterocyclic ChemistryWeb Edition ISSN: 1881-0942
Published online by The Japan Institute of Heterocyclic Chemistry
e-Journal
Full Text HTML
Received, 11th July, 2012, Accepted, 30th August, 2012, Published online, 14th September, 2012.
DOI: 10.3987/COM-12-S(N)96
■ INTRAMOLECULAR CYCLIZATION OF γ-ACETYLENIC ACIDS USING DENDRIMER-ENCAPSULATED Pd2+ CATALYSTS
Zen Maeno, Takato Mitsudome, Tomoo Mizugaki, Koichiro Jitsukawa, and Kiyotomi Kaneda*
GSC Lab, Research Center for Solar Energy Chemistry, Osaka University, 1-3 Machikaneyama, Toyonaka, Japan
Abstract
Polyamine dendrimer-encapsulated Pd2+ catalysts were prepared by complexation of PdCl2 with internal tertiary amino groups of the dendrimer. The fifth-generation Pd2+ dendrimer catalyst showed cooperative catalysis between Pd2+ species and the internal nanocavity consisting of regularly arranged tertiary amino groups to promote the intramolecular cyclization of γ-acetylenic acids efficiently.γ-Alkylidene-γ-butyrolactones are important chemicals as constituent units of a number of natural products and synthetic intermediates of pharmaceuticals.1 Among the synthetic methods of γ-alkylidene-γ-butyrolactones, intramolecular cyclizations of acetylenic acids have attracted attention because of their 100% atom efficiency.2-7 To date, various transition metal catalysts, such as Pd,2 Hg,3 Pt,4 Au,5 Ag,6 Ir, and Rh,7 have been reported to promote the cyclization of acetylenic acids. In particular, Pd with base catalyst systems2 showed higher catalytic activities than other metal ones. Nozaki et al. first reported that PdCl2(PhCN)2 with triethylamine (TEA) catalyzed the intramolecular cyclization of acetylenic acids.2a Hidai et al. exhibited that the combination of a cuboidal PdMo3S4 cluster and TEA achieved a high catalytic turnover number.2e Recently, heterogeneous Pd catalysts were also developed by Michelet et al. through the immobilization of Pd complexes onto basic Zn2AlNO3 layered double hydroxide (Pd/LDHs).2g
Dendrimers are well-defined macromolecules with highly branched structures and internal nanocavities composed of a core and branch units.8 Various guests such as organic molecules, metal ions, and nanoparticles can be accommodated within their
nanocavity,8b-d giving rise to dendritic catalysis such as site-isolation as well as a high local concentration of the substrate and/or active species.9 Accordingly, we have demonstrated the unique dendritic catalysis using poly(propylene imine) (PPI) dendrimers encapsulating metal complexes,9a,d nanoparticles,10a subnano clusters10b,c and quaternary ammonium groups.9e Very recently, the tertiary amino group within the nanocavities of the PPI dendrimer was also found to act as an efficient organocatalyst, promoting the intramolecular cyclization.10d Herein, we report the cooperative effect between Pd2+ species and the confined nanocavity of the PPI dendrimers on the catalytic intramolecular cyclization of acetylenic acids. The fifth-generation dendrimer encapsulating Pd2+ species was found to act as an efficient catalyst for the cyclization of γ-acetylenic acids.
