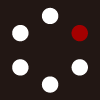
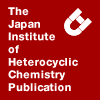
HETEROCYCLES
An International Journal for Reviews and Communications in Heterocyclic ChemistryWeb Edition ISSN: 1881-0942
Published online by The Japan Institute of Heterocyclic Chemistry
e-Journal
Full Text HTML
Received, 24th December, 2012, Accepted, 21st January, 2013, Published online, 31st January, 2013.
DOI: 10.3987/COM-12-12657
■ Synthesis of Tetracyclic Indoline and Indolenine Derivatives Having β-Lactam Using Amphiphilic Reactivity of 2-Methylindolenine
Atsuo Nakazaki, Yukari Hara, Shigeo Kajii, and Toshio Nishikawa*
Laboratory of Organic Chemistry, Graduate School of Bioagricultural Sciences, Nagoya University, Furo-cho, Chikusa, Nagoya, Aichi 464-8601, Japan
Abstract
Novel tetracyclic indoline and indolenine β-lactam derivatives were synthesized from 2-methylindolenine β-lactams using the amphiphilic nature of 2-methylindolenine.β-Lactam is a ubiquitous framework in biologically active compounds including naturally occurring antibiotics1 and drug candidates.2 β-Lactam is also incorporated in indole alkaloids such as chartelline marine alkaloids,3 whose unique structures have stimulated the development of synthetic methods for the construction of their core frameworks (Figure 1). During the course of our synthetic studies on the chartelline family,4 we have already developed synthetic methods for the construction of β-lactams bearing oxindole4a and indolenine.4b In the latter method, indolenine β-lactam was synthesized by an intramolecular nucleophilic substitution of the nitrogen atom of O-sulfonylated hydroxamic acid derivative (Scheme 1).
2-Methylindolenine is known to show amphiphilic reactivity,5 as shown in Scheme 2. Thus, 2-methylindolenine A could react as an imine with a nucleophilic functional group to provide 2,2-disubstituted indoline C. On the other hand, enamine B, a tautomer of A, could react with an electrophilic functional group to provide indolenine D in which the 2-methyl group can be functionalized. Because this amphiphilic reactivity is potentially useful for the construction of various indoline and indolenine compounds, we investigated the amphiphilic reactivity of 2-methylindolenine β-lactams to find two cyclizations leading to tetracyclic compounds.
In order to study the amphiphilic reactivity, we planned to investigate the cyclization of 2-methylindolenines with nucleophilic or electrophilic functionality on β-lactam (Scheme 3). Thus, allylsilane F and aldehyde H would be derived from a common hydroxamic acid allyl ester E, and then cyclizations to the functionalized 5-membered indoline G and 7-membered indolenine I were examined.
The synthesis of the cyclization precursors 8 (= F, in Scheme 3) and 9 (an equivalent of H) is described in Scheme 4. To prepare substrates 6a and 6b for β-lactam cyclization, we modified the procedure reported by this group.4b N-Boc indoleacetic acid 1, prepared from a commercially available 2-methylindoleacetatic acid, was converted into amide 2 using EDCI and allyloxyamine in 88% yield. N-Allylation of 2 was followed by the removal of O-allyl and Boc groups under conventional conditions to afford hydroxamic acid 5a in good overall yield. The hydroxy group in 5a was transformed into a p-nitrobenzenesulfonate (nosyl) group to yield 6a. According to the β-lactam formation method,4b the cyclization of 6a was carried out with LiHMDS as a base to afford the desired indolenine β-lactam 7a in 27% yield.6 Surprisingly, the cyclization of 6a using NaHCO3 at 40 °C provided 7a in higher yield (49%).6 Next, the cross metathesis of the obtained N-allyl β-lactam 7a with allyltrimethylsilane and Grubbs 2nd generation catalyst7 gave the corresponding allylsilane 8 in moderate yield as an inseparable mixture of E/Z isomers (E/Z = 4:1).
N-(3-Hydroxypropyl) β-lactam 9 was also synthesized from 2 in a manner similar to 7a (Scheme 3). The N-alkylation of 2 with 3-siloxypropyl bromide gave 3b in 77% yield. The deprotection of O-allyl and Boc groups followed by O-nosylation gave 6b. The β-lactam cyclization of 6b using LiHMDS was found to give the desired β-lactam 7b in the same level of yield as that of 7a.6 Unfortunately, the cyclization of 6b using NaHCO3 resulted in a complex mixture, and no desired product was observed. The TBDPS group in 7b was removed with TBAF, leading to the cyclization precursor 9 as an inseparable mixture of its tautomer, N,O-acetal 9'.
