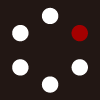
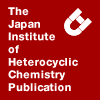
HETEROCYCLES
An International Journal for Reviews and Communications in Heterocyclic ChemistryWeb Edition ISSN: 1881-0942
Published online by The Japan Institute of Heterocyclic Chemistry
e-Journal
Full Text HTML
Received, 10th September, 2012, Accepted, 5th October, 2012, Published online, 12th October, 2012.
DOI: 10.3987/COM-12-12583
■ The New Convenient Synthesis of 6-Fluoropurine and Its 7-/9-Unsubstituted Analogues
Mei Deng, Ning-Yu Wang, Guangming Xiang, and Luo-Ting Yu*
State Key Laboratory of Biotherapy, West China Hospital, West China Medical School, Sichuan University, Chengdu 610041, China
Abstract
6-Fluoropurine and its 7-/9-unsubstituted analogues have good biological activity and serve as important pharmaceutical intermediates. This paper describes a new and convenient synthesis of 6-fluoropurine and its 7-/9-unsubstituted analogues, by first replacing the chlorine atoms with trimethylammonio groups through the reaction of 6-chloropurine and its 7-/9-unsubstituted analogues with trimethylamine, and then replacing the trimethylammonio groups with fluorine atoms using safe and cheap TBAF·3H2O as fluorinating agent at room temperature. Compared with reported methods, the new synthesis has milder conditions, shorter reaction times, simpler post-processing and higher (or similar) yields.6-Fluoropurine and its 7-/9-unsubstituted analogues have good biological activity and serve as important pharmaceutical intermediates.1,2 Purines are integral parts of DNA and RNA. Purine analogues are a very important class of nucleoside pharmaceutical intermediates and have special effect on cancer, AIDS, leukemia and others.3-9 Fluorine-substituted compounds such as Fludarabine, show remarkable differences in biological activities and pharmacological properties compared to their parent molecules, as introduction of fluorine can improve the metabolic stability, modulate the physicochemical properties and increase binding affinity to a target protein.10,11
According to the literature,12-16 6-fluoropurine and its 7-/9-unsubstituted analogues can be obtained by the following methods: (i) 6-chloropurine and its 7-/9-unsubstituted analogues react with dry potassium fluoride in dry DMSO at 180 ˚C using tetraphenylphosphonium bromide as phase transfer catalyst; (ii) 6-chloropurine and its 7-/9-unsubstituted analogues react with dry potassium fluoride under microwave using ionic liquid as solvent; (iii) a modified Schiemann Reaction on an aminopurine in which the diazotization product is treated with fluoroboric acid; (iv) displacement of trimethylammonio groups formed by the reaction of 6-chloropurine and its 7-/9-unsubstituted analogues with trimethylamine, on treatment with hydrogen potassium fluoride; (v) fluorination of 6-chloropurine and its 7-/9-unsubstituted analogues using "anhydrous" tetrabutylammonium fluoride (TBAFanh) in anhydrous DMSO at 20 ˚C.
But all the five methods have disadvantages: methods (i) and (ii) need high temperatures, and ionic liquid used in method (ii) is hard to get; method (iii) gives low yields and needs harsh reaction conditions; method (iv) uses hydrogen potassium fluoride which is corrosive, giving poor yields of products (6-fluoropurine, 35% and 2-chloro-6-fluoropurine, 32%) and considerable amounts of byproducts, and sometimes the reaction should be heated (6-fluoropurine, 60 ˚C); method (v) requires long reaction times (6-fluoropurine, 14 days) ending up with low yields (6-fluoropurine, 65%), and high vacuum (<0.1 mmHg) demanded in the preparation of TBAFanh is difficulty to achieve.
In order to find a new convenient synthesis of 6-fluoropurine and its 7-/9-unsubstituted analogues, we first analyzed the causes leading to the long reaction times and low yields in method (v) which has the mildest reaction conditions in the five methods. It was found that TBAFanh behaves not only as a good source of nucleophilic fluoride but also a strong base and hydrolyzing agent,17 and it deprotonates 6-fluoropurine and its 7- or 9-unsubstituted analogues, generating an equivalent of bifluoride ion16 and resulting in some degree of deactivation of the reactants.