The triethoxybenzamide terminated-PPI dendrimers (Gx-TEBA, x denotes the generation number of PPI dendrimer, (Figure 1)) were synthesized using the reported procedures.10a The PPI dendrimer-encapsulated Pd2+ catalysts (Gx-Pd2+n, n denotes the molar ratio of Pd2+ ions to Gx-TEBA) were prepared by treatment of Gx-TEBA with Na2PdCl4.10b,c
The intramolecular cyclization of 4-pentynoic acid (1a) using Gx-Pd2+n catalysts was examined in THF at 30 °C (Table 1).11 The ratio of G5-TEBA to Pd2+ (n) strongly affected the catalytic activity of G5-Pd2+n (entries 1-6). G5-Pd2+8 showed the highest activity to give γ-methylene-γ-butyrolactone (2a) in 95% yield (entry 3). Interestingly, the catalytic activity increased with increasing generation of the dendrimer; the fifth-generation dendrimer catalyst showed the highest activity among G5-Pd2+8, G4-Pd2+8, and G3-Pd2+8 (entries 3, 7, and 8). When the aliphatic tertiary amines comparable in basicity with G5-TEBA (pKa = 10.35)12 were used instead of G5-TEBA
under similar conditions, these amines such as TEA (pKa = 10.6),13 N,N,N’,N’-tetramethyl-1,3-propanediamine (TMPDA, pKa = 9.8),14 and N,N,N’,N’’,N’’-pentamethyldipropylenetriamine (PMDPT, pKa = 10.0)14 resulted in lower yields of 2a compared to that of G5-TEBA (entries 9-11).15 Furthermore, an irregularly branched polyamine of TEBA-modified polyethyleneimine (PEI-TEBA)16 was tested in the reaction. However, PEI-TEBA was not effective, giving only 15% yield of 2a (entry 12). These results indicate that the encapsulation of Pd2+ species within the nanocavity consisting of regularly arranged tertiary amino groups of G5-TEBA is necessary to achieve high catalytic efficiency.
Table 2 shows the substrate scope of the G5-Pd2+8 catalyst. The cyclization of α-substituted and β-substituted γ-acetylenic acids 1b-d proceeded efficiently to afford the corresponding γ-methylene-γ-butyrolactones 2b-d in excellent yields (entries 2-4). β-Acetylenic acid 1e was also easily converted to the corresponding γ-butyrolactone 2e (entry 5). The double cyclization of dipropargylmalonic acid 1f
gave the spiro lactone product 2f quantitatively (entry 6). However, the cyclization of δ-acetylenic acid such as 5-hexynoic acid 1g did not proceed smoothly (entry 7). A γ-acetylenic acid with an internal alkyne 1h was intact (entry 8)17. In contrast, the PdCl2(PhCN)2-TEA system showed good to moderate catalytic activities for the cyclizations of 1g and 1h (entries 7 and 8).
The high activity of G5-Pd2+8 for the formation of γ-butyrolactones was further exemplified in the competitive reaction between γ-acetylenic acid and δ-one. In the intermolecular competitive reaction between 1a and 1g, 1a was converted to 2a quantitatively without formation of 2g (Scheme 1). In comparison, the PdCl2(PhCN)2-TEA system afforded a mixture of 2a and 2g. The intramolecular competitive cyclization of 1i proceeded exclusively to give the 5-membered lactone 2i (Scheme 2) while the reaction of 1i using the PdCl2(PhCN)2-TEA system resulted in the formation of a mixture of 2i and the 6-membered lactone 3i. It has also been reported that the Pd/LDH catalyst converts 1i to a mixture of 2i and 3i in 86% and 14% selectivity, respectively.2g These results clearly show that G5-Pd2+8 exhibits the specific activity for the cyclization of γ-acetylenic acids compared to δ-one.
The Pd-catalyzed cyclization of acetylenic acids generally involves the intramolecular nucleophilic attack of a carboxylate anion to the acetylenic bond coordinated to a Pd center, followed by the protonolysis of the resulting vinylpalladium species.2a,g In the case of the G5-Pd2+8 catalyst, Pd2+ species and the regularly arranged tertiary amino groups would cooperatively function within the sterically confined nanocavity; a carboxyl group of the substrate is oriented toward an acetylenic bond on the Pd species by the steric effect of the nanocavity of G5-Pd2+8, resulting in facile intramolecular nucleophilic attack of the carboxyl group activated by regularly arranged tertiary amino groups (Figure 2). The specific activity of G5-Pd2+8 for the cyclization of γ-acetylenic acids may be attributable to the steric congestion around the Pd species within the internal nanocavity of the G5-TEBA dendrimer.18
In conclusion, the PPI dendrimer-encapsulated Pd2+ catalysts synthesized by the complexation of PdCl2 with internal tertiary amino groups can be applied to the intramolecular cyclization of acetylenic acids. The fifth-generation dendrimer catalyst, G5-Pd2+8, specifically and efficiently promoted the cyclization of γ-acetylenic acids due to the cooperative catalysis between the Pd2+ species and the internal nanocavity consisting of regularly arranged tertiary amino groups of G5-TEBA.
