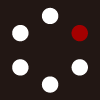
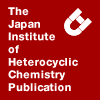
HETEROCYCLES
An International Journal for Reviews and Communications in Heterocyclic ChemistryWeb Edition ISSN: 1881-0942
Published online by The Japan Institute of Heterocyclic Chemistry
e-Journal
Full Text HTML
Received, 11th April, 2013, Accepted, 26th April, 2013, Published online, 14th May, 2013.
DOI: 10.3987/REV-13-769
■ SYNTHESIS AND CHARACTERIZATION OF TRIPHYRINS: A NEW FAMILY OF PORPHYRINOIDS
Daiki Kuzuhara and Hiroko Yamada*
Graduate School of Materials Science, Nara Institute of Science and Technology, 8916-5 Takayama, Ikoma, Nara 630-0192, Japan
Abstract
This article reviews the recent reports about the syntheses, structures, photophysical and redox properties of newly synthesized [14]triphyrins(2.1.1), their metal complexes and their core-modified triphyrins, following the overview of the other triphyrin relatives containing three pyrrole rings and more than three methine carbons. Free-base [14]triphyrins(2.1.1) work as tridentate monovalent anionic cyclic ligands and a variety of metal complexes with Re(I), Mn(I), Ru(II), Pd(II), Pd(IV), Fe(II), Fe(III) and B(III) ions were obtained due to the flexible structure of the triphyrin macrocycles. The core-modified [14]triphyrin(2.1.1) was also expected, but the alkoxy-substituted compounds were obtained instead of the desired thiatriphyrin, probably because of the larger size of sulfur atom compared to nitrogen atom and the electronic repulsion between the lone pairs of inner nitrogen atoms.CONTENTS
1. Introduction
1-1 Corroles and [14]triphyrins(1.1.1)
2. Synthesis and properties of ring-contracted porphyrins and their metal complexes
2-1 Subpyriporphyrins
2-2 [18]Triphyrins(6.1.1)
2-3 [18]Triphyrins(4.1.1)
2-4 [15]Triphyrins(3.1.1)
3. Synthesis and characterization of free-base [14]triphyrins(2.1.1)
3-1 Synthesis and statistic properties
3-2 Spectroscopic properties
4. Synthesis and characterization of metal complexes of [14]triphyrins(2.1.1)
4-1 Octahedral coordinated manganese(I), rhenium(I) and ruthenium(II) complexes
4-2 Platinum(II) and platinum(IV) complexes
4-3 Iron(II) and iron(III) complexes
4-4 Tetrahedral coordinated boron(III) complexes
5. Core-modified triphyrins
5-1 Thiatriphyrin(2.1.1) derivatives
6. Conclusion
1. INTRODUCTION
1-1 CORROLES AND [14]TRIPHYRINS(1.1.1)
Ring-contracted porphyrins are a series of porphyrinoids, which lack meso-carbon atoms or one of four pyrroles. Corroles lack one methine carbon from porphyrins and have one direct pyrrole-pyrrole link (Figure 1).1 They are known as important ligands that stabilize high valence metal ions. In addition, norcorrole has been reported to lack two methine carbons from the parent porphyrin (Figure 1). Norcorroles have two direct pyrrole-pyrrole links and the nickel complex showed the smallest antiaromatic character with 16π-electrons.2
On the other hands, triphyrin is a subclass of modified porphyrins described by a general formula [N]triphyrin(x.y.z) (N = sum of macrocyclic π-electrons; x, y, z ≥ 1: numbers of linker atoms between pyrroles) and consists of only three pyrrole or pyrrole related heterocycles. There have been a lot of reports about ring-expanded porphyrinoids3 to date, but the chemistry of the ring-contracted porphyrinoids is relatively new. First triphyrin-related compound was subphthalocyanine reported in 1972,4 then the next relative, subporphyrazine, was only reported in 2005.5
In 2006 Osuka et al. reported the synthesis of tribenzosubporphyrins ([14]benzotriphyrins(1.1.1)).6 Subsequently, Kobayashi, Osuka, and co-workers independently developed synthetic protocols for meso−aryl substituted subporphyrins.7,8 Interestingly subphthalocyanines and subporphyrins have a boron atom in the cavity of the divalent-tridentate cyclic structures with a monoanionic axial ligand. The X-ray single crystal analysis gave the bowl-shaped structure and the boron atom could never be removed. Only recently a planar-shaped subporphyrin borenium cation was obtained as a carborane anion salt.9 In contrast to the porphyrins and phthalocyanines, the electronic structure of these molecules is based on a 14π−electron system, since there are three, rather than four, pyrrole or isoindoline moieties. These subporphyrins have strong fluorescence properties and the emission can be tuned by the meso-substituents effectively. In recent years considerable interest has appeared in subphthalocyanines and subporphyrins due to the potential applications in a variety of high technology fields as non-linear optical absorption, highly fluorescent compounds, organic light emitting diodes (OLED) and organic solar cells (OSC). Several good reviews about [14]triphyrins(1.1.1) (subporphyrins and subphthalocyanines) have been already published.10 Therefore, recent progress of chemistry about subpyriporphyrins, [18]triphyrins and [14]triphyrins(2.1.1) will be focused in this review.
2. SYNTHESIS AND PROPERTIES OF RING-CONTRACTED PORPHYRINS AND THEIR METAL COMPLEXES
2-1 SUBPYRIPORPHYRINS
In 2006, subpyriporphyrin was reported as a free-base triphyrin homologue.11 It had one pyridine ring instead of one of three pyrrole rings and showed a non-aromatic electronic structure at free-base form. The synthetic route of subpyriporphyrins is shown in Scheme 1. Pyridine-included tripyrrane 2 was prepared from 2,6-bis[hydroxy-(mesityl)-methyl]pyridine 1 in 38% yield. Monobenzoylation of 2 afforded 3a (R = H) in 42% yield. Subsequently, reduction of 3a with NaBH4 gave alcohol derivative, followed by intramolecular condensation reaction to produce subpyriporphyrin 4a in 6% yield as green solids. The 11-p-nitrophenyl-substituted subpyriporphyrin 4b was also reported from the same synthetic route (Scheme 1).12
The 1H NMR spectrum of 4a showed the extreme downfield shift of NH resonance (18.07 ppm in CD2Cl2, 203 K) resulted from the strong hydrogen bonding interaction. The X-ray crystal structure of 4a showed the slightly ruffled structure. The inner proton laid in three-centered strong hydrogen bonding with N-N distances of 2.370(2) and 2.534(2) Å. The peripheral substituent could be changed from phenyl group to p-nitrophenyl group, then the absorption spectrum arising from charge-transfer (CT) transition was observed.12 Furthermore, the NH-tautomerization was a key factor determining the excited state dynamics: at 293 K the fluorescence lifetime was 8 ps due to the excited state proton transfer from pyrrole-nitrogen to pyridine-nitrogen, but at 77 K in 2-methyltetrahydrofuran, below its melting-point, the tautomerization was locked and the excited state lifetime was long as 458 ps.