It was reported that safe, commercially available and cheap trihydrate tetrabutylammonium fluoride (TBAF·3H2O) is a powerful, nonbasic and nucleophilic fluorinating agent,17 but it could not fluorinate 6-chloropurine and its 7-/9-unsubstituted analogues (1a-1c), because of its weaker nucleophilicity of fluoride ion than that in TBAFanh. To solve the problem, we first replaced the chlorine atoms with trimethylammonio groups which are better leaving groups, through the reaction of 6-chloropurine and its 7-/9-unsubstituted analogues (1a-1c) with aqueous solution of trimethylamine15,18 in high yields (see Table 1), and then replaced the trimethylammonio groups with fluorine atoms using TBAF·3H2O as fluorinating agent (see Table 2).
In order to find the optimized reaction conditions we first studied the preparation of 6-fluoropurine 3a from 2a. The results showed that 3a was produced in a good yield of 71.3% with no byproduct within 7 days using 3 equivalents of TBAF·3H2O as fluorinating agent in DMSO at room temperature (Entry 10). And the overall yield of 3a (64.2%, Entry 10) was similar with that in method (v) (65%) but with a shorter reaction time (7.5 days vs 14 days) and less fluorinating agent (3 eq vs 4 eq).16 In fact, our method will save more time. Because the preparation of TBAFanh needs 2 days under high vacuum (<0.1 mmHg)16 which is hard to achieve, whereas TBAF·3H2O is commercially available and cheap. When the reaction time prolonged to 14 days (Entry 11), the yield of 3a increased to 80.0% and 3.8% byproduct was generated,probably by the hydrolysis of 3a. 3a was achieved in a better yield in DMSO than in DMF, THF and EtOH (Entries 2 and 4-6), and in a higher yield with TBAF·3H2O than with KF·2H2O (Entries 2 and 8), because of the good solubility of TBAF·3H2O in DMSO. The reaction in EtOH (Entry 6) gave a significant amount of byproduct, maybe because of EtOH also reacting with 2a. When the temperature raised up to 50 ˚C (Entry 7), the reaction afforded 3a in a reduced yield and byproduct in a considerable yield. And it was probably because of that 2a started to decompose, water reacted with 2a and the hydrolysis of 3a was enhanced at 50 ˚C.
Then, we investigated the preparation of its 7-/9-unsubstituted analogues 3b and 3c, respectively. Compared with 3a in Entry 10, 3b and 3c (Entries 12 and 13) were produced in better yields (90.0% and 91.0%), together with no byproducts within shorter reaction time (0.5 day) under the same reaction conditions, probably due to that the R = Cl in 2b and X = C in 2c (Table 2) increased the electrophilicity of the carbon atoms connecting to the trimethylammonio groups. We verified our point by the preparation of 6-fluoro-9H-purin-2-amine from 6-chloro-9H-purin-2-amine (Table 1, R = NH2, X = N) through our route, ending up with a high yield of intermediate and no product. The overall yields of 3b and 3c in our report (Entries 12 and 13) were remarkably higher than reported. Because Entries 10, 12 and 13 produced no byproducts, the post-processing was simpler.
In conclusion, this paper described a new and convenient syntheses of 6-fluoropurine and its 7-/9-unsubstituted analogues, in which we first replaced the chlorine atoms with trimethylammonio groups which are better leaving groups, through the reaction of 6-chloropurine and its 7-/9-unsubstituted analogues with aqueous solution of trimethylamine, and then replaced the trimethylammonio groups with fluorine atoms using safe, commercially available and cheap TBAF·3H2O as fluorinating agent in DMSO at room temperature. The new method has advantages of milder conditions (compared with methods (i)-(v)), shorter reaction times (compared with method (v)), simpler post-processing (compared with methods (i)-(v)) and similar to higher yields (compared with methods (i)-(v)) compared with the reported methods.