ACKNOWLEDGEMENTS
This work was supported by a Grant-in-Aid for Challenging Exploratory Research (23656514) from the Japan Society for the Promotion of Science (JSPS).
References
1. (a) S. S. C. Koch and A. R. Chamberlin, ‘Studies in Natural Products Chemistry’ Vol. 16, ed. by Atta-ur-Rahman, Elsevier Science, Amsterdam, 1995, 687; (b) M. Seitz and O. Reiser, Curr. Opin. Chem. Biol., 2005, 9, 285; CrossRef (c) E.-I. Negishi and M. Kotora, Tetrahedron, 1997, 53, 6707. CrossRef
2. Pd catalysis (a) C. Lambert, K. Utimoto, and H. Nozaki, Tetrahedron Lett., 1984, 25, 5323; CrossRef (b) A. Arcadi, A. Burini, S. Cacchi, M. Delmastro, F. Marinelli, and B. R. Pietroni, J. Org. Chem., 1992, 57, 976; CrossRef (c) M. Cavicchioli, D. Bouyssi, J. Goré, and G. Balme, Tetrahedron Lett., 1996, 37, 1429; CrossRef (d) X. Wang and X. Lu, J. Org. Chem., 1996, 61, 2254; CrossRef (e) T. Wakabayashi, Y. Ishii, K. Ishikawa, and M. Hidai, Angew. Chem., Int. Ed. Engl., 1996, 35, 2123; CrossRef (f) Z. Huo, N. T. Patil, T. Jin, N. K. Pahadi, and Y. Yamamoto, Adv. Synth. Catal., 2007, 349, 680; CrossRef (g) F. Neatu, L. Protesescu, M. Florea, V. I. Párvulescu, C. M. Teodorescu, N. Apostol, P. Y. Toullec, and V. Michelet, Green. Chem., 2010, 12, 2145; CrossRef (h) K. Ogata, D. Sasano, T. Yokoi, K. Isozaki, H. Seike, H. Takaya, and M. Nakamura, Chem. Lett., 2012, 41, 498. CrossRef
3. Hg catalysis (a) M. Yamamoto, J. Chem. Soc., Perkin Trans. 1, 1981, 582; CrossRef (b) R. A. Amos and J. A. Katzenellenbogen, J. Am. Chem. Soc., 1981, 103, 5459; CrossRef (c) A. Jellal, J. Grimaldi, and M. Santelli, Tetrahedron Lett., 1984, 25, 3179; CrossRef (d) R. W. Spencer, T. F. Tam, E. Thomas, V. J. Robinson, and A. Krants, J. Am. Chem. Soc., 1986, 108, 5589; CrossRef (e) H. Imagawa, Y. Fujikawa, A. Tsuchihiro, A. Kinoshita, T. Yoshinaga, H. Takao, and M. Nishizawa, Synlett, 2006, 639. CrossRef
4. Pt catalysis (a) J. Alemán, V. del Solar, and C. Navarro-Ranning, Chem. Commun., 2010, 46, 454; CrossRef (b) J. Alemán, V. del Solar, L. Cubo, A. G. Quiroga, and C. Navarro-Ranning, Dalton Trans., 2010, 39, 10601. CrossRef
5. Au catalysis (a) E. Genin, P. Y. Toullec, S. Antoniotti, C. Brancour, J.-P. Genét, and V. Michelet, J. Am. Chem. Soc., 2006, 128, 3112; CrossRef (b) H. Harkat, J.-M. Weibel, and P. Pale, Tetrahedron Lett., 2006, 47, 6273; CrossRef (c) E. Marchal, P. Uriac, B. Legoin, L. Toupet, and P. van de Weghe, Tetrahedron, 2007, 63, 9979. CrossRef
6. Ag catalysis (a) J. Castaner and J. Pascual, J. Chem. Soc., 1958, 3962; CrossRef (b) P. Pale and J. Chuche, Tetrahedron Lett., 1987, 28, 6447; CrossRef (c) V. Dalla and P. Pale, Tetrahedron Lett., 1994, 35, 3525; CrossRef (d) V. Dalla and P. Pale, New J. Chem., 1999, 23, 803; CrossRef (e) C. H. Oh, H. J. Yi, and J. H. Lee, New J. Chem., 2007, 31, 835. CrossRef
