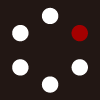
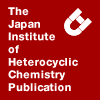
HETEROCYCLES
An International Journal for Reviews and Communications in Heterocyclic ChemistryWeb Edition ISSN: 1881-0942
Published online by The Japan Institute of Heterocyclic Chemistry
e-Journal
Full Text HTML
Received, 29th August, 2012, Accepted, 1st October, 2012, Published online, 15th October, 2012.
DOI: 10.3987/COM-12-S(N)114
■ SYNTHESIS OF 1,4-PHOSPHASILACYCLOHEXA-2,5-DIENES BEARING HYDROGEN OR CHLORINE ATOMS ON THE SILICON ATOMS
Yoshiyuki Mizuhata, Satoshi Morikawa, and Norihiro Tokitoh*
Division of Synthetic Chemistry, Organoelement Chemistry, Institute for Chemical Research, Kyoto University, Uji, Kyoto 611-0011, Japan
Abstract
2,3,5,6-Hydrogen-substituted 1,4-phosphasilacyclo¬hexa-2,5-dienes bearing a functional group on the silicon atom were synthesized via the corresponding stannacycle. The structures of newly synthesized silacyclic compounds were determined by X-ray crystallographic analysis.The chemistry of [4n+2]π-electron ring systems containing a heavier group 14 element (Si, Ge, Sn, Pb), which we call, “heavy aromatic compounds,”1-3 has attracted much attention from the viewpoint of comparison with the parent aromatic hydrocarbons, which play very important roles in organic chemistry. Although heavy aromatic compounds have been known to be highly reactive and undergo ready dimerization or oligomerization under ambient conditions, we have succeeded in the synthesis of the first stable neutral sila-, germa-, and stanna-aromatic compounds,2 i. e., heavier analogues of benzene [Si,4 Ge5 (Sn: unstable6), naphthalene (Si,7,8 Ge,9 Sn10), anthracene (Si,11 Ge12), and phenanthrene [Si,13 Ge12 (Sn: unstable14)], by taking advantage of kinetic stabilization using efficient steric protection groups, 2,4,6-tris[bis(trimethylsilyl)methyl]phenyl (Tbt) and 2,6-bis[bis(trimethylsilyl)methyl]-4-[tris(trimethylsilyl)methyl]phenyl (Bbt). During the course of our studies on heavy aromatic compounds, we have been interested in the corporation of heavier group 14 elements and heteroatoms of other group, that is, the synthesis of heavier congeners of heteroaromatic compounds. As the first target compounds, we designed the 1,4-phosphasilabenzene derivatives, whose synthetic strategies are shown in Scheme 1. For their syntheses, 1,4-phosphasilacyclohexa-2,5-dienes 1 bearing a functional group on the silicon atom should be key precursors. As for 1,4-phosphasilacyclohexa-2,5-diene skeletons, some benzene- or thiophene-fused derivatives have been reported,15 but there is no example bearing a functional group on the silicon atom. In addition, 2,3,5,6-unsubstituted derivative is limited to only one example, 4,4-dimethyl-1-phenyl-1,4-phosphasilacyclohexa-2,5-diene, reported by Märkl et al.16 In this paper, we report the construction of 1,4-phosphasilacyclohexa-2,5-diene skeletons bearing hydrogen or chlorine atoms on the silicon atoms together with their properties.
It is well known that the stannacycles are good precursors for the construction of heterocycles by the direct exchange of the tin atom with a heteroatom source17 or by the lithiation with alkyllithium and the subsequent reaction with a heteroatom source.4-6,18 Therefore, we synthesized 1,4-silastannacyclohexa-2,5-diene 2 according to the route shown in Scheme 2. In order to achieve selective introduction of two ethynyl substituents, we used dimethoxysilane 3 as a starting material. The reaction of 3 with ethynylmagnesium bromide afforded diethynylsilane 4 in 91% yield. Then, the hydrostannylation reaction of 4 using dibutyltin dihydride in heptane afforded 2 in 70% yield. The structures of 2 and 4 were reasonably supported by the 1H and 13C NMR and mass spectra along with elemental analysis, and finally determined by X-ray crystallographic analysis. The structure of 2 is shown in Figure 1. This is the first structural determination of a 2,3,5,6-unsubstituted 1,4-silastannacyclohexa-2,5-diene. The central 1,4-silastannacyclohexa-2,5-diene skeleton of 2 has a boat shape, and the deviations of Si1 and Sn1 atoms from the C1–C2–C3–C4 plane are 0.275 and 0.331 Å, respectively. The selected structural parameters of 2 are summarized in Table 1.