The reaction of subpyriporphyrin 4a with PhBCl2 gave dome shaped σ-phenyl boron (III) triphyrin complex 5a, wherein the macrocycle acted as a monoanionic tridentate ligand.11 The 1H NMR resonances of the boron complex showed marked downfield shifts in comparison with those of subpyriporphyrin 4a and the signal of ortho-protons of B-phenyl was observed at 4.87 ppm, revealing a significant contribution by the ring current. When ethoxide ion was added to the boron complex, ethoxide was inserted to the m-position of pyridine moiety as a mixture of two aromatic stereoisomers.
2-2 [18]TRIPHYRINS(6.1.1)
In 2002, Latos-Grażyński et al. have reported 21-vacataporphyrin (8-H2; [18]triphyrin(6.1.1)), which was prepared by acid treatment of telluraporphyrin 7 to remove Te atom (Scheme 2).13 It has a butadiene linker due to a lack of the nitrogen atom from the parent porphyrin. The signals of butadiene parts were observed at -2.50 ppm (inside) and 9.65 ppm (outside), which clearly showed the nature of the 18π-electron macrocyclic aromaticity. The free-base compound 8-H2 showed nearly flat structure from X-ray single crystal diffraction and the spectroscopic and structural features are similar to the parent porphyrin.
This macrocycle 8-H2 is a monovalent anionic ligand. The diamagnetic 8-Zn(II) and 8-Cd(II) complexes and a paramagnetic 8-Ni(II) complex have been reported, coordinated to three pyrrolic nitrogens.14 Coordination imposes a steric constraint on the ligand geometry and leads to stereoisomers with the annulene part oriented outward-8-M(II) (out-8-M(II)) or inward-8-M(II) (in-8-M(II)) (M = Zn, Cd and Ni) macrocyclic center (Scheme 3). The out-8-Zn(II), out-8-Cd(II) and the free-base 8-H2 share a common 1H NMR spectral pattern as the basic structural features, which are preserved after the metal ion insertion. The 1H NMR spectra of in-8-Zn(II) and in-8-Cd(II) reflect a considerable decrease of aromaticity accounted by the inverted geometry.
The 8-Fe(II) also showed the isomers at annulene part; the annulene chain oriented inward and outward geometry (Scheme 4).15 The out-8-Fe(II) is more reactive with molecular oxygen than another complex, and gave iron(II) 21-oxaporphyrin complex 9. All these complexes showed characteristics typical for high-spin iron(II) complexes of porphyrinoids.
Palladium(II) complexes of the 21-vacataporphyrin (out-8-PdCl) were also prepared (Scheme 5).16 The out-8-PdCl was photochemically changed to the complex 10 having Pd-C bond with leaving of an HCl molecule. The macrocycle revealed the unique structural flexibility triggered by coordination of palladium. The palladium vacataporphyrin complexes reveal Hückel aromaticity (out-8-PdCl, [out-8-Pd(H2O)]+ and 10) or Möbius antiaromaticity (11 and 12) of [18]annulene applying the butadiene fragment as a topology selector.
In this regards, divacataporphyrin (dideazaporphyrin) was independently reported by Lash and Latos-Grażyński and their co-workers.17,18 Lash et al. reported intermolecular McMurry coupling of dialdehyde 13 to give tetraphenyl dideazaporphyrin 15a in 23% yield. Latos-Grażyński et al. reported the synthesis of meso-tetraaryldivacataporphyrin 15b by heating ditelluraporphyrin 14 with concentrated HCl in o-dichlorobenzene (Scheme 6). This shows fundamental structural and spectroscopic features of the parent porphyrin, but the framework is flexible. 21-Vacataporphyrin and divacataporphyrin have been investigated in the field of photodynamic therapy (PDT).19
Texaphyrin was prepared by a Schiff-base derived macrocycle by Sessler et al.20 Diformyltripyrrane 16 was reacted with ethylenediamine or o-phenylenediamine derivatives to afford the hydrotexaphyrins 17. It lacks a pyrrolic nitrogen atom from the patent porphyrin and replaces two carbon atoms to nitrogen atoms. These macrocycles behaved as mono-anionic pentadentate structures that lead to various metal complexes 17-M (Scheme 7). In particular, lanthanide ions could be coordinated to texaphyrin and these lanthanide metal complexes were used for biomolecule imaging and PDT. There are several satisfactory reviews and books about texaphyrins and their metal complexes.21,22
2-3 [18]TRIPHYRINS(4.1.1)
In 1999, Lee et al. reported Schiff-base derived [18]oxatriphyrin(4.1.1) derivatives.23 These macrocycles were synthesized from diformylheterotripyrranes with diamine compounds (Scheme 8). In the case of diformyloxatripyrrane 18a, it reacted with hydrazine to afford the azo-bridged macrocycle 19 in 43% yield.23 In addition, a reaction of 18a and 1,2-diphenyl-ethylenediamine caused the unusual rearrangement to afford the [18]oxatriphyrin(4.1.1) 20 in 23% yield,23 while diformylthiatripyrrane 18b and 1,2-diphenyl-ethylenediamine gave only macrocycle 21 which was formed by a general addition-elimination reaction of aldehyde and amine.24 From 1H NMR spectra, the N-H protons of 20 were observed at -1.38 and -0.84 ppm. This result indicated clear evidence of macrocyclic aromaticity. The absorption spectrum of 20 showed Soret-like band at 381 nm and five less-intense bands at 436, 461, 489, 554 and 603 nm, similar absorption shape with corroles, indicating [18]oxatriphyrin(4.1.1) 20 has the corrole-like macrocyclic aromatic characters. In contrast, NH-protons of macrocycle 21 were observed at 9.89 ppm and 8.08 ppm at 223 K, which were the chemical shifts of normal pyrrolic hydrogen.