With requisite precursors 8 and 9 now in hand, their cyclizations were next examined (Schemes 5 and 6). Upon the treatment of indolenine β-lactam 8 with TBAF, intramolecular allylation occurred to give a mixture of the desired tertacyclic indoline β-lactam syn-10 and anti-10 in 24% and in 19% yield, respectively (Scheme 5). The intramolecular imine allylation of 8 did not take place under Lewis acidic conditions (BF3·OEt2, CH2Cl2, –78 °C to rt). These epimeric products were separable by silica gel column chromatography, and the relative stereochemistry of the diastereomers was determined by NOESY correlations (Figure 2). It revealed that the geometry of allylsilane in 8 does not appear to influence the relative stereoselectivity of products 10. Interestingly, the elimination of the 4-(trimethylsilyl)but-2-enyl group from β-lactam in 8 was not detected, indicating that intramolecular allylation (path a, Scheme 5) was much faster than the loss of the 4-(trimethylsilyl)but-2-enyl group (path b).
Next, the cyclization of aldehyde derived from 9 was examined to synthesize tetracyclic indolenine β-lactam 11 by using an enamine nature of 2-methylindolenine (Scheme 6). Treatment of a mixture of tautomers 9 and 9' with 1.2 equiv of Dess-Martin periodinane in CH2Cl2 smoothly led to an alcohol 11 having a 7-membered ring in 31% yield as a single diastereomer. In this reaction, both of tautomers 9 and 9' were consumed, and none of its epimer and the corresponding aldehyde and ketone derived from product 11 were observed. This transformation involves oxidation with concomitant tautomerization of the imine moiety and intramolecular enamine aldol reaction in a one-pot manner. Other oxidation conditions (TEMPO, NaClO, KBr, NaHCO3 aq., CH2Cl2, rt; SO3·py, Et3N, DMSO, CH2Cl2, rt; IBX, EtOAc, 80 °C; PCC, MS 4A, NH4OAc, CH2Cl2, rt) gave inferior results. The relative stereochemistry of 11 was determined by 1H NMR and NOESY analyses, as depicted in Figure 3. Thus, the present process serves as a simple and mild synthetic route for obtaining the potentially useful indolenine with requisite functionality for the construction of chartellamide core scaffold (Figure 1).8
In conclusion, we have developed two synthetic approaches to the functionalized tetracyclic indoline and indolenine β-lactam derivatives using the amphiphilic reactivity of 2-methylindolenine. The results of our study should serve as a potentially useful method for the synthesis of chartelline marine alkaloids. Our study also revealed that the β-lactam formation of the nosyl N-allyl hydroxamic acid derivative proceeded under mild conditions (NaHCO3, THF, 40 °C).
EXPERIMENTAL
General Techniques.
All reactions were monitored by thin-layer chromatography (TLC) on 0.25 mm silica gel coated glass plates 60F254 (Merck, #1.05715). Preparative thin-layer chromatographic separations were carried out on 0.5 mm silica gel plates 60F254 (Merck, #1.05744). Silica gel 60N (spherical, neutral, particle size 63-210 µm, Kanto Chemical Co., Inc., #37565-84) was used for open-column chromatography. Silica gel 60N (spherical, neutral, particle size 40-50 µm, Kanto Chemical Co., Inc., #37563-84) and silica gel 60 (spherical, particle size 40-50 µm, Kanto Chemical Co., Inc., #37562-84) were used for flash column chromatography. Dehydrated THF, Et2O and CH2Cl2 were purchased from Kanto Chemical Co., Inc. Infrared spectra (IR) were recorded on a JASCO FT/IR-4100 type A spectrophotometer and reported in wave number (cm–1). Proton nuclear magnetic resonance (1H NMR) spectra were recorded on a Varian Gemini-2000 (300 MHz) or a Bruker ARX-400 (400 MHz). NMR samples were dissolved in CDCl3, CD3OD, or C6D6, and chemical shifts were reported in ppm relative to the residual undeuterated solvent (CDCl3 as δ = 7.26, CD3OD as δ = 3.30, or C6D6 as δ = 7.15). 1H NMR data were reported as follows: chemical shifts, integration, multiplicity (s = singlet, d = doublet, t = triplet, q = quartet, br = broadened, m = multiplet), coupling constant(s), and assignment. Carbon nuclear magnetic resonance (13C NMR) spectra were recorded on a Varian Gemini-2000 (75 MHz) or a Bruker ARX-400 (100 MHz). NMR samples were dissolved in CDCl3, CD3OD, or C6D6, and chemical shifts were reported in ppm relative to the solvent (CDCl3 as δ = 77.0, CD3OD as δ = 49.0, or C6D6 as δ = 128.0). Elemental analyses were performed by the Analytical Laboratory of the Graduate School of Bioagricultural Sciences, Nagoya University. Melting points (Mp) were recorded on a Yanaco MP-S3 melting point apparatus and are not corrected. High resolution mass spectra (HRMS) were recorded on an Applied Biosystems Mariner ESI-TOF spectrometer and are reported in m/z.