7. Ir and Rh catalysis (a) S. Elgafi, L. D. Field, and B. A. Messerle, J. Organometal. Chem., 2000, 607, 97; CrossRef (b) E. Mas-Marzá, E. Peris, I. Castro-Rodriguez, and K. Meyer, Organometallics, 2005, 24, 3158; CrossRef (c) S.-G. Lim, B.-I. Kwon, M.-G. Choi, and C.-H. Jun, Synlett, 2005, 1113; CrossRef (d) J. H. H. Ho, D. S. C. Black, B. A. Messerle, J. K. Clegg, and P. Turner, Organometallics, 2006, 25, 5800; CrossRef (e) E. Mas-Marzá, J. A. Mata, and E. Peris, Angew. Chem. Int. Ed., 2007, 46, 3729. CrossRef
8. (a) S. Campagna, P. Ceroni, and F. Puntoriero, ‘Designing Dendrimers’ John Wiley & Sons, Inc., Hoboken, New Jersey, 2012; (b) K. Yamamoto and T. Imaoka, Bull. Chem. Soc. Jpn., 2006, 79, 511; CrossRef (c) D. Astruc, E. Boisselier, and C. Omelas, Chem. Rev., 2010, 110, 1857; CrossRef (d) V. S. Myers, M. G. Weir, E. V. Carino, D. F. Yancey, S. Pande, and R. M. Crooks, Chem. Sci., 2011, 2, 1632. CrossRef
9. (a) M. Ooe, M. Murata, T. Mizugaki, K. Ebitani, and K. Kaneda, J. Am. Chem. Soc., 2004, 126, 1604; CrossRef (b) C. Muller, L. J. Ackerman, J. N. H. Reek, P. C. J. Kamer, and P. W. N. M. V. Leeuwen, J. Am. Chem. Soc., 2004, 126, 14960; CrossRef (c) B. Helms, C. O. Liang, C. J. Hawker, and J. M. J. Fréchet, Macromolecules, 2005, 38, 5411; CrossRef (d) T. Mizugaki, Y. Miyauchi, M. Murata, K. Ebitani, and K. Kaneda, Chem. Lett., 2005, 34, 286; CrossRef (e) T. Mizugaki, C. E. Hetrick, M. Murata, K. Ebitani, M. D. Amiridis, and K. Kaneda, Chem. Lett., 2005, 34, 420; CrossRef (f) B. Helms and M. J. Frechet, Adv. Synth. Catal., 2006, 348, 1125; CrossRef (g) A. K. Diallo, E. Boisselier, L. Liang, J. Ruiz, and D. Astruc, Chem. Eur. J., 2010, 16, 11832; CrossRef (h) T. Imaoka, Y. Kawana, M. Tsuji, and K. Yamamoto, Chem. Eur. J., 2010, 16, 11003. CrossRef
10. (a) M. Ooe, M. Murata, T. Mizugaki, K. Ebitani, and K. Kaneda, Nano Lett., 2002, 2, 999; CrossRef (b) T. Mizugaki, T. Kibata, K. Ota, T. Mitsudome, K. Ebitani, K. Jitsukawa, and K. Kaneda, Chem. Lett., 2009, 38, 1118; CrossRef (c) Z. Maeno, T. Kibata, T. Mitsudome, T. Mizugaki, K. Jitsukawa, and K. Kaneda, Chem. Lett., 2011, 40, 180; CrossRef (d) Z. Maeno, T. Mitsudome, T. Mizugaki, K. Jitsukawa, and K. Kaneda, Chem. Lett., 2012, 41, 801. CrossRef
11. A typical cyclization reaction of acetylenic acid was carried out in a Schlenk glass tube. The glass tube was charged with acetylenic acid (0.1 mmol), THF (2 mL), and G5-Pd2+8 (Pd: 1 μmol). The reaction mixture was vigorously stirred at 30 °C. After the reaction, the reaction mixture was analyzed by GC and 1H NMR using an internal standard technique.