In order to introduce a phosphorus moiety instead of the dibutyltin unit of 2, we initially attempted the direct exchange using phosphorus trichloride by following the syntheses of heavier congeners of pyridine.17 However, desired product was not obtained even after several attempts. Next, we tried the transmetalation reaction between tin and lithium and the following subsequent reactions with aryldichlorophophines. As a result of the optimization of reaction conditions, it was found that the reaction of 2 with 3.2 equivalent of n-BuLi in the presence of 3.2 equivalents of tetramethylethylenediamine afforded the corresponding dilithio species effectively. The subsequent addition of dichlorophosphines afforded the corresponding 1,4-phosphasilahexa-2.5-dienes 5 and 6 in moderate yields (Scheme 3). In the case using dichlorophenylphosphine, ca. 1:1 mixture of cis- and trans-isomers 5 was obtained as judged by 31P NMR (δP = –25.5, –25.7). In the case using Mes*-substituted dichlorophosphine, on the other hand, only trans-isomer 6 was generated, judging from the sole signal in the 31P NMR (δP = –24.5) and the results of its X-ray crystallographic analysis (Figure 2). The bulkiness of Mes* group is considered to suppress the formation of the corresponding cis-isomer. Since 1,4-phosphasilahexa-2.5-dienes 5 and 6 were found to be somewhat unstable and their isolation in enough amount to analyze their chemical properties were difficult, their oxidation by hydrogen peroxide was performed. The reactions proceeded cleanly to afford the corresponding phosphine oxides 7 and 8. At this stage, cis- and trans-isomers of 7 were isolable. The structures of 7 and 8 were reasonably supported by the 1H and 13C NMR and mass spectra together with elemental analysis, and finally determined by X-ray crystallographic analysis (Figure 2). These are the first examples of structural determination of 2,3,5,6-unsubstituted 1,4-phoshasilacyclohexa-2,5-diene. The oxidation of 6 proceeded with an inversion fashion. The 31P NMR signals were observed at 3.4 (cis-7), 5.7 (trans-7), and 12.6 (trans-8) ppm.
Selected structural parameters of 2, 6, 7, and 8 and their crystallographic data are summarized in Tables 1 and 2, respectively. The structural parameters of cis-7 are not accurate due to the severe disorder covering the whole of Tbt group. The conformations of SiC4P rings of Mes*-substituted derivatives, trans-6 and 8, were boat shapes similarly to that of stannacycle 2. On the other hand, phenyl-substituted ones, trans- and cis-7, have almost planar SiC4P rings, and the sums of the internal bond angles are 718.8 (trans-7) and 719.6º (cis-7). While the bond lengths in 6-8 have little differences, there are large differences in their internal angles. Theoretical calculations by using the model compounds bearing hydrogen atoms on the silicon and phosphorus atoms instead of aryl substituents (phosphine 9 and phosphine oxide 10, respectively) showed that the boat shape of 9 and the planar geometry of 10 were global minima (Table 1). Therefore, the boat-shape conformations of 8 were considered to be most likely due to the steric repulsion between bulky substituents and/or the packing forces. The benzene- and thiophene-fused 1,4-phosphasilacyclohexa-2,5-diene were reported to have planar or butterfly-shape towards the Si–P axis.
In order to obtain 5 in a pure form, the reduction of isolated 7 using trichlorosilane was performed (Scheme 4). Unexpectedly, chlorination of Si–H occurred together with the reduction of the phosphine oxide moiety to afford 11. Although we have not obtained detailed structural information for 11, a single isomer was generated from each isomer (δSi = –26.4, δP = –25.2). The structure of 11 was supported by the reasonable chemical shifts in 29Si and 31P NMR and the fact that the reaction of 11 with lithium aluminum hydride afforded hydrosilane 5 again as a mixture of isomers. On the other hand, the reaction of 8 with trichlorosilane did not proceed under similar conditions.
By using hydro- or chlorosilanes 5, 6, and 11 here obtained, we performed several reactions expecting the generation of 1,4-phosphasilabenzenium cations (5 or 6 with Ph3C+TPFPB–, 11 with Ag+TPFPB–, and so on). However, all attempts were unsuccessful so far and gave a complicated mixture. On the other hand, we have a preliminary result of the elimination of phenyl group on the phosphorus atom in 5 by the reaction with lithium dispersion. We think this result suggests the possibility to access 1,4-phosphasilabenzene. At the same time, the ring-closing methodology using stannacycle 2 is considered to be applicable to synthesize other silicon-containing heterocycles. We are currently continuing this study with a scope of various atom combinations.
EXPERIMANTAL
General procedures. All experiments were performed under an argon atmosphere unless otherwise noted. Solvents used for the reactions were purified by The Ultimate Solvent System (GlassContour Company).19 1H NMR (300 MHz), 13C NMR (76 MHz), 31P NMR (121 MHz), and 119Sn NMR (111 MHz) spectra were measured in CDCl3 or C6D6 with a JEOL JNM-AL300 spectrometer. In 1H NMR, signals due to CHCl3 (7.25 ppm) and C6D5H (7.15 ppm) were used as references, and those due to CDCl3 (77 ppm) and C6D6 (128 ppm) were used in 13C NMR. 31P NMR spectra were measured using 85% H3PO4 in water (0 ppm) as an external standard. 119Sn NMR was measured with NNE technique using SnMe4 as an external standard. Multiplicity of signals in 13C NMR spectra was determined by DEPT technique, which was described as s, d, t, and q for compounds 2-4 and as C, CH, CH2, and CH3 with the coupling with 31P for phosphorus-containing compounds 5-8 and 11. GPLC (gel permeation liquid chromatography) was performed on an LC-908, LC-918, or LC-908-C60 (Japan Analytical Industry Co., Ltd.) equipped with JAIGEL 1H and 2H columns (eluent: chloroform or toluene). All melting points were determined on a Yanaco micro melting point apparatus and were uncorrected. Elemental analyses were carried out at the Microanalytical Laboratory of the Institute for Chemical Research, Kyoto University. All theoretical calculations were carried out using the Gaussian 0920 programs.