Amide 2: To a solution of N-Boc indole-3-acetic acid 1 (6.15 g, 21.3 mmol) in CH2Cl2 (500 mL) were added AllylO-NH2·HCl (2.83 g, 25.8 mmol), Et3N (3.57 mL, 25.8 mmol) and EDC·HCl (3.84 g, 20.0 mmol). After being stirred at room temperature for 2 h, the reaction was quenched with H2O (500 mL), and the resulting mixture was extracted with CH2Cl2 (300 mL x 3). The combined extracts were washed with H2O (500 mL), 0.01 M NaOH aq. (300 mL x 2) and brine (500 mL), dried over Na2SO4, and concentrated under reduced pressure. The resulting residue was purified by silica gel column chromatography (AcOEt:hexane = 2:3) to afford amide 2 (6.08 g, 88%) as a white solid.
Mp 101-103 °C; IR (film) νmax 3186, 1731, 1657 cm–1; 1H NMR (CDCl3, 300 MHz) δ 1.69 (9H, s, -Boc), 2.56 (3H, s, -Me), 3.64 (2H, s, -CH2-), 4.30 (2H, d, J = 6 Hz, -CH2-CH=CH2), 5.19 (1H, d, J = 17 Hz, -CH=CHAHB), 5.20 (1H, d, J = 11 Hz, -CH=CHAHB), 5.84 (1H, m, -CH=CH2), 7.22 (1H, td, J = 8, 1 Hz, indole), 7.27 (1H, t, J = 8 Hz, indole), 7.42 (1H, d, J = 8 Hz, indole), 8.20 (1H, dd, J = 8, 1 Hz, indole), 8.21 (1H, brs, -NH); 13C NMR (CDCl3, 100 MHz) δ 14.1, 28.2, 30.0, 77.2, 84.1, 110.2, 115.5, 117.7, 121.0, 122.9, 124.1, 128.9, 131.8, 135.5, 135.7, 150.4, 167.6; Anal. Calcd for C19H24N2O4: C, 66.27; H, 7.02; N, 8.13. Found: C, 66.24; H, 6.90; N, 8.11.
N-Allylamide 3a: A dried two-necked flask was charged with N2, and NaH (60% dispersion in mineral oil, 0.86 g, 29.6 mmol) was added. The mineral oil was removed by washing with hexane (5 mL x 3) and DMF (300 mL) was added in the flask. After being stirred at 0 °C, to the resulting solution were added a solution of amide 2 (6.08 g, 17.7 mmol) in DMF (50 mL), allyl bromide (2.56 mL, 29.6 mmol), and TBAI (0.654 g, 1.77 mmol). After being stirred for 26 h at 4 °C, the reaction was quenched with H2O (700 mL), and the resulting mixture was extracted with AcOEt (500 mL x 2). The combined extracts were washed with H2O (1 L x 2) and brine (1 L), dried over Na2SO4, and concentrated under reduced pressure. The resulting residue was purified by silica gel column chromatography (AcOEt:hexane = 1:10 to 1:9 to 1:5 to 1:4 to 1:2 to 1:1) to afford N-allylamide 3a (5.20 g, 76%) as a colorless oil.