EXPERIMENTAL
The melting points were obtained on a SGW X-4 microscopic melting point apparatus and were uncorrected. IR spectra were determined with a Bruker Tensor 27 spectrophotometer. The 1H and 13C NMR spectra were determined in DMSO-d6 using TMS as an internal reference with a Bruker AscendTM 400 NMR spectrometer operating at 400 MHz and 100 MHz, respectively. Low-resolution MS spectra (ESI) were measured by a Waters Quattro Premier XE spectrometer. High-resolution MS spectra (ESI) were measured by a Waters Q-TOF Premier spectrometer. TLC was carried out on TLC silica gel 60 F254 plates. Column chromatography was performed using silica gel (300-400 mesh, Qingdao Marine Chemical Ltd., Qingdao, China). HPLC was carried out on Agela Technologies LC-10F. All the commercially available reagents were used without further purification.
General Procedure for the Preparation of 2. To a solution of 1 (10 mmol) in THF (3 mL) at rt was slowly added 33% trimethylamine water solution (~3 mL, 30 mmol). When TLC (eluent: EtOAc) monitoring indicated that the reaction was completed (ca. 0.5 day) the crystalline precipitate was washed with ether and recrystallized from aqueous acetone (rt) giving 2.
N,N,N-Trimethyl-9H-purin-6-aminium chloride (2a): a pale-yellow solid; yield 90%; mp 201.5-202.5 ˚C (decomp.) (lit.,15 191-193 ˚C (decomp.)); IR (KBr) 1617, 1578 cm-1; 1H NMR δ 14.70 (s, 1H), 9.06 (s, 1H), 8.97 (s, 1H), 3.84 (s, 9H); 13C NMR δ 156.6, 151.5, 150.4, 147.5, 123.3, 54.2; MS (ESI) m/z 178 [M-Cl]+. HRMS (ESI) Calcd for C8H12N5 [M-Cl]+: 178.1093; Found: 178.1092.
2-Chloro-N,N,N-trimethyl-9H-purin-6-aminium chloride (2b): a pale-yellow solid; yield 90%; mp > 300 ˚C (lit.,15 > 300 ˚C); IR (KBr) 1612, 1561 cm-1; 1H NMR δ 14.88 (s, 1H), 8.98 (s, 1H), 3.81 (s, 9H); 13C NMR δ 154.5, 152.3, 152.1, 138.4, 117.9, 54.4; MS (ESI) m/z 212 [M-Cl]+. HRMS (ESI) Calcd for C8H11N5Cl [M-Cl]+: 212.0703; Found: 212.0702.
N,N,N-Trimethyl-7H-pyrrolo[2,3-d]pyrimidin-4-aminium chloride (2c): a white solid; yield 90%, mp 191.2-192.2 ˚C; IR (KBr) 1604, 1565 cm-1; 1H NMR δ 13.21 (s, 1H), 8.89 (s, 1H), 8.00 (d, 1H, J = 3.4 Hz), 7.22 (d, 1H, J =3.4 Hz), 3.76 (s, 9H); 13C NMR δ 155.1, 154.7, 148.7, 130.4, 107.2, 99.6, 54.2. MS (ESI) m/z 177 [M-Cl]+. HRMS (ESI) Calcd for C9H13N4 [M-Cl]+: 177.1140; Found: 177.1141.
General Procedure for the Preparation of 3. As indicated in Table 2, to a solution of 2 (1.2 mmol) in solvent (3 mL) at the temperature was added fluorinating agent. The reaction was maintained at that temperature for the time indicated in Table 2 and was monitored by HPLC. When the reaction was terminated, add water (30 mL) into the reaction mixture and extract it with EtOAc five times (10 mL each). The combined extracts were washed with water and brine and dried over anhydrous magnesium sulfate. After filtration, the solvent was removed in vacuum. In Entries 6, 7, 8 and 11, the residue was purified by silica gel column chromatography.
6-Fluoro-9H-purine (3a): a white solid; mp 125.5-126.6 ˚C (lit.,13 122-124 ˚C); IR (KBr) 1634, 1578 cm-1; 1H NMR δ 13.98 (s, 1H), 8.68 (s, 1H), 8.65 (s, 1H); 13C NMR δ 158.4 (J = 214.7 Hz), 151.1, 151.0, 145.7, 118.7; MS (ESI) m/z 137 [M-H]-. HRMS (ESI) Calcd for C5H4N4F [M+H]+: 139.0420; Found: 139.0422.