Then Latos-Grażyński et al. have reported core-modified [18]triphyrin(4.1.1) compounds (Scheme 9). These compounds include one ethynyl bond at meso-position and one or two thiophene rings instead of pyrrole rings. Dithiaethyneporphyrin 23 has two thiophene rings and one pyrrole ring with monovalent property.25 Dithiaethyneporphyrin 23 was obtained from 1,4-bis(4-(phenylhydroxymethyl) thien-2-yl)-1,4-diphenyl-2-butyne 22 and pyrrole in the presence of methanesulfonic acid by [3+1] condensation method in 13% yield. NH proton was observed at -3.24 ppm to show the macrocyclic ring-current effect. Two canonical electronic structures 23ac and 23cum can be described as reflecting the combination of acetylene and cumulene character from 13C NMR chemical shifts. X-Ray single crystal analysis showed the bow-like deformation at the butyne moiety with C-C bond lengths of 1.390(9), 1.231(19), and 1.418(10) Å, respectively. It could make Ru(II) complex 24 with one Cl– and two CO ligands. Due to the lower symmetry of 1H NMR spectrum, octahedral coordination of dithiaethyneporphyrin with severely tilted two thiophene rings to opposite side was considered. Double-decker Fe(II) complex 25 was also reported.26 This was the first example of porphyrin double-decker complex reported for the transition metals of groups 6 to 11. Dithiaethyneporphyrin rings can rotate around the Fe(II) ion. The variable temperature 1H NMR studies revealed two enantiomeric couples of four fundamentally staggered rotamers were involved. In the presence of O2, dithiaethyneporphyrin 23 showed oxidative cleavage to open-chain diastereomer 26 terminated by carbonyl groups.27
Dithiaethyneazuliporphyrin 27 has one azulene and two thiophene rings on behalf of three pyrrole rings (Scheme 10).28 It was prepared by Rothemund-type condensation of diol compound 22 and azulene in 15% yield. Three canonical structures defined 18 π-electron macrocyclic delocalization pathways. From 13C NMR, acetylene carbon atoms of 27 were observed at 106.1 ppm, upfield region compared to dithiaethyneporphyrin 23 (116.8 ppm). Thus the acetylene character prevailed in this compound. It could make ruthenium complex 28 as a monoanionic ligand, forming the Ru-C σ-bond at inner carbon of azulene. This complex was Cs symmetry with a mirror plane passing through the ruthenium, chloride, and coordinated azulene carbon.
In 2012 thiaethyneporphyrin 30 with one thiophene and two pyrrole rings was obtained in a simple modification of the [3 + 1] approach described for the synthesis of porphyrins (Scheme 11).29 NH protons at -3.29 ppm and β-pyrrolic hydrogen atoms confirmed the 18π-electron structure. From 13C NMR spectrum, both of the acetylene and cumulene structure contributed to the electronic structure, although the cumulenic character was predominant. Two-electron oxidation of thiaethyneporphyrin gave 16π-compound 302+ and the NH protons at 25.35 ppm showed the paratropic ring current was present in the macrocycle. Making intramolecular metal(II)−η2-CC bond, 31-Pd and 31-Ni complexes were obtained. 31-Pd complex was reduced by NaBH4, then Pd(II)−η2-C1C2 interaction has been replaced by the typical Pd(II)−C σ bond (Pd−C1), affording the complex 32 of a regular carbaporphyrinoid, which was observed by 1H NMR measurement. The NaBH4 treatment of free base thiaethyneporphyrin 30 gave aromatic 20-thiaethyeneporphyrin. The NaBH4 treatment of Ni(II) complex only gave the demetalation. The titration of 31-Ni with pyridine converted the low-spin species into the high-spin complex due to the axial ligand.
2-4 [15]TRIPHYRINS(3.1.1)
The synthesis of [15]triphyrin(3.1.1) 34 has been reported by Anderson et al. (Scheme12).30 The acid-
catalyzed Rothemund condensation of TIPS-propynal and diethylpyrrole 33 followed by the oxidation with DDQ gave [15]triphyrin(3.1.1) 34 with corrole, porphyrin, [24]pentaphyrin(1.1.1.1.1), [28]hexaphyrin(1.1.1.1.1.1), and two linear tripyrromethenes. In the BF3·OEt2 catalyzed reaction [15]triphyrin(3.1.1) 34 was obtained in 3.5% as a byproduct, but it became the main product with 26% yield when the reaction was catalyzed with TFA. The reaction mechanism is shown in Scheme 12. From the X-ray crystal structural analysis of Zn complex 35, Zn and exocyclic C=C bond revealed a remarkable interaction as zinc−η2-alkene complex.
3. SYNTHESIS AND CHARACTERIZATION OF FREE-BASE [14]TRIPHYRINS(2.1.1)
3-1 SYNTHESIS AND STATISTIC PROPERTIES
Recently, [14]triphyrin(2.1.1) 37 has been reported as a new family of free-base 14π-ring contracted porphyrins. The first [14]triphyrin(2.1.1) 37a was prepared serendipitously by Shen, Yamada, You, and Kobayashi et al. in 2008 by a modified Lindsey’s method (Scheme 13).31 They intended the preparation of meso-tetraaryl bicycle[2.2.2]octadiene(BCOD)-fused-porphyrin 38 in the presence of BF3•OEt2 by the
previously reported method.32 Then, interestingly, the treatment of an equimolar mixture of BCOD-fused pyrrole 36 and methyl 4-formylbenzoate with 1.2 eq. of BF3•OEt2 followed by oxidation with DDQ gave [14]triphyrin(2.1.1) 37a in 34% yield unexpectedly, while with the normal concentration (0.4 eq.) of BF3•OEt2 gave tetraarylporphyrin 38 as usual. This [14]triphyrin(2.1.1) 37a has flat and metal-free structure, which is the first example of metal free ring contracted porphyrinoid with 14π aromaticity. The reaction was monitored by the change of UV-vis spectra. In the presence of 1.2 eq. of acid, typical spectra of triphyrin 37a (411 nm) and porphyrin 38 (457 nm) were detected 1 h after the acid addition, but gradually the Soret band at 457 nm of porphyrin 38 decreased, and after 12 h only the spectrum of triphyrin 37a was observed. In the presence of 0.4 eq. of acid, no triphyrin 37a was observed during the reaction. Therefore the accidental high concentration of acid seems to induce the preparation of [14]triphyrin(2.1.1) 37a.33 The [14]triphyrins(2.1.1) 37b-e were also prepared with 4-fluorophenyl,
phenyl, 4-tolyl, and 4-methoxybenzaldehyde in 20-40% yields (Scheme 14). The pyrrole moiety was also changeable to 3,4-diethylpyrrole 33 and dihydroethanonaphthalene pyrrole 41 moieties with relatively lower yield. The BCOD framework can be converted to benzo- or naphtho-group by retro-Diels-Alder reaction at 220 ºC or 300 ºC, respectively.33 Thus, the Lindsey’s method is suitable for the tetraaryl- triphyrins with same substituents at β-positions of pyrroles and meso-position, respectively. If pyrroles and arylaldehydes are available, the triphyrins can be obtained only by acid-catalyzed cyclization and oxidation.
In 2011, we reported meso-free-hexaalkyl-triphyrins 46 by an intramolecular McMurry coupling of diformyltripyrrane 44 followed by oxidation with DDQ in 16% yield in 2 steps (Scheme 15).34 The intermediate 45 before oxidation was purified and characterized successfully. McMurry coupling needs stepwise synthesis of tripyrranes, but the substituents at β-position can be changed freely. The core-modified triphyrin can be also expected by insertion of heteroaromatic compounds instead of pyrrole. The meso-aryl-β-free triphyrin(2.1.1) 48 was reported by Anju et al. in 2011, by acid-condensation of 5,6-diphenyldipyrromethane 47 with pentafluorobenzaldehyde, followed by oxidation with DDQ in 5% yield (Scheme15).35 The mechanism was speculated as the condensation reaction of the starting materials with a free pyrrole obtained in situ, followed by an oxidation. This was the first example of β-free triphyrin(2.1.1) with two kinds of aryl groups at meso-positions.