IR (film) νmax 1729, 1669 cm–1; 1H NMR (CDCl3, 300 MHz) δ 1.67 (9H, s, -Boc), 2.56 (3H, s, -Me), 3.84 (2H, s, -CH2-CO), 4.26 (2H, dt, J = 6, 1 Hz, -CH2-CH=CH2), 4.38 (2H, dt, J = 6, 1 Hz, -CH2-CH=CH2), 5.20 (1H, ddt, J = 10, 1.5, 1 Hz, -CH=CH2), 5.24 (1H, ddt, J = 17, 1.5, 1 Hz, -CH=CH2), 5.35 (1H, ddt, J = 10, 1.5, 1 Hz, -CH=CH2), 5.38 (1H, ddt, J = 17, 1.5, 1 Hz, -CH=CH2), 5.85 (1H, ddt, J = 17, 10, 6 Hz, -CH=CH2), 5.98 (1H, ddt, J = 17, 10, 6 Hz, -CH=CH2), 7.18 (1H, td, J = 7, 1.5 Hz, indole), 7.22 (1H, td, J = 7, 1.5 Hz, indole), 7.46 (1H, dd, J = 7, 1.5 Hz, indole), 8.09 (1H, dd, J = 7, 1.5 Hz, indole); 13C NMR (CDCl3, 100 MHz) δ 14.3, 28.2, 28.6, 49.4, 75.8, 83.5, 111.8, 115.3, 118.0, 118.3, 120.7, 122.5, 123.4, 129.8, 131.3, 132.2, 135.0, 135.6, 150.6, 172.2; Anal. Calcd for C22H28N2O4: C, 68.73; H, 7.34; N, 7.29. Found: C, 68.76; H, 7.36; N, 7.29.
N-Allylhydroxamic acid 4a: To a solution of N-allyl amide 3a (5.20 g, 13.5 mmol) in MeCN (108 mL) and H2O (27 mL) were added Pd(OAc)2 (303 mg, 1.35 mmol), PPh3 (1.41 g, 5.41 mmol), HCO2H (4.43 mL, 118 mmol), and Et3N (16.3 mL, 118 mmol) and the resulting mixture was heated at 40 °C. After being stirred at 40 °C for 7 h, the reaction was diluted with AcOEt (200 mL). The resulting mixture was washed with H2O (200 mL x 2) and brine (200 mL), dried over Na2SO4, and concentrated under reduced pressure. The resulting residue was purified by silica gel column chromatography (AcOEt:hexane = 1:1) to afford N-allylhydroxamic acid 4a (4.23 g, 91%) as a yellow solid.
Mp 152-153 °C; IR (film) νmax 3171, 1729, 1611 cm–1; 1H NMR (CD3OD, 400 MHz) δ 1.67 (9H, s, -Boc), 2.53 (3H, s, -Me), 3.87 (2H, s, -CH2-CO), 4.20 (2H, d, J = 6 Hz, -CH2-CH=CH2), 5.18 (1H, dd, J = 10, 1.5 Hz, -CH=CHAHB), 5.22 (1H, dd, J = 17, 1.5 Hz, -CH=CHACHB), 5.83 (1H, ddt, J = 17, 10, 6 Hz, -CH=CH2), 7.15 (1H, td, J = 7, 1.5 Hz, indole), 7.19 (1H, td, J = 7, 1.5 Hz, indole), 7.46 (1H, dd, J = 7, 1.5 Hz, indole), 8.06 (1H, dd, J = 7, 1.5 Hz, indole); 13C NMR (CD3OD, 100 MHz) δ 14.4, 28.5, 29.1, 52.3, 84.9, 113.5, 116.2, 118.5, 119.2, 123.5, 124.4, 131.4, 133.1, 136.2, 137.1, 152.1, 173.3; Anal. Calcd for C19H24N2O4: C, 66.26; H, 7.02; N, 8.13. Found: C, 66.29; H, 7.00; N, 8.13.
Indole 5a: To a solution of N-allylhydroxamic acid 4a (4.23 g, 12.3 mmol) in CH2Cl2 (369 mL) was added TFA (41 mL) at 0 °C, and the resulting solution was stirred at 0 °C for 24 h. The mixture was diluted with toluene and concentrated under reduced pressure. The residue was purified by silica gel column chromatography (AcOEt:hexane = 3:2) to afford indole 5a (2.60 g, 87%) as a colorless oil.