Synthesis of 3. To a THF solution (30 mL) of TbtBr (5.00 g, 7.91 mmol) at –78 ºC was added t-BuLi (1.57 M, 12.0 mL, 19.0 mmol). After stirring for 30 min,trimethoxysilane (3.02 mL, 2.09 g, 23.7 mmol) was added to the solution followed by warming up to room temperature overnight. Then, MeOH was added to the mixture, and the reaction mixture was extracted with hexane/H2O. The organic layer was dried over MgSO4 and the solvent was removed to give NMR pure 3 (5.02 g, 7.79 mmol, 99%) as a colorless solid. 3: mp 163 ºC (dec.); 1H NMR (300 MHz, CDCl3, 298 K): δ 0.01 (s, 36H), 0.25 (s, 18H), 1.30 (s, 1H), 2.32 (s, 2H), 3.59 (s, 6H), 5.14 (s, 1H), 6.20 (s, 1H), 6.33 (s, 1H); 13C NMR (75 MHz, CDCl3, 298 K); δ 0.5 (q), 0.7 (q), 27.0 (d), 27.4 (d), 30.5 (d), 51.6 (q), 121.7 (d), 124.2 (s), 126.5 (d), 145.4 (s), 151.6 (s), 151.9 (s); 29Si NMR (60 MHz, CDCl3, 298 K); δ –22.2, 1.7, 1.9; HRMS (FAB) m/z calcd for C29H66O2Si7 642.344 (M+), Found: 642.3443 (M+); Anal. Calcd for C29H66O2Si7: C, 54.13; H, 10.34. Found: C, 53.90; H, 10.21.
Synthesis of 4. To a THF solution (20 mL) of 3 (6.05 g, 9.40 mmol) was added ethynylmagnesium bromide in THF (0.5 M, 56.4 mL, 28.2 mmol) at room temperature. The reaction mixture was stirred for 13 h under reflux conditions. Then, NH4Cl aq was added to the mixture at 0 ºC, and the reaction mixture was extracted with hexane. The organic layer was dried over MgSO4 and the solvent was removed. The reaction mixture was separated by silica gel chromatography (hexane) to give 4 (5.38 g, 8.53 mmol, 91%) as a colorless solid. 4: Rf = 0.37 (hexane); colorless crystals; mp 166.5-167.8 ºC; 1H NMR (300 MHz, 298 K, CDCl3) δ 0.04 (br s, 18H), 0.05 (br s, 18H), 0.07 (br s, 18H), 1.33 (s with satellites, 1H, 2JHSi = 10.0 Hz), 2.40 (s, 2H), 2.580 (s, 1H), 2.584 (s, 1H), 5.22 (s with satellites, 1H, 1JHSi = 225 Hz), 6.29 (br s, 1H), 6.41 (br s, 1H); 13C NMR (75 MHz, 298 K, CDCl3) δ 0.6 (q), 0.7 (q), 1.0 (q), 29.1 (d), 29.6 (d), 30.8 (d), 85.1 (s), 97.0 (d), 118.5 (s), 121.9 (d), 126.8 (d), 146.3 (s), 152.5 (s), 152.9 (s); 29Si NMR (59 MHz, 298 K, CDCl3) δ –76.5, 2.2, 2.8; HRMS (FAB) m/z calcd for C31H62Si7 630.3236 (M+), Found 630.3237; Anal. Calcd for C31H62Si7: C, 58.97; H, 9.99. Found: C, 59.22; H, 9.93.
Synthesis of 2. The heptane solution (35 mL) of 4 (790 mg, 1.25 mmol) and dibutyltin dihydride (1.11 g, 4.75 mmol) was stirred for 18 h under reflux conditions. Then, the solvent was removed, and the reaction mixture was separated by silica gel chromatography (hexane) and GPC (eluent: toluene) to give 2 (759 mg, 0.876 mmol, 70%) as a colorless solid. 2: Rf = 0.87 (hexane); colorless crystals; mp 114.9-115.4 ºC; 1H NMR (300 MHz, 298 K, C6D6) δ 0.16 (br s, 18H), 0.20 (br s, 18H), 0.21 (br s, 18H), 0.86-1.12 (m, 10H), 1.15-1.79 (m, 8H), 1.46 (s, 1H), 2.60 (br s, 1H), 2.63 (br s, 1H), 5.63 (s with satellites, 1H, 1JHSi = 188 Hz), 6.53 (br s, 1H), 6.63 (br s, 1H), 7.27 (d, 3J = 18.9 Hz, 2H), 7.44 (d, 3J = 18.9 Hz, 2H); 13C NMR (75 MHz, 298 K, C6D6) δ 1.0 (q), 1.1 (q), 1.5 (q), 11.1 (t), 11.3 (t), 13.88 (q), 13.90 (q), 27.4 (t), 27.6 (t), 29.4 (d), 29.5 (t×2), 29.6 (d), 30.9 (d), 122.3 (d), 125.2 (s), 127.2 (d), 145.2 (s), 151.3 (d), 152.5 (d), 152.4 (s), 153.1 (s); 29Si NMR (59 MHz, 298 K, C6D6) δ –57.2, 2.0, 2.3; 119Sn NMR (111 MHz, 298 K, C6D6) δ –170.9; HRMS (FAB) m/z calcd for C39H82Si7Sn 866.3823 (M+), Found 866.3828; Anal. Calcd for C39H82Si7Sn: C, 54.07; H, 9.54. Found: C, 54.11; H, 9.55.