In general 1H NMR spectra of porphyrin NH protons are observed around 0 to -2 ppm due to the diatropic ring current of the porphyrin macrocycle. On the contrary triphyrins showed unusual downfield shifts of inner NH protons at 7.68 ppm for 37a, 8.16 ppm for 40a, 9.0 ppm for 43, 7.25 ppm for 46 and 9.07 ppm for 48 due to the strong inner hydrogen bonding. Such downfield shift was also observed for porphycenes,36 isocorroles37 and N-fused porphyrins38 with modified central cavities containing significantly shorter N•••N distances than conventional porphyrinoids.
The crystal structures of 37c, 40c and 46 are shown in Figure 3. Different from the known [14]triphyrin(1.1.1) compounds, [14]triphyrins(2.1.1) are planar metal-free macrocycles and can be anionic monodentate cyclic ligands. For each triphyrin, pyrroles are slightly tilted from the plane made by meso- carbons. In particular, the angle of pyrrole 2 and the mean plane of four meso-carbons is larger than that made by the other two pyrroles and the mean plane. This is due to the stronger hydrogen bonding
between nitrogen atoms of pyrroles 1 and 3 by the planar conformation. The pivot freedom of pyrrole 1 let the [14]triphyrin(2.1.1) coordinate to the various metal ions, as described in section 4-1.
3-2 SPECTROSCOPIC PROPERTIES
UV-vis absorption spectra of 37c and 40c are shown in Figure 4. Triphyrin 37c showed B-like band at 373 nm and broad Q-like band reached to 600 nm in CH2Cl2. With the expansion of π-conjugation, 40c showed B-like band at 414 nm and Q-like band at 523 and 578 nm;31 43 showed B-like band at 447 nm and Q-like band at 561and 614nm in CH2Cl2.33 The fusion of phenyl rings at β-positions of pyrrole moieties induced the red-shifts of absorption spectra. Triphyrin 46 showed blue-shift compared to the 37c: B-like band at 336 nm and Q-like band at 440-560 nm with broad peaks at 470 and 550 nm.34
Triphyrins 40c and 48 were fluorescent at room temperature and the fluorescence quantum yields were 0.018 and 0.008, respectively.33,35 Kim et al. investigated the comparative photophysical properties of 37c and 40c.39 They concluded 40c increased the overall molecular planarity and rigidity, which explained the relatively long fluorescence lifetimes and red-shifted fluorescence and absorption spectra of 40c. Tominaga et al. also reported the nonplanarity of the macrocycle enhanced the internal conversion from the singlet excited state, which shorten the excited state lifetime of 40c.40
4. SYNTHESIS AND CHARACTERIZATION OF [14]TRIPHYRIN(2.1.1) METAL COMPLEXES
The inner three nitrogen atoms of [14]triphyrin(2.1.1) consist of the two imine and one amine nitrogen atoms and act as monoanionic-tridentate ligands. Cyclic monoanionic tridentate ligands have been extensively studied in the field of coordination-chemistry. The typical examples are subpyriporphyrin,11 vacataporphyrin,13-16 thiaethyneporphyrins,25-29 [15]triphyrin(3.1.1)30 and N-fused porphyrins (NFPs) (Scheme 16).38 The coordination abilities and properties of their metal complexes except for NFPs have been described above. NFPs 50 also behave as the mono-anionic-tridentate ligands which can coordinate to Re(Ⅰ),41 Re(Ⅶ),42 Mn(Ⅰ),43 Ru(Ⅱ),44 Fe(II),45 B(Ⅲ),46 Si(IV)47 and P(Ⅴ)48 ions. These metal complexes have the nature of near-infrared absorption, the generation of singlet oxygen and the ability to work as catalysts. In particular, Re(Ⅶ)O3NFP can catalyze deoxygenation reaction of pyridine N-oxide derivatives, affording the corresponding pyridine derivatives quantitatively.42 These NFP metal complexes were summarized as good review and book by Furuta.44,49 Therefore, in this session we describe the recent results of the metal complexes of [14]triphyrins(2.1.1).
4-1 OCTAHEDRAL COORDINATED MANGANESE(I), RHENIUM(I) AND RUTHENIUM(II) COMPLEXES
Due to the flexibility of the macrocycle, [14]triphyrin(2.1.1) can coordinate to various metal ions. In 2011, 46-Mn(I)(CO)3,34 46-Re(I)(CO)3,34 and 40a-Re(I)(CO)333 complexes were prepared by the reaction of triphyrins 40a and 46 with MnBr(CO)5 or ReCl(CO)5, in toluene in the presence of NaOAc (Scheme 17).
The crystal structures are shown in Figure 5. Three inner nitrogen atoms and three CO moieties are coordinated to the Mn(I) or Re(I) ions, respectively. The triphyrins are bowl-shaped structure and Mn and Re ions sit 1.300 Å and 1.455 Å above the plane made by three inner nitrogen atoms, respectively. The distances between C2-C13 and C7-C16 are 5.986(3) and 6.496(3) Å for 46-Mn(I)(CO)3 and 5.981(3) and 6.383(3) Å for 46-Re(I)(CO)3, respectively. The nitrogen-nitrogen distances of N1-N2, N2-N3, and N3-N1 were 2.586(2), 2.594(2), and 2.696(2) Å for 46-Mn(I)(CO)3 and 2.650(2), 2.654(2), and 2.779(2) for 46-Re(I)(CO)3, respectively. Each N-N length of 46-Mn(I)(CO)3 is 0.06 Å shorter than that of 46-Re(I)(CO)3, because of the larger size of Re(I) ion than Mn(I) ion. This also influences the shape of the bowl: the curve of 46-Re(I)(CO)3 is deeper than that of 46-Mn(I)(CO)3.
The bowl depth of 40a-Re(I)(CO)3, defined as 2.248 Å by the distance from the metal atom to the mean plane of the six peripheral pyrrole β-carbon atoms, is similar to that reported for hydroxy-ligated boron tribenzosubporphyrin (2.33 Å)6, but the dihedral angles of the meso-aryl substituents (Φ1–Φ4) are significantly larger. The average Re-N and Re-C (CO) bond lengths of 2.129 and 1.915 Å, respectively, are similar to values reported previously for the Re(I)- complex of NFP.41
Ruthenium complex 40c-Ru(II)Cl(CO)2 was prepared by the reaction of 40c with Ru3(CO)12 (Scheme 18).33 The 40-Ru(II)Cl(CO)2 and 46-Ru(II)Cl(CO)2 were also prepared using [Ru(CO)2Cl]2.50 The X-ray crystal structure of these Ru(II) complexes were obtained as octahedral structures (Figure 6).