IR (film) νmax 3401, 2917, 1622, 1464, 1241 cm–1; 1H NMR (CD3OD, 300 MHz) δ 2.36 (3H, s, -Me), 3.86 (2H, s, -CH2-), 4.19 (2H, brd, J = 6 Hz, -CH2-CH=CH2), 5.13 (lH, brd, J = 10 Hz, -CH=CHAHB), 5.20 (lH, brd, J = 17 Hz, -CH=CHAHB), 5.72-5.90 (1H, m, -CH=CH2), 6.92 (1H, t, J = 7 Hz, indole), 6.98 (lH, t, J = 7 Hz, indole), 7.21 (1H, d, J = 7 Hz, indole), 7.45 (lH, d, J = 7 Hz, indole); 13C NMR (CD3OD, 75 MHz) δ 11.5, 29.3, 52.1, 105.2, 111.3, 118.4, 118.9, 119.6, 121.4, 130.2, 133.3, 134.4, 137.1, 174.8. Anal. Calcd for C14H16N2O2: C, 68.83; H, 6.60; N, 11.47. Found: C, 68.77; H, 6.70; N, 11.43.
Sulfonate 6a: To a stirred solution of hydroxamic acid 5a (116 mg, 0.475 mmol) and Et3N (0.131 mL, 0.952 mmol) in CH2Cl2 (4.8 mL) was added p-NsCl (106 mg, 0.476 mmol). After being stirred for 5 min, the reaction mixture was directly purified by silica gel column chromatography (AcOEt:hexane= 1:5 to 1:2) to afford sulfonate 6a (193 mg, 95%) as a orange amorphous solid.
lH NMR (CDCl3, 400 MHz) δ 2.25 (3H, s, -Me), 3.61 (2H, s, -CH2-), 4.36 (2H, brd, J = 5 Hz, -CH2-CH=CH2), 5.20 (lH, brd, J = 15 Hz, -CH2CH=CHAHB), 5.23 (lH, brd, J = 8 Hz, -CH2CH=CHAHB), 5.69-5.81 (lH, m, -CH2-CH=CH2), 7.04 (lH, t, J = 7 Hz, indole), 7.10 (lH, t, J = 7 Hz, indole), 7.18 (lH, d, J = 7 Hz, indole), 7.24 (lH, d, J = 7 Hz, indole), 7.86 (1H, brs, NH of indole), 8.02 (2H, d, J = 9 Hz, p-Ns), 8.12 (2H, d, J = 9 Hz, p-Ns).
N-Allyl β-lactam 7a: The freshly prepared sulfonate 6a (147 mg, 0.342 mmol) was dissolved in THF (34 mL) with NaHCO3 (58 mg, 0.68 mmol). The mixture was heated at 40 °C with stirring for 24 h. The solution was allowed to cool to room temperature and diluted with AcOEt, washed with H2O (x 2) and brine, dried over Na2SO4, and concentrated. The residue was purified by silica gel column chromatography (AcOEt:hexane = 1:2 to 1:1) to afford N-allyl β-lactam 7a (37.8 mg, 49%) as a colorless oil.
IR (film) νmax 3321, 2926, 1762, 1586, 1459 cm–1; 1H NMR (CDCl3, 400 MHz) δ 2.35 (3H, s, -Me), 3.23 (lH, d, J = 15 Hz, -CHAHB-), 3.28 (1H, d, J = 15 Hz, -CHAHB-), 3.43 (1H, dd, J = 15, 7 Hz, -CHCHD-CH=CH2), 3.82 (lH, dd, J = 15, 6 Hz, -CHCCHD-CH=CH2), 4.92 (1H, dd, J = 17, 1 Hz, -CH2CH=CHEHF), 5.00 (1H, dd, J = 10, 1 Hz, -CH2CH=CHEHF), 5.48-5.59 (lH, m, -CH2-CH=CH2), 7.26 (lH, t, J = 7 Hz, indolenine), 7.38 (IH, d, J = 7 Hz, indolenine), 7.41 (lH, t, J = 7 Hz, indolenine), 7.52 (lH, d, J = 7 Hz, indolenine); 13C NMR (CDCl3, 100 MHz) δ 15.1, 44.4, 45.9, 68.6, 120.3, 120.8, 122.2, 126.1, 130.1, 130.3, 134.1, 154.0, 165.4, 180.6; HRMS (FAB) (M+H)+ ca1cd for C14H15ON2 227.1184, found 227.1163.