Synthesis of 7 via 5. To a THF solution (0.78 mL) of 2 (115 mg, 0.133 mmol) and tetramethylethylenediamine (0.0639 mL, 0.426 mmol) was added n-BuLi (1.48 M in hexane, 0.288 mL, 0.426 mmol) at –78 ºC. After stirring for 15 h at the same temperature, dichlorophenylphosphine (0.0904 mL, 0.666 mmol) was added to the reaction mixture at –78 ºC. The reaction mixture was allowed to warm up to –60 ºC, and kept for 6 h at the same temperature. Then, H2O2 aq (30%, 0.0367 mL, 1.20 mmol) was added to the reaction mixture at 0 ºC. After stirring for 3 h at room temperature, the reaction mixture was extracted with hexane. The organic layer was dried over MgSO4 and the solvent was removed. The reaction mixture was separated by silica gel chromatography (Et2O) to give cis-7 (32.9 mg, 0.0434 mmol, 33%) and trans-7 (34.8 mg, 0.0459 mmol, 35%) as colorless solids. cis-7: Rf = 0.57 (Et2O); colorless crystals; mp 154 ºC (dec.); 1H NMR (300 MHz, 298 K, CDCl3) δ 0.04 (br s, 18H), 0.05 (br s, 18H), 0.07 (br s, 18H), 1.35 (s with satellites, 1H, 2JSiH = 5.0 Hz), 2.13 (br s, 1H), 2.15 (br s, 1H), 5.21 (s with satellites, 1H, 1JHSi = 199 Hz), 6.32 (br s, 1H), 6.43 (br s, 1H), 6.99-7.25 (m, 4H), 7.46-7.55 (m, 3H), 7.72-7.80 (m, 2H); 13C{1H} NMR (75 MHz, 298 K, CDCl3) δ 0.7 (CH3), 1.0 (CH3), 29.9 (CH), 30.3 (CH), 30.8 (CH), 119.3 (C), 122.0 (CH), 126.9 (CH), 128.7 (CH, d, JCP = 11.7 Hz), 130.8 (CH, d, JCP = 10.5 Hz), 131.8 (CH, d, JCP = 3.1 Hz), 132.8 (C, d, JCP = 105 Hz), 141.7 (CH, d, 1JCP = 99.8 Hz), 145.9 (CH, d, 3JCP = 6.2 Hz), 146.5 (C), 152.9 (C), 153.2 (C); 31P NMR (121 MHz, 298 K, CDCl3) δ 3.43 (3JPSi = 33 Hz); 29Si NMR (59 MHz, 298 K, CDCl3) δ –61.9 (d, 3JSiP = 33 Hz), 1.9, 2.3; Anal. Calcd for C37H70OPSi7: C, 58.67; H, 9.18. Found: C, 57.77; H, 8.96. trans-7: Rf = 0.30 (Et2O); colorless crystals; mp 178 ºC (dec.); 1H NMR (300 MHz, 298 K, CDCl3) δ 0.026 (br s, 18H), 0.037 (br s, 18H), 0.042 (br s, 18H), 1.35 (s, 1H), 1.92 (br s, 1H), 1.97 (br s, 1H), 5.31 (d with sateslites, 1H, 4JHP = 5.9 Hz, 1JHSi = 202 Hz), 6.31 (br s, 1H), 6.43 (br s, 1H), 6.93-7.36 (m, 4H), 7.46-7.58 (m, 3H), 7.73-7.78 (m, 2H); 13C{1H} NMR (75 MHz, 298 K, CDCl3) δ 0.67 (CH3), 0.72 (CH3), 1.0 (CH3), 30.3 (CH), 30.6 (CH), 30.9 (CH), 120.0 (C), 122.0 (CH), 126.9 (CH), 128.7 (CH, d, JCP = 12.3 Hz), 130.7 (CH, d, JCP = 10.5 Hz), 132.1 (CH, d, JCP = 2.5 Hz), 132.6 (C, d, JCP = 105.9 Hz), 141.4 (CH, d, JCP = 99.8 Hz), 146.5 (C), 149.1 (CH, d, JCP = 5.5 Hz), 152.5 (C), 152.7 (C); 31P NMR (121 MHz, 298 K, CDCl3) δ 5.65 (3JPSi = 35 Hz); 29Si NMR (59 MHz, 298 K, CDCl3) δ –61.6 (d, 3JSiP = 35 Hz), 1.9, 2.1; HRMS (FAB) m/z calcd for C37H70OPSi7 [(M+H)+]: 757.3549, found 757.3525; Anal. Calcd for C37H72O2PSi7 (+H2O): C, 57.30; H, 9.23. Found: C, 57.58; H, 9.14.
In several attempts, before adding H2O2 aq, removing the solvents and filtration through Celite with hexane were performed to give a mixture containing 5 mainly. However, separation of isomers each other from the mixture was difficult. 5 (a mixture of cis- and trans-isomers): a colorless solid; 1H NMR (300 MHz, 298 K, C6D6, only for characteristic peaks) δ 5.48 (Si–H, d, 1H, 4JHP = 17.8 Hz), 5.82 (Si–H, d with satellites, 1H, 4JHP = 3.3 Hz, 1JHSi = 192 Hz); 31P NMR (121 MHz, 298 K, C6D6) δ –25.7, –25.5; 29Si NMR (59 MHz, 298 K, C6D6) δ –61.4 (d, 3JSiP = 11.0 Hz), –57.0 (d, 3JSiP = 12.2 Hz), 2.0, 2.1, 2.3.