4-2 PLATINUM(II) AND PLATINUM(IV) COMPLEXES
Considering the tridentate structure of [14]triphyrin(2.1.1), it is challenging to make the square-planar Pt(II) complex. We reported the treatment of 40d with PtCl2 under N2 atmosphere and the subsequent separation over a silica gel column chromatography using CHCl3 as an eluent to provide 40d-Pt(IV) as a green fraction (Rf = 0.71) in trace and then 40d-Pt(II) as a yellow green fraction (Rf = 0.12) in 59% yield, respectively (Scheme 19).51 When the same reaction was performed under air, then 40d-Pt(II) and 40d-Pt(IV) were obtained in 47% and 11% yields, respectively. These results suggested the Pt(II) complex was oxidized to Pt(IV) complex under air. The UV-vis spectra of 40d, 40d-Pt(II), and 40d-Pt(IV) are shown in Figure 7.
Single crystals of 40d-Pt(II) were obtained from N2-bubbled solvent (CH2Cl2/MeOH) under N2 atmosphere in a glove box. The crystal structure of 40d-Pt(II) is shown in Figure 8. The Pt(II) ion is coordinated to two pyrrolic nitrogen atoms and two chloride ions with following bond lengths: 2.021(4) Å for Pt-N(1) and 2.314(1) Å for Pt−Cl in cis-configuration to form a rigid square-planar conformation. The triphyrin macrocycle shows saddle shape with CS symmetry. The mutual angle between two pyrrole rings coordinated to Pt ion is 119.36° with C(1)-C(14) distance of 3.131(7) Å. The angle between the non-coordinated pyrrole ring and the NNClCl plane is 43.69°.
When the 40d-Pt(II) was crystallized from CH2Cl2/EtOH solution under air, green plate-shape crystals were obtained. It was a new Pt(II)-TriP complex, 40d-Pt(II)O, with oxygen adduct between two pyrrole rings as shown in Figures 8b. The space group of the crystal for 40d-Pt(II)O was P21/m and the molecule 40d-Pt(II)O was positioned in a mirror plane. An oxygen atom is incorporated between C(1) and C(14) atoms to form a 2,5-dihydrofuran ring, with C(1)−O bond length of 1.47(1) Å. The whole molecular π-conjugation system of 40d-Pt(II)O is interrupted with C(1) sp3 carbons. The bond length of C(15)−C(16) (1.331(1) Å) is close to normal C=C double bond and significantly shorter than that of 40d-Pt(II) (1.386(7) Å). The bond lengths connected to C(1) atom (1.53(1) Å for C(1)−C(16); 1.52(1) Å for C(1)−C(2); 1.49(1) Å for C(1)−N(1)) in 40d-Pt(II)O are obviously longer than the corresponding bond lengths of 40d-Pt(II), 1.475(19), 1.384(19), and 1.352(6) Å, respectively. The newly formed dihydrofuran moiety shows chair-shape, bended by 33.18° at C(15)−C(16) axis (Figure 8b). The mutual angle between two pyrrole rings coordinated to Pt ion is 109.04° and the C(1)−C(14) distance is only 2.32 Å, indicating the deeper saddle shape compared with 40d-Pt(II). The angle between the non-coordinated pyrrole ring and the NNClCl plane of 40d-Pt(II)O is 46.53°, lifting Pt-plane 2.86° more than 40d-Pt(II).
The single crystals of 40d-Pt(IV) were obtained from toluene/n-hexane solution. The X-ray structure analysis revealed a bowl-shaped conformation for 40d-Pt(IV), similar to 40a-Re(I)(CO)3.33 The distance between Pt(IV) ion and the NNN plane is 1.275 Å, which is shorter than the corresponding distances in Re(I) complex by 0.201 Å and is more flat than the Re(I) complex. The bond length of C(15)−C(16) (1.43(1) Å) is longer than those of 40d-Pt(II) and 40d-Pt(II)O and the bond lengths of the macrocyclic ring suggest the clear 14π-conjugation.
4-3 IRON(II) AND IRON(III) COMPLEXES
Ferrocene, an iron(II) center sandwiched by a pair of aromatic cyclopentadienyl (Cp) ligands, is the first known and archetypal metallocene that was discovered in 1951.52 After that, research into ferrocene-containing compounds continues apace within diverse areas.53 However the larger macrocyclic π-conjugated system with monodentate anionic character is little reported so far, due to the weak coordination of π-extended Cp-type ligands. In particular, in porphyrin families there are a few reports in this context.54 (1) Cp-Sc(III)−porphyrin,55a Cp−Zr(II)−porphyrin,55b Cp*−Ru(IV)−porphycene (Cp* = pentamethyl-cyclopentadienyl),56 although porphyrin and porphycene are bidentate ligands; (2) β,β’-fused monoruthenocenylporphyrins and bisferrocenoporphyrins,57a metaloporphycenes57b and cyclopentadienylruthenium π complexes of subphthalocyanines57c where five-membered ring part (pyrrole or cyclohexadiene moiety) acted as ligands.57 Only recently double-decker iron(II) complexes of dithiaethyneporphyrin26 and NFP,45 where macrocycles
behaved as macrocyclic tridentate ligands with a single negative charge, have been reported. During the synthesis of NFP complex, the Cp−Fe(II)−NFP has been detected by mass spectra but could not be separated. To data, the synthesis of Cp−Fe(II)−porphyrin sandwich compound remains a considerable challenge.
behaved as macrocyclic tridentate ligands with a singleThe treatment of 40a and 40d with [Fe(CO)2(Cp)]2 gave sandwich η5-cyclopentadienyl-iron(II) triphyrin complexes (40a-Fe(II) and 40d-Fe(II)) in 40-45% yields (Scheme 20), whose structures were assigned by 1H NMR, HR-ESI-MS and X-ray diffraction analysis (Figure 9a).58 The redox behavior of Fe ion of the complex was reversible but the potential was 0.54 V lower than that of ferrocene. Fe(III) complex was stable and could be detected by ESR measurement. The protonated Fe(II) complex was easily oxidized to Fe(III) complex to show the same UV-vis spectrum with the electrochemically oxidized Fe(III) complex.negative charge, have been reported. During the synthesis of NFP complex, the Cp−Fe(II)−NFP has been detected by mass spectra but could not be separated. To data, the synthesis of Cp−Fe(II)−porphyrin sandwich compound remains a considerable challenge.
The UV-vis spectrum was reversibly changed to Fe(II) complex by adding DBU.