N-(4-Silyl-2-butenyl) β-lactam 8: To a solution of N-allyl β-lactam 7a (10.0 mg, 0.044 mmol) and allyltrimethylsilane (0.021 mL, 0.132 mmol) in CH2Cl2 (0.44 mL) was added Grubbs 2nd generation catalyst (2.0 mg, 2.4 µmol) and the resulting mixture was refluxed. After being stirred at that temperature for 16.5 h, the reaction was concentrated under reduced pressure. The resulting residue was purified by preparative TLC (AcOEt:hexane = 1:1) to afford N-(4-silyl-2-butenyl) β-lactam 8 (6.2 mg, 45%, E/Z = 4:1 determined by 1H NMR analysis) as a yellow oil.
IR (film) νmax 1764 cm–1; 1H NMR (CDCl3, 400 MHz) δ –0.12 (1.8H, s, -SiC(CH3)), –0.09 (7.2H, s, -SiC(CH3)), 1.04-1.19 (0.4H, m, Si-CH2-), 1.27 (1.6H, d, J = 8 Hz, Si-CH2-), 2.34 (3H, s, -Me), 3.18 (0.8H, d, J = 15 Hz, -CHAHB), 3.20-3.27 (0.4H, m, -CHAHB), 3.23 (0.8H, d, J = 15 Hz, -CHAHB), 3.37 (0.8H, dd, J = 15, 8 Hz, N-CHCHD), 3.43-3.48 (0.2H, m, N-CHCHD), 3.78 (0.8H, dd, J = 15, 8 Hz, N-CHCHD), 3.81-3.87 (0.2H, m, N-CHCHD), 4.93-5.02 (0.2H, m, -NCH2-CH=CH-), 4.96 (0.8H, ddd, J = 15, 8, 7 Hz, -NCH2-CH=CH-), 5.26 (0.8H, dt, J = 15, 8 Hz, -CH=CH-CH2-Si), 5.44 (0.2H, q, J = 9.5 Hz, -CH=CH-CH2-Si), 7.25 (1H, t, J = 7 Hz, indolenine), 7.37 (1H, t, J = 7 Hz, indolenine), 7.40 (1H, d, J = 7 Hz, indolenine), 7.51 (1H, d, J = 7 Hz, indolenine); 13C NMR (CDCl3, 100 MHz) δ –2.0, 15.0, 18.5, 22.7, 37.9, 43.9, 45.7, 68.6, 118.2, 120.1, 120.8, 122.2, 126.1, 130.1, 134,0, 134.6, 165.2, 180.9; HR-MS (ESI, positive): calcd. For C18H24N2ONaSi (M+Na), 335.1550; found, 335.1545.
N-Siloxypropylamide 3b: To a solution of 3-(tert-butyldiphenylsiloxy)propyl bromide (2.19 g, 5.81 mmol) in acetone (19.4 mL) were added amide 2 (2.00 g, 5.81 mmol), K2CO3 (3.20 g, 23.2 mmol) and KI (96 mg, 0.58 mmol) at room temperature. After the reaction mixture was refluxed for 35 h, the reaction was quenched with saturated NH4Cl aq. (40 mL), and the resulting mixture was extracted with AcOEt (30 mL x 3). The combined extracts were washed with H2O (100 mL x 2) and brine (100 mL), dried over Na2SO4, and concentrated under reduced pressure. The resulting residue was purified by silica gel column chromatography (AcOEt:hexane = 1:12 to 1:5 to 1:3) to afford N-siloxypropylamide 3b (2.86 g, 77%) as a colorless oil.