12. G. J. M. Koper, M. H. P. van Genderen, C. Elissen-Román, M. W. P. L. Baars, E. W. Meijer, and M. Borkovec, J. Am. Chem. Soc., 1997, 119, 6512. CrossRef
13. O. Urs, B. Zbigniew, X. Aiping, R. Bruno, and S. Gabriela, Anal. Chem., 1986, 58, 2285. CrossRef
14. R. von Rometsch, A. Marxer, and K. Miescher, Helv. Chim. Acta, 1951, 34, 1611. CrossRef
15. The ratio of tertiary amino groups to Pd2+ in the PdCl2(PhCN)2-aliphatic tertiary amine systems was adjusted to that in G5-Pd2+8 catalyst.
16. TEBA-modified polyethyleneimine (PEI-TEBA) was synthesized as follows: to a THF solution (100 mL) of polyethyleneimine (0.50 g, 3.15, 3.15, and 2.7 mmol for primary, secondary, and tertiary amine, respectively) and TEA (3.07 g, 30.4 mmol) was added a THF solution (20 mL) of 3,4,5-triethoxybenzoyl chloride dropwise (2.39 g, 8.8 mmol) at 30 °C for 5 min. The mixture was stirred at 40 °C for 48 h, then concentrated under reduced pressure. The residue was washed with 1 M NaOH aqueous solution (3 x 200 mL) and water (5 x 200 mL), and dried under vacuum for 24 h to give PEI-TEBA as a brownish solid (1.48 g). 1H NMR (400 MHz, CDCl3, TMS, 50 °C), δ 0.66-1.47 (1512H, br, CH3CH2O-), 2.01-2.95 (444H, br, (CH2)3N), 2.96-4.55 (1512H, br, (CH2)2NCOAr + CH2NHCOAr + CH3CH2O-), 6.08-6.70 (168H, br, (CH2)2NCOAr), 6.78-7.18 (168H, br, CH2NHCOAr), 7.50-8.29 (84H, br, CH2NHCOAr). 13C{1H} NMR (100 MHz, CDCl3. TMS, 50 °C), 14.9, 15.6, 38.4, 53.5, 64.8, 68.8, 106.5, 129.3, 131.2, 139.2, 141.2, 152.8, 167.6, 171.8. IR (KBr, cm-1), 3329 (N-H stretch), 3016 (Aromatic C-H stretch), 1629 (C=O stretch), 1580 (N-H vend), 1215 (C-N stretch), 1030 (Ar-O-CH2 stretch), 755 (Aromatic C-H vend), 668 (-NH vibration). Anal. Calcd for C2664H3888N240O672: C, 63.95; H, 7.83; N, 6.71. Found: C, 63.56; H, 8.01; N, 6.59. PEI-Pd2+8 was prepared in a similar way by treatment of PEI-TEBA with Na2PdCl4 aqueous solution. See refs 10a and 10b.
17. The low reactivity of 1h might be due to the steric hindrance of the methyl group which inhibits the coordination of the alkyne group to Pd2+.
18. Several transition metal complex catalysts having bulky ligands were reported to show extremely lower catalytic activity for the cyclization of 5-hexynoic acid than that of 4-pentynoic acid. See refs 2e, 7b, and 7d.