This stability allowed us to make single crystals of the oxidative state of 40d-Fe(III). Fortunately, the crystallization of the oxidized 40d-Fe(II) with excess amount of CF3SO3Ag gave tiny crystals, from which we could perform the X-ray diffraction analysis (Figure 9b). The determined structure contained [CF3SO3]2Ag]– as a counter anion. The Cp ring is coordinated to Fe(III) ion through the five carbon atoms, with the distance of 1.723 Å, which is longer than that of 40d-Fe(II), suggesting that the additional positive charge in 40d-Fe(III) is mostly localized on the formal Fe(III) atom.
4-4 TETRAHEDRAL COORDINATED BORON(III) COMPLEXES
The boron complexes of triphyrins are standing on the very important place of the contracted porphyrins since most of the previously reported triphyrins have been coordinated to boron atom. [14]Triphyrins(2.1.1) boron complexes were obtained from free-base [14]triphyrins(2.1.1) 40c and 46 with phenyl boron dichloride (Scheme 21).59 In 1H NMR spectrum, B-phenyl protons of 46-B(III) were observed at 3.24 (ortho), 5.99 (meta) and 6.27 (para) ppm, which are the characteristic high-field shifts for the diatropic ring current of the triphyrin macrocycle. The signals of axial phenyl ring of 40c-B(III) were also observed at 4.00 (ortho), 6.28 (meta) and 6.52 (para) ppm. The crystal structures of 40c-B(III) and 46-B(III) are shown in Figure 10. Boron atom was coordinated by three nitrogen atoms and one phenyl carbon atom.
5. CORE-MODIFIED TRIPHYRINS
5-1 THIATRIPHYRIN(2.1.1) DERIVATIVES
It is well known that remarkable changes in the optical and electrochemical properties and coordination abilities of porphyrins can be induced by core-modification of porphyrinoids.60,61 In light of this, it is naturally expected that the core-modification of triphyrins will also give them new functionality.
We tried the McMurry coupling reaction of 52 to give 5,10-dihydro-5,10-ditolylthiatriphyrin 53 in 50% yield, which is an intermediate for the [14]thiatriphyrin(2.1.1) 56 (Scheme 22). The direct oxidation of 53 with 2 eq. of DDQ in CHCl3 was not successful to give the desired thiatriphyrin 56, but resulted in the formation of small amount of the thiatriphyrin 54b with an ethoxy group at the meso-position, where ethanol was assumed to come from the presence of ethanol in CHCl3. The step-wise oxidation of 53 with 1 eq. of DDQ in CH2Cl2 gave 5-hydrothiatriphyrin 55 in 50% yield, then the additional oxidation gave the alkoxy-substituted triphyrins 54a-c in the presence of nucleophile like methanol, ethanol, and isopropanol, respectively. The second oxidation in the absence of nucleophiles induced only the decomposition.62
Alkoxy group attached thiatriphyrin derivatives 54a-c were characterized by NMR and X-ray single crystal diffraction analysis. From 1H NMR, the signal of inner NH-proton of 54b was observed at 12.7 ppm, which suggested the nature of hydrogen bonds between inner nitrogen atoms. The determined X-ray crystal structure of 54b is shown in Figure 11a. The ethoxy group is oriented in the same direction as the sulfur atom relative to the plane of the macrocycle. The bond lengths and angles in the pyrrole moieties indicate that one of the pyrrole units has the structure of an imine, whereas the other has an amine structure. This observation suggests a hydrogen-bonding interaction between the two inner nitrogen atoms. The thiophene ring is significantly tilted out of the plane of the macrocycle, with a dihedral angle between the thiophene ring and pyrrole of 69.53°. The elimination of alkoxy groups under acidic conditions has been performed, following the method reported for subpyriporphyrins,11 an N-fused porphyrin–boron complex,46 dithiaethyneporphyrin,25 and a homoporphyrin–nickel complex.63 Then the protonated thiatriphyrin 56+ was obtained by addition of acid and it was reversible to 56 in the presence of DBU (Scheme 23).
Fortunately, the crystal structure of 56+ was obtained (Figure 11b). The thiophene ring is also tilted out of the macrocycle plane and the dihedral angle between thiophene ring and pyrrole was 50.28º. Furthermore, the desired thiatriphyrin 56 is expected to have two iminium-type nitrogen and the lone pairs of the nitrogen atoms would be a hindrance to each other to give a stable structure. The protonation of one of the pyrroles would help the stabilization of 56+ by the hydrogen bonding between the two pyrrole rings.
6. CONCLUSION
In this account we have featured on the synthesis, structures and optical properties of a new group of ring-contracted porphyrin, [14]triphyrin(2.1.1) and its metal complexes, in comparison with other ring-contracted porphyrins. [14]Triphyrin(2.1.1) was discovered serendipitously as the first metal-free 14π-porphyrinoid 5 years ago, and afterwards several synthetic routes have been developed by us and others to modify the substituents at meso- and β-positions. The remarkable properties of [14]triphyrins(2.1.1) are the flexibility of the macrocycle structure as monoanionic tridentate ligand which enables them to coordinate to a variety of metal ions like Re(Ⅰ), Mn(Ⅰ), Ru(Ⅱ), Pt(II), Pt(IV), Fe(II) and B(III) ions as octahedral, square-planar, sandwich-type or tetrahedral complexes. Core-modified triphyrins might be also realizable considering the synthetic scheme, but thiatriphyrin was not isolated due to the large atomic diameter of sulfur atom in the triphyrin cavity and the electronic repulsion of lone pairs of inner nitrogen atoms, instead alcohol adducts were obtained successfully. Further synthesis of metal complexes and core-modified triphyrins will be possible and various properties and functions are remains to be found.
ACKNOWLEDGEMENTS
This work was partly supported by JSPS Postdoctoral Fellowship for Foreign Researchers, Grants-in-Aid No 24655034 and the Green Photonics Project in NAIST sponsored by the MEXT, Japan. We thank Prof. Hidemitsu Uno and Dr. Shigeki Mori in Ehime University and Prof. Naoki Aratani in Nara Institute of Science and Technology for the X-ray single crystal analysis of [14]triphyrin(2.1.1) compounds. Finally, special thanks are given to those listed as the coauthors in our papers cited here, particularly to Dr. Zhao-Li Xue in Jiangsu University, Prof. Zhen Shen in Nanjing University and Yuka Sakakibara in NAIST for their contribution to these results.