Synthesis of trans-6. To a THF solution (0.78 mL) of 2 (790 mg, 0.912 mmol) and tetramethylethylenediamine (0.438 mL, 2.92 mmol) was added n-BuLi (1.48 M in hexane, 2.05 mL, 3.03 mmol) at –78 ºC. After stirring for 11 h at the same temperature, Mes*PCl2 (1.580 g, 4.56 mmol) was added to the reaction mixture at –78 ºC. The reaction mixture was allowed to warm up to –30 ºC, and kept for 24 h at the same temperature. Then, the solvents were removed at –30 ºC, and the reaction mixture was put into a glovebox filled with argon. To the reaction mixture was added n-hexane, and the resulting suspension was filtered with Celite. The solvent was removed in vacuo. Fortunately, the single crystal of trans-6 was obtained from the mixture at this stage. However, the isolation of pure trans-6 in enough amount to analyze its chemical properties was difficult in spite of a number of times of recrystallization in a glovebox. Therefore, the mixture was separated by GPC (eluent: toluene) to give trans-6 (632 mg, 0.694 mmol, 76%, if pure) contaminated with some impurities. trans-6: 1H NMR (300 MHz, 298 K, C6D6) δ 0.18 (s, 18H), 0.24 (br s, 36H), 1.29 (s, 9H), 1.49 (s, 1H), 1.64 (br s, 18H), 2.60 (br s, 2H), 5.97 (br s, 1H), 6.46 (dd, J = 16.5, 22.3 Hz, 2H), 6.56 (br s, 1H), 6.66 (br s, 1H), 7.12-7.40 (m, 2H), 7.62 (s, 1H), 7.63 (s, 1H); 31P NMR (121 MHz, 298 K, C6D6) δ –24.5.
Synthesis of trans-8. To a THF solution (0.84 mL) of trans-6 (130 mg, 0.143 mmol, if pure) was added H2O2 aq (30%, 0.0438 mL, 1.43 mmol) at 0 ºC. After stirring for 12 h at room temperature, the solvents were removed in vacuo. The reaction mixture was separated by silica gel chromatography (n-hexane:Et2O = 2:1) to give trans-8 (108 mg, 0.117 mmol, 82%) as a colorless solid. trans-8: Rf = 0.60 (n-hexane:Et2O = 2:1); colorless crystals; mp 242 ºC (dec.); 1H NMR (300 MHz, 298 K, CDCl3) δ 0.03 (br s, 36H), 0.04 (br s, 18H), 1.29 (s, 9H), 1.34 (s, 1H), 1.48 (s, 18H), 1.83 (br s, 1H), 1.92 (br s, 1H), 5.36 (d, 1H, 4JHP = 6.1 Hz, satellite, 1JHSi = 211 Hz), 6.32 (br s, 1H), 6.44 (br s, 1H), 7.07 (dd, J = 41.7, 16.5 Hz, 2H), 7.34 (s, 1H), 7.35 (s, 1H), 7.49 (dd, J = 30.2, 16.5 Hz, 2H); 13C{1H} NMR (75 MHz, 298 K, CDCl3) δ 0.8 (CH3), 1.1 (CH3), 30.1 (CH), 30.3 (CH), 30.8 (CH), 31.0 (CH3), 33.8 (CH3), 34.5 (CH), 40.1 (C, d, JCP = 3.1 Hz), 121.1 (C), 122.0 (CH), 123.8 (CH, JCP = 11.3 Hz), 126.3 (C, d, JCP = 106.8 Hz), 127.1 (CH), 143.2 (CH, d, JCP = 4.2 Hz), 145.8 (C), 149.4 (CH, d, JCP = 98.6 Hz), 151.0 (C, JCP = 3.2 Hz), 152.2 (C), 152.6 (C), 159.3 (C); 31P NMR (121 MHz, 298 K, CDCl3) δ 12.6 (3JPSi = 35 Hz); 29Si NMR (59 MHz, 298 K, CDCl3) δ –62.0 (d, 3JSiP = 35 Hz), 1.9, 2.1; HRMS (APPI-TOF) m/z calcd for C49H94OPSi7 [(M+H)+]: 925.5422, found 925.5500; Anal. Calcd for C49H93OPSi7: C, 63.57; H, 10.12. Found: C, 63.60; H, 10.28.