2-Chloro-6-fluoro-9H-purine (3b): a white solid; mp 146.0-148.2 ˚C (lit.,15 146 ˚C); IR (KBr) 1656, 1634, 1565 cm-1; 1H NMR δ 14.01 (s, 1H), 8.72 (s, 1H); 13C NMR δ 157.8 (J = 207.1 Hz), 149.9, 149.8, 146.9, 117.8; MS (ESI) m/z 171 [M-H]-. HRMS (ESI) Calcd for C5H3N4FCl [M+H]+: 173.0030; Found: 173.0029.
4-Fluoro-7H-pyrrolo[2,3-d]pyrimidine (3c): a white solid; mp 172.8-173.8 ˚C; IR (KBr) 1629, 1594, 1576 cm-1; 1H NMR δ 12.61 (s, 1H), 8.49 (s, 1H), 7.66 (d, 1H, J = 3.0 Hz), 6.66 (d, 1H, J = 3.0 Hz). 13C NMR δ 161.4 (J = 197.8 Hz), 155.5 (J = 9.9 Hz), 149.8 (J = 11.1 Hz), 127.5, 103.1 (J = 26.2 Hz), 96.9 (J = 4.1 Hz); MS (ESI) m/z 136 [M-H]-. HRMS (ESI) Calcd for C6H5N3F [M+H]+: 138.0468; Found: 138.0465.
ACKNOWLEDGEMENT
This work was supported by National Science and Technology Major Project of China (2012ZX09103101079). We thank Mr Ming-Hai Tang of this lab for determining both low- and high-resolution mass spectra and thank Analytical & Testing Center of Sichuan University for determining infrared spectroscopy.
References
1. J.-Q. Liu and M. J. Robins, J. Am. Chem. Soc., 2007, 129, 5962. CrossRef
2. T. Shirasaka, K. M. Happy-Ford, and J. Kelly, Proc. Natl. Acad. Sci. U.S.A., 1990, 87, 9426. CrossRef
3. A. Van-Aerschot, M. Petros, and A. Piet, J. Med. Chem., 1993, 36, 2938. CrossRef
4. K. G. Estep and K. A. Josef, J. Med. Chem., 1995, 38, 2582. CrossRef
5. X.-Y. Luo, H. X. S. J., 2001, 23, 184.
6. J. Xu, Chinese J. New Drugs, 1992, 1, 63.
7. L. Zhang, Y.-Q. Chen, G.-Q. Qian, H.-H. Wu, L.-Y. Dai, and L.-P. Yang, Chinese J. Pharm., 1999, 30, 93.
8. A. Graul and P. A. Leeson, Drugs Fut., 1998, 23, 1155. CrossRef
9. Farina and Paolo, EP: 1464708A1.
10. H. J. Böhm, D. Banner, S. Bendels, M. Kansy, B. Kuhn, K. Müler, U. Obst-Sander, and M. Stahl, Chem. Biol. Chem., 2004, 5, 637. CrossRef
11. W. R. Dolbier Jr., J. Fluorine Chem., 2005, 126, 157. CrossRef
12. H.-F. Lu, G.-F. Han, and M. Lu, Chinese J. Org. Chem., 2008, 28, 1462.
13. S.-H. Xu and P. Yao, H. X. S. J., 2010, 32, 862.
14. J. A. Montgomery and K. Hewson, J. Org. Chem., 1969, 34, 1396. CrossRef
15. J. Kiburis and J. H. Lister, J. Chem. Soc. (C), 1971, 3942. CrossRef
16. H.-R. Sun and S. G. DiMagno, Angew. Chem. Int. Ed., 2006, 45, 2720. CrossRef
17. D. Albanese, D. Landini, and M. Penso, J. Org. Chem., 1998, 63, 9587. CrossRef
18. H.-C. Cai, D.-Z. Yin, L. Zhang, and Y.-X. Wang, J. Fluorine Chem., 2006, 127, 837. CrossRef