References
1. For reviews. a) Z. Gross, J. Biol. Inorg. Chem., 2001, 6, 733; CrossRef b) D. T. Gryko, Eur. J. Org. Chem., 2002, 1735; CrossRef c) A. Ghosh and E. Steene, J. Inorg. Biochem., 2002, 91, 423; CrossRef d) I. Aviv and Z. Gross, Chem. Commun., 2007, 1987; CrossRef e) L. Flamigni and D. T. Gryko, Chem. Soc. Rev., 2009, 38, 1635; CrossRef f) I. Aviv-Harel and Z. Gross, Chem. Eur. J., 2009, 15, 8382; CrossRef g) H.-Y. Liu, M. H. R. Mahmood, S.-X. Qiu, and C. K. Chang, Coord. Chem. Rev., 2013, 257, 1306. CrossRef
2. a) A. Ghosh, I. H. Wasbotten, W. Davis, and J. C. Swarts, Eur. J. Inorg. Chem., 2005, 4479; CrossRef b) M. Bröring, S. Köhler, and C. Kleeberg, Angew. Chem. Int. Ed., 2008, 47, 5658; CrossRef c) T. Ito, Y. Hayashi, S. Shimizu, J.-Y. Shin, N. Kobayashi, and H. Shinokubo, Angew. Chem. Int. Ed., 2012, 51, 8542. CrossRef
3. For reviews. a) A. Jasat and D. Dolphin, Chem. Rev., 1997, 97, 2267; CrossRef b) J. L. Sessler and D. Seidel, Angew. Chem. Int. Ed., 2003, 42, 5134; CrossRef c) J. M. Lim, Z. S. Yoon, J.-Y. Shin, K. S. Kim, M.-C. Yoon, and D. Kim, Chem. Commun., 2009, 261; CrossRef d) S. Saito and A. Osuka, Angew. Chem. Int. Ed., 2011, 50, 4342; CrossRef e) M. Stępień, N. Sprutta, and L. Latos-Grażyński, Angew. Chem. Int. Ed., 2011, 50, 4288. CrossRef
4. A. Meller and A. Ossko, Monatsh. Chem., 1972, 103, 150. CrossRef
5. M. S. Rodríguez-Morgade, S. Esperanza, T. Torres, and J. Barberá, Chem. Eur. J., 2005, 11, 354. CrossRef
6. Y. Inokuma, J. H. Kwon, T. K. Ahn, M.-C. Yoo, D. Kim, and A. Osuka, Angew. Chem. Int. Ed., 2006, 45, 961. CrossRef
7. Y. Inokuma, Z. S. Yoon, D. Kim, and A. Osuka, J. Am. Chem. Soc., 2007, 129, 4747. CrossRef
8. N. Kobayashi, Y. Takeuchi, and A. Matsuda, Angew. Chem. Int. Ed., 2007, 46, 758. CrossRef
9. E. Tsurumaki, S. Hayashi, F. S. Tham, C. A. Reed, and A. Osuka, J. Am. Chem. Soc., 2011, 133, 11956. CrossRef
10. a) C. G. Claessens, D. González-Rodríguez, and T. Torres, Chem. Rev., 2002, 102, 835; CrossRef b) Y. Inokuma and A. Osuka, Dalton Trans., 2008, 2517; CrossRef c) A. Osuka, E Tsurumaki, and T. Tanaka, Bull. Chem. Soc. Jpn., 2011, 84, 679. CrossRef
11. R. Myśliborski, L. Latos-Grażyński, L. Szterenberg, and T. Lis, Angew. Chem. Int. Ed., 2006, 45, 3670. CrossRef
12. K. S. Kim, J. M. Lim, R. Myśliborski, M. Pawlicki, L. Latos-Grażyński, and D. Kim, J. Phys. Chem. Lett., 2011, 2, 477. CrossRef
13. E. Pacholska, L. Latos-Grażyński, and Z. Ciunik, Chem. Eur. J., 2002, 8, 5403. CrossRef
14. E. Pacholska-Dudziak, J. Skonieczny, M. Pawlicki, L. Szterenberg, and L. Latos-Grażyński, Inorg. Chem., 2005, 44, 8794. CrossRef
15. E. Pacholska-Dudziak, A. Gaworek, and L. Latos-Grażyński, Inorg. Chem., 2011, 50, 10956. CrossRef
16. E. Pacholska-Dudziak, J. Skonieczny, M. Pawlicki, L. Szterenberg, Z. Ciunik, and L. Latos- Latos-Grażyński, J. Am. Chem. Soc., 2008, 130, 6182. CrossRef
17. T. D. Lash, S. A. Jones, and G. M. Ferrence, J. Am. Chem. Soc., 2010, 132, 12786. CrossRef
18. E. Pacholska-Dudziak, L. Szterenberg, and L. Latos-Grażyński, Chem. Eur. J., 2011, 17, 3500. CrossRef
19. M. Klyta, P. Ostasiewicz, K. Jurczyszyn, K. Duś, L. Latos-Grażyński, E. Pacholska-Dudziak, and P. Ziółkowski, Laser Surg. Med., 2011, 43, 607. CrossRef
20. J. L. Sessler, T. Murai, V. Lynch, and M. Cyr, J. Am. Chem. Soc., 1988, 110, 5586. CrossRef
21. a) J. L. Sessler, G. Hemmi, T. D. Mody, T. Murai, A. Burrell, and S. W. Young, Acc. Chem. Res., 1994, 27, 43; CrossRef b) J. L. Sessler, V. Král, M. C. Hoehner, K. O. A. Chin, and R. M. Dávila, Pure Appl. Chem., 1996, 68, 1291; CrossRef c) J. L. Sessler and R. A. Miller, Biochem. Pharmacol., 2000, 59, 733; CrossRef d) D. J. Magda, Z. Wang, N. Gerasimchuk, W. Wei, P. Anzenbacher, and J. L. Sessler, Pure Apple Chem., 2004, 76, 365; CrossRef e) S. R. Thomas and D. Khuntia, Int. J. Nanomedicine, 2007, 2, 79; CrossRef f) J. F. Arambula, C. Preihs, D. Borthwick, D. Magda, and J. L. Sessler, Anticancer Agents Med. Chem., 2011, 11, 222; CrossRef g) C. Preihs, J. F. Arabula, D. Magda, H. Jeong, D. Yoo, J. Cheon, Z. H. Siddik, and J. L. Sessler, Inorg. Chem., Article ASAP, DOI: 10.1021/ic400226g. CrossRef
22. ‘Expanded. Contracted & Isomeric Porphyrins’, J. L. Sesller and D. T. Gryko, Pergamon, 1997.