Synthesis of 11. To a C6D6 solution (1.55 mL) of cis-7 (140 mg, 0.186 mmol) in an NMR tube (5φ) filled with argon was added trichlorosilane (0.0934 mL, 0.924 mmol) at room temperature. The NMR tube was sealed with screw-type cap, and then heated at 80 ºC. Starting material was consumed after 1.5 h, resulting in the formation of 11 almost quantitatively. Recrystallization form n-hexane at –40 ºC afforded 11 (77.0 mg, 0.0992 mmol, 53%). When using trans-7 as a starting material, the same compound was generated. 11: 1H NMR (300 MHz, 298 K, C6D6) δ 0.15 (br s, 18H), 0.24 (br s, 18H), 0.27 (br s, 18H), 1.47 (s, 1H), 2.78 (s, 2H), 6.52 (br s, 1H), 6.63 (br s, 1H), 6.77-7.15 (m, 7H), 7.40-7.45 (m, 2H); 13C{1H} NMR (75 MHz, 298 K, C6D6) δ 1.0 (CH3), 1.1 (CH3), 1.5 (CH3), 29.1 (CH), 29.4 (CH), 31.1 (CH), 122.9 (CH), 124.6 (C, d, JCP = 6.2 Hz), 127.7 (CH), 128.8 (CH, d, JCP = 8.6 Hz), 130.1 (CH), 133.4 (C, d, JCP = 4.9 Hz), 133.9 (CH, d, JCP = 5.6 Hz), 134.1 (CH, d, JCP = 21.6 Hz), 146.7 (C), 148.2 (CH, d, JCP = 16.1 Hz), 152.9 (C), 153.4 (C); 31P NMR (121 MHz, 298 K, C6D6) δ –25.2 (satellite, 3JPSi = 9 Hz); 29Si NMR (59 MHz, 298 K, C6D6) δ –26.4 (d, 3JSiP = 9 Hz), 2.3, 2.8.
Reaction of 11 with lithium aluminum hydride. To a THF solution (0.65 mL) of 11 (25.4 mg, 0.0327 mmol) was added lithium aluminum hydride (1.7 mg, 0.045 mmol) at 0 ºC. The reaction mixture was warmed up to room temperature. After stirring for 1 h, the solvent was removed, and the reaction mixture was put into a glovebox filled with argon. To the reaction mixture was added n-hexane, and the resulting suspension was filtered with Celite to afford almost pure 5 (a mixture of isomers, 24.5 mg, 0.0330 mmol, if pure).
Reaction of 5 with Ph3C+TPFPB–. In a glovebox filled with argon, C6D6 (0.60 mL) was added to the mixture of 5 (a mixture of isomers, 17.8 mg, 0.0240 mmol) and Ph3C+TPFPB– (22.1 mg, 0.0240 mmol) in an NMR tube (5φ) at room temperature. The NMR tube was sealed with screw-type cap. Starting material was almost consumed after heating at 80 ºC for 51 h. Although the generation of Ph3CH was observed, the 29Si and 31P NMR spectra were complicated (δP: –15.2, –15.0, 6.7, 7.0; δSi: –62.0, –61.9, –61.4, –61.3).
Reaction of 6 with Ph3C+TPFPB–. In a glovebox filled with argon, C6H6 (1.90 mL) solution of 6 (60.6 mg, 0.0666 mmol) was added to Ph3C+TPFPB– (61.4 mg, 0.0666 mmol) in a glass tube (10φ) at room temperature. The NMR tube was degassed and sealed. During heating at 70 ºC for 15 h, the reaction mixture was separated to two phases. The tube was opened in a glovebox, and the solvent was removed. Although the generation of Ph3CH was observed, many signals were observed around –44 and –23 ppm in the 31P NMR. In addition to these signals, characteristic peak was observed at +221.4 ppm. Unfortunately, we could not determine the structure of the corresponding compound.
Reaction of 11 with Ag+TPFPB–. In a glovebox filled with argon, a C6D6 solution (0.77 mL) of 11 (30.0 mg, 0.0387 mmol) was added to Ag+TPFPB– (39.5 mg, 0.0387 mmol) in an NMR tube (5φ) at room temperature. The NMR tube was sealed with screw-type cap. Starting material was consumed after 1 h, resulting in the complicated mixture (δP = 6.5, 8.2, and many signals appeared between –19 and –26 ppm).
Reaction of 5 with lithium dispersion. In a glovebox filled with argon, lithium dispersion (3.6 mg, 0.52 mmol) was added to a THF solution (0.64 mL) of 5 (a mixture of isomers, 38 mg, 0.051 mmol) in an NMR tube (5φ) at room temperature. The NMR tube was sealed with screw-type cap. Starting material (δP = –25.1, –25.3 in THF) was consumed after 3 h, resulting in the quantitative formation of novel species (δP = –17.4 in THF). The non-decoupling 31P NMR showed tt pattern (J = 44.3, 11.7 Hz), suggesting the retain of the –C(H)=C(H)–P–C(H)=C(H)– moiety. The 1H NMR signals in 5-8 ppm were observed at 5.69 (dd, J = 15.9, 11.7 Hz, 2H), 6.49 (s, 1H, Si–H), 6.58 (br s, 1H, m-Tbt), 6.67 (br s, 1H, m-Tbt), 7.73 (dd, J = 44.3, 15.9 Hz, 2H) ppm. The detachment of the phenyl group and formation of P–Li species are most reasonable. However, this species decomposed by condensation, and several attempts of trapping reaction by MeOH or benzyl chloride afforded a complicated mixture.
General procedure for X-ray crystallographic analysis of compounds 2, trans-6, [trans-7·H2O], cis-7, and [trans-8·2.25CHCl3]. The intensity data were collected on a Rigaku/MSC Mercury CCD diffractometer with graphite monochromated MoKα radiation (λ = 0.71070 Å). A single crystal suitable for X-ray analysis was mounted on a glass fiber. The structures were solved by a direct method (SHELXS-97)21 and refined by full-matrix least-squares procedures on F2 for all reflections (SHELXL-97).21 All hydrogen atoms except for Si–H were placed using AFIX instructions, while all other atoms were refined anisotropically. Deposition numbers CCDC-898201 (2), 898202 (trans-6), 898205 (trans-7·H2O), 898204 (cis-7), and 898203 (trans-8·2.25CHCl3). Free copies of the data can be obtained via http://www.ccdc.cam.ac.uk/conts/retrieving.html (or from the Cambridge Crystallographic Data Centre, 12 Union Road, Cambridge, CB2 1EZ, UK; Fax: +44 1223 336033; e-mail: deposit@ccdc.cam.ac.uk).