23. C. H. Lee, J. W. Ka, and D. H. Won, Tetrahedron Lett., 1999, 40, 6799. CrossRef
24. C. H. Lee and K. T. Oh, Tetrahedron Lett., 1999, 40, 1921. CrossRef
25. A. Berlicka, L. Latos-Grażyński, and T. Lis, Angew. Chem. Int. Ed., 2005, 44, 5288. CrossRef
26. A. Berlicka and L. Latos-Grazyński, Inorg. Chem., 2009, 48, 7922. CrossRef
27. A. Berlicka, L. Latos-Grażyński, L. Szterenberg, and M. Pawlicki, Eur. J. Org. Chem., 2010, 5688. CrossRef
28. A. Berlicka, N. Sprutta, and L. Latos-Grażyński, Chem. Commun., 2006, 3346. CrossRef
29. E. Nojman, A. Berlicka, L. Szterenberg, and L. Latos-Grażyński, Inorg. Chem., 2012, 51, 3247. CrossRef
30. A. Krivokapic, A. R. Cowley, and H. L. Anderson, J. Org. Chem., 2003, 68, 1089. CrossRef
31. Z.-L. Xue, Z. Shen, J. Mack, D. Kuzuhara, H. Yamada, T. Okujima, N. Ono, X.-Z. You, and N. Kobayashi, J. Am. Chem. Soc., 2008, 130, 16478. CrossRef
32. S. Ito, T. Murashima, N. Ono, and H. Uno, Chem. Commun., 1998, 1661. CrossRef
33. Z.-L. Xue, J. Mack, H. Lu, L. Zhang, X.-Z. You, D. Kuzuhara, M. Stillman, H. Yamada, S. Yamauchi, N. Kobayashi, and Z. Shen, Chem. Eur. J., 2011, 17, 4396. CrossRef
34. D. Kuzuhara, H. Yamada, Z.-L. Xue, T. Okujima, S. Mori, Z. Shen, and H. Uno, Chem. Commun., 2011, 47, 722. CrossRef
35. K. S. Anju, S. Ramakrishnan, and A. Srinivasan, Org. Lett., 2011, 13, 2498. CrossRef
36. E. Vogel, M. Köcher, H. Schmickler, and J. Lex, Angew. Chem., Int. Ed. Engl., 1986, 25, 257. CrossRef
37. E. Vogel, B. Binsack, Y. Hellwig, C. Erben, A. Heger, J. Lex, and Y.-D. Wu, Angew. Chem., Int. Ed. Engl., 1997, 36, 2612. CrossRef
38. H. Furuta, T. Ishizuka, A. Osuka, and T. Ogawa, J. Am. Chem. Soc., 1999, 121, 2945. CrossRef
39. Y. M. Sung, J. M. Lim, Z.-L. Xue, Z. Shen, and D. Kim, Chem. Commun., 2011, 47, 12616. CrossRef
40. Y. Iima, D. Kuzuhara, Z.-L. Xue, H. Uno, S. Akimoto, H. Yamada, and K. Tominaga, Chem. Phys. Lett., 2011, 513, 67. CrossRef
41. a) M. Toganoh, T. Ishizuka, and H. Furuta, Chem Commun., 2004, 2464; CrossRef b) M. Toganoh, S. Ikeda, and H. Furuta, Inorg. Chem., 2007, 46, 10003. CrossRef
42. M. Toganoh, S. Ikeda, and H. Furuta, Chem. Commun., 2005, 4589. CrossRef
43. S. Ikeda, M. Toganoh, and H. Furuta, Inorg. Chem., 2011, 50, 6029. CrossRef
44. M. Toganoh and H. Furuta, Handbook of Porphyrin Science, ed. K. M. Kadish, K. M. Smith, and R. Guilard, World Scientific Publication Co. Pte. Ltd, Singapore, 2010, Vol. 2, pp. 295-367.
45. M. Toganoh, A. Sato, and H. Furuta, Angew. Chem. Int. Ed., 2011, 50, 2752. CrossRef
46. A. Młodzianowska, L. Latos-Grażyński, L. Szterenberg, and M. Stȩpień, Inorg. Chem., 2007, 46, 6950. CrossRef
47. J. Skonieczny, L. Latos-Grażyński, and L. Szterenberg, Inorg. Chem., 2009, 48, 7394. CrossRef
48. A. Młodzianowska, L. Latos-Grazyński, and L. Szterenberg, Inorg. Chem., 2008, 47, 6364. CrossRef
49. M. Toganoh and H. Furuta, Chem. Commun., 2012, 48, 937. CrossRef
50. Unpublished data.
51. Z.-L. Xue, D. Kuzuhara, S. Ikeda, T. Okujima, S. Mori, H. Uno, and H. Yamada, Inorg. Chem., 2013, 52, 1688. CrossRef
52. a) T. J. Kealy and P. L. Pauson, Nature, 1951, 168, 1039; CrossRef b) Ferrocenes: Ligands, Materials and Biomolecules (Ed. by P. Stepnicka), Wiley, Chichester, 2008.
53. C. Bucher, C. H. Devillers, J.-C. Moutet, G. Royal, and E. Saint-Aman, Coord. Chem. Rev., 2009, 253, 21. CrossRef
54. L. Cuesta, J. L. Sessler, Chem. Soc. Rev., 2009, 38, 2716 and references cited therein. CrossRef
55. J. Arnold and G. C. Hoffman, J. Am. Chem. Soc., 1990, 112, 8620; CrossRef H.-J. Kim, S. Jung, Y.-M. Jeon, D. Whang, and K. Kim, Chem. Commun., 1997, 2201. CrossRef
56. L. Cuesta, E. Karnas, V. M. Lynch, P. Chen, J. Shen, K. M. Kadish, K. Ohkubo, S. Fukuzumi, and J. L. Sessler, J. Am. Chem. Soc., 2009, 131, 13538. CrossRef
57. H. J. H. Wang, L. Jaquinod, M. M. Olmstead, M. G. H. Vicente, K. M. Kadish, Z. Ou, and K. M Smith, Inorg. Chem., 2007, 46, 2898; CrossRef b) G. I. Vargas-Zúñiga, V. V. Roznyatovskiy, A. Nepomnyaschii, V. M. Lynch, and J. L. Sessler, J. Porphyrins Phthalocyanines, 2012, 16, 479; CrossRef c) E. Caballero, J. Fernández-Ariza, V. M. Lynch, C. Romero-Nieto, M. S. Rodríguez-Morgade, J. L. Sessler, D. M. Guldi, and T. Torres, Angew. Chem. Int. Ed., 2012, 51, 11337.. CrossRef
58. Z. Xue, D. Kuzuhara, S. Ikeda, Y. Sakakibara, K. Ohkubo, N. Aratani, T. Okujima, H. Uno, S. Fukuzumi, and H. Yamada, Angew. Chem. Int. Ed., DOI: 10.1002/anie.201302815and 10.1002/ange.201302815.
59. D. Kuzuhara, Z. Xue, S. Mori, T. Okujima, H. Uno, and H. Yamada, Chem. Commun., to be submitted.
60. L. Latos-Grazyński in The Porphyrin Handbook Vol. 2, (Ed. by K. M. Kadish, K. M. Smith, and R. Guilard), Academic Press, San Diego, 2000, pp. 361-416.
61. P. J. Chmielewski and L. Latos-Grazyński, Coordin. Chem. Rev., 2005, 249, 2510. CrossRef
62. D. Kuzuhara, Y. Sakakibara, S. Mori, T. Okujima, H. Uno, and H. Yamada, Angew. Chem. Int. Ed., 2013, 52, 336. CrossRef
63. H. J. Callot and E. Schaeffer, J. Org. Chem., 1977, 42, 1567. CrossRef