ACKNOWLEDGEMENTS
This work was supported by Grants-in-Aid for Creative Scientific Research (No. 17GS0207), Scientific Research (B) (No. 22350017), Scientific Research on Innovative Areas (No. 23108707, “π-Space”), Young Scientist (B) (No. 21750042), and the Global COE Program (B09, “Integrated Materials Science”) from the Ministry of Education, Culture, Sports, Science and Technology, of Japan. Y.M. thanks Mitsubishi Chemical Corp., the Society of Synthetic Organic Chemistry, Japan, and Kinki Invention Center, for financial support.
References
1. M. Haaf, T. A. Schmedake, and R. West, Acc. Chem. Res., 2000, 33, 704; CrossRef V. Y. Lee, A. Sekiguchi, M. Ichinohe, and N. Fukaya, J. Organomet. Chem., 2000, 611, 228; CrossRef A. Sekiguchi and V. Y. Lee, Chem. Rev., 2003, 103, 1429; CrossRef V. Y. Lee and A. Sekiguchi, Angew. Chem. Int. Ed., 2007, 46, 6596; CrossRef V. Y. Lee and A. Sekiguchi, Organometallic Compounds of Low-Coordinate Si, Ge, Sn and Pb: From Phantom Species to Stable Compounds; Wiley, 2010; Chapter 6. CrossRef
2. N. Tokitoh, Acc. Chem. Res., 2004, 37, 86. CrossRef
3. A. Sekiguchi, M. Tsukamoto, and M. Ichinohe, Science, 1997, 275, 60; CrossRef S. B. Choi, P. Boudjouk, and P. R. Wei, J. Am. Chem. Soc., 1998, 120, 5814; CrossRef T. Nishinaga, Y. Izukawa, and K. Komatsu, J. Am. Chem. Soc., 2000, 122, 9312; CrossRef M. Saito, R. Haga, and M. Yoshioka, Chem. Commun., 2002, 1002; CrossRef M. Saito, R. Haga, and M. Yoshioka, Chem. Lett., 2003, 32, 912; CrossRef M. Saito, R. Haga, M. Yoshioka, K. Ishimura, and S. Nagase, Angew. Chem. Int. Ed., 2005, 44, 6553; CrossRef M. Ichinohe, M. Igarashi, K. Sanuki, and A. Sekiguchi, J. Am. Chem. Soc., 2005, 127, 9978; CrossRef V. Y. Lee, R. Kato, M. Ichinohe, and A. Sekiguchi, J. Am. Chem. Soc., 2005, 127, 13142; CrossRef S. Ishida, T. Nishinaga, R. West, and K. Komatsu, Chem. Commun., 2005, 778; CrossRef M. Driess, S. Yao, M. Brym, and V.-C. Wullen, Angew. Chem. Int. Ed., 2006, 45, 6730; CrossRef R. Kinjo, M. Ichinohe, A. Sekiguchi, N. Takagi, M. Sumimoto, and S. Nagase, J. Am. Chem. Soc., 2007, 129, 7766; CrossRef M. Igarashi, M. Ichinohe, and A. Sekiguchi, J. Am. Chem. Soc., 2007, 129, 12660; CrossRef M. Saito, M. Sakaguchi, T. Tajima, K. Ishimura, S. Nagase, and M. Hada, Science, 2010, 328, 339. CrossRef
4. K. Wakita, N. Tokitoh, R. Okazaki, and S. Nagase, Angew. Chem. Int. Ed., 2000, 39, 634; CrossRef K. Wakita, N. Tokitoh, R. Okazaki, N. Takagi, and S. Nagase, J. Am. Chem. Soc., 2000, 122, 5648; CrossRef A. Shinohara, N. Takeda, T. Sasamori, T. Matsumoto, and N. Tokitoh, Organometallics, 2005, 24, 6141; CrossRef N. Tokitoh, K. Wakita, T. Matsumoto, T. Sasamori, R. Okazaki, N. Takagi, M. Kimura, and S. Nagase, J. Chin. Chem. Soc., 2008, 55, 487.
5. N. Nakata, N. Takeda, and N. Tokitoh, J. Am. Chem. Soc., 2002, 124, 6914; CrossRef N. Nakata, N. Takeda, and N. Tokitoh, J. Organomet. Chem., 2003, 672, 66; CrossRef N. Nakata, N. Takeda, and N. Tokitoh, Angew. Chem. Int. Ed., 2003, 42, 115. CrossRef
6. Y. Mizuhata, N. Noda, and N. Tokitoh, Organometallics, 2010, 29, 4781. CrossRef
7. N. Tokitoh, K. Wakita, R. Okazaki, S. Nagase, P. v. R. Schleyer, and H. Jiao, J. Am. Chem. Soc., 1997, 119, 6951; CrossRef K. Wakita, N. Tokitoh, R. Okazaki, S. Nagase, P. v. R. Schleyer, and H. Jiao, J. Am. Chem. Soc., 1999, 121, 11336; CrossRef K. Wakita, N. Tokitoh, and R. Okazaki, Bull. Chem. Soc. Jpn., 2000, 73, 2157. CrossRef
8. N. Takeda, A. Shinohara, and N. Tokitoh, Organometallics, 2002, 21, 4024; CrossRef A. Shinohara, N. Takeda, T. Sasamori, and N. Tokitoh, Bull. Chem. Soc. Jpn., 2005, 78, 977. CrossRef
9. N. Nakata, N. Takeda, and N. Tokitoh, Organometallics, 2001, 20, 5507; CrossRef N. Nakata, N. Takeda, and N. Tokitoh, Chem. Lett., 2002, 818; CrossRef N. Nakata, N. Takeda, and N. Tokitoh, Organometallics, 2003, 22, 481. CrossRef
10. Y. Mizuhata, T. Sasamori, N. Takeda, and N. Tokitoh, J. Am. Chem. Soc., 2006, 128, 1050; CrossRef Y. Mizuhata, T. Sasamori, N. Nagahora, Y. Watanabe, Y. Furukawa, and N. Tokitoh, Dalton Trans., 2008, 4409. CrossRef
11. N. Takeda, A. Shinohara, and N. Tokitoh, Organometallics, 2002, 21, 256; CrossRef A. Shinohara, N. Takeda, and N. Tokitoh, J. Am. Chem. Soc., 2003, 125, 10804. CrossRef
12. T. Sasamori, K. Inamura, W. Hoshino, N. Nakata, Y. Mizuhata, Y. Watanabe, Y. Furukawa, and N. Tokitoh, Organometallics, 2006, 25, 3533. CrossRef
13. N. Tokitoh, A. Shinohara, T. Matsumoto, T. Sasamori, N. Takeda, and Y. Furukawa, Organometallics, 2007, 26, 4048. CrossRef
14. Y. Mizuhata, N. Takeda, T. Sasamori, and N. Tokitoh, Chem. Lett., 2005, 128, 1088. CrossRef
15. a) Y. Ren and T. Baumgartner, Chem. Asian J., 2010, 5, 1918; CrossRef b) Y. Ren, T. Linder, and T. Baumgartner, Can. J. Chem., 2009, 87, 1222; CrossRef c) H. Tsuji, S. Komatsu, Y. Kanda, T. Umehara, T. Saeki, and K. Tamao, Chem. Lett., 2006, 35, 758; CrossRef d) N. K. Skvortsov and S. V. Toldov, Russ. J. General Chem., 1994, 64, 1583; e) A. Ishii, T. Tsuchiya, J. Nakayama, and M. Hoshino, Tetrahedron Lett., 1993, 34, 2347. CrossRef
16. G. Märkl, H. Baier, and R. Liebl, Synthesis, 1977, 842. CrossRef
17. a) A. J. Ashe, T. R. Diephouse, and M. Y. El-Sheikh, J. Am. Chem. Soc., 1982, 104, 5693; CrossRef b) G. Märkl and F. Kneidl, Angew. Chem., 1974, 86, 745; CrossRef c) A. J. Ashe and M. D. Gordon, J. Am. Chem. Soc., 1972, 94, 7596; CrossRef d) A. J. Ashe, J. Am. Chem. Soc., 1971, 93, 6690. CrossRef
18. G. Märkl, H. Baier, R. Liebl, and D. S. Stephenson, Liebigs Ann. Chem., 1981, 870; CrossRef G. Märkl, H. Baier, and R. Liebl, Liebigs Ann. Chem., 1981, 919. CrossRef
19. A. B. Pangborn, M. A. Giardello, R. H. Grubbs, R. K. Rosen, and F. J. Timmers, Organometallics, 1996, 15, 1518. CrossRef
20. Gaussian 09, Revision A.02, M. J. Frisch, G. W. Trucks, H. B. Schlegel, G. E. Scuseria, M. A. Robb, J. R. Cheeseman, G. Scalmani, V. Barone, B. Mennucci, G. A. Petersson, H. Nakatsuji, M. Caricato, X. Li, H. P. Hratchian, A. F. Izmaylov, J. Bloino, G. Zheng, J. L. Sonnenberg, M. Hada, M. Ehara, K. Toyota, R. Fukuda, J. Hasegawa, M. Ishida, T. Nakajima, Y. Honda, O. Kitao, H. Nakai, T. Vreven, J. A. Montgomery, Jr., J. E. Peralta, F. Ogliaro, M. Bearpark, J. J. Heyd, E. Brothers, K. N. Kudin, V. N. Staroverov, R. Kobayashi, J. Normand, K. Raghavachari, A. Rendell, J. C. Burant, S. S. Iyengar, J. Tomasi, M. Cossi, N. Rega, J. M. Millam, M. Klene, J. E. Knox, J. B. Cross, V. Bakken, C. Adamo, J. Jaramillo, R. Gomperts, R. E. Stratmann, O. Yazyev, A. J. Austin, R. Cammi, C. Pomelli, J. W. Ochterski, R. L. Martin, K. Morokuma, V. G. Zakrzewski, G. A. Voth, P. Salvador, J. J. Dannenberg, S. Dapprich, A. D. Daniels, O. Farkas, J. B. Foresman, J. V. Ortiz, J. Cioslowski, and D. J. Fox, Gaussian, Inc., Wallingford CT, 2009.
21. G. M. Sheldrick, Acta Cryst., 2008, A64, 112. CrossRef