IR (film) νmax 1730, 1666 cm–1; 1H NMR (CDCl3, 300 MHz) δ 1.06 (9H, s, -SiC(CH3)3), 1.68 (9H, s, -Boc), 1.88 (2H, tt, J = 7, 6 Hz, -CH2CH2CH2-), 2.55 (3H, s, -Me), 3.70 (2H, t, J = 6 Hz, -CH2-), 3.80 (2H, t, J = 7 Hz, -CH2- ), 3.80 (2H, s, -CH2-CO-), 4.36 (2H, dt, J = 6, 1 Hz, -CH2-CH=CH2), 5.34 (1H, dd, J = 10, 1 Hz, -CH=CHAHB), 5.37 (1H, dd, J = 17, 1 Hz, -CH=CHAHB), 5.98 (1H, ddt, J = 17, 10, 6 Hz -CH=CH2), 7.17 (1H, td, J = 7, 2 Hz, indole), 7.23 (1H, td, J = 7, 2 Hz, indole), 7.34-7.46 (6H, m, TBDPS), 7.45 (1H, d, J = 7, 2 Hz, indole), 7.63-7.69 (4H, m, TBDPS), 8.10 (1H, dd, J = 7, 2 Hz, indole); 13C NMR (CDCl3, 75 MHz) δ 14.2, 19.1, 26.7, 28.2, 28.5, 29.8, 43.4, 61.4, 75.2, 83.5, 112.0, 115.4, 118.0, 120.7, 122.5, 123.4, 127.7, 129.7, 129.9, 131.4, 133.7, 135.1, 135.6, 135.7, 150.8, 172.1; Anal. Calcd for C38H48N2O5Si: C, 71.20; H, 7.55; N, 4.37. Found: C, 71.20; H, 7.45; N, 4.25.
N-Siloxypropylhydroxamic acid 4b: To a solution of N-siloxypropylamide 3b (2.99 g, 4.67 mmol) in MeCN (37.4 mL) and H2O (9.3 mL) were added Pd(OAc)2 (104 mg, 0.467 mmol), PPh3 (0.489 g, 1.87 mmol), HCO2H (1.53 mL, 40.6 mmol) and Et3N (5.62 mL, 40.6 mmol), and the resulting mixture was heated at 40 °C. After being stirred at 40 °C for 23 h, the reaction was quenched with H2O (20 mL), and the resulting mixture was extracted with AcOEt (30 mL x 3). The combined extracts were washed with H2O (60 mL x 2) and brine (60 mL), dried over Na2SO4, and concentrated under reduced pressure. The resulting residue was purified by silica gel column chromatography (AcOEt:hexane = 1:5 to 1:3 to 1:2) to afford N-siloxypropylhydroxamic acid 4b (2.33 g, 83%) as an orange amorphous solid.
IR (film) νmax 3177, 1730, 1608 cm–1; 1H NMR (CD3OD, 300 MHz) δ 1.00 (9H, s, -SiC(CH3)3), 1.67 (9H, s, -Boc), 1.88 (2H, tt, J = 7, 6 Hz, -CH2CH2CH2-), 2.50 (3H, s, -Me), 3.68 (2H, t, J = 6 Hz, N-CH2-), 3.76 (2H, t, J = 7 Hz, O-CH2- ), 3.82 (2H, s, -CH2-CO-), 7.10 (1H, td, J = 7, 2 Hz, indole), 7.18 (1H, td, J = 7, 2 Hz, indole), 7.32-7.41 (6H, m, TBDPS), 7.42 (1H, d, J = 7, 2 Hz, indole), 7.61-7.66 (4H, m, TBDPS), 8.06 (1H, dd, J = 7, 2 Hz, indole); 13C NMR (CDCl3, 100 MHz) δ 14.2, 19.1, 26.8, 28.3, 28.7, 30.3, 46.8, 64.1, 83.4, 112.3, 115.3, 118.3, 122.5, 123.3, 127.8, 128.0, 130.2, 132.1, 135.0, 135.4, 135.6, 150.7, 170.5; Anal. Calcd for C35H44N2O5Si: C, 69.97; H, 7.38; N, 4.66. Found: C, 69.99; H, 7.28; N, 4.71.
Hydroxamic acid 5b: To a solution of N-siloxypropylhydroxamic acid 4b (1.00 g, 1.66 mmol) in CH2Cl2 (45.4 mL) was added TFA (10.0 mL) at 0 °C. After being stirred at 0 °C for 5.3 h, the reaction mixture was diluted with toluene and concentrated under reduced pressure. The resulting residue was purified by silica gel column chromatography (AcOEt:hexane = 1:3) to afford hydroxamic acid 5b (0.679 g, 82%) as an orange amorphous solid.
IR (film) νmax 3195, 1615 cm–1; 1H NMR (CD3OD, 300 MHz) δ 1.01 (9H, s, -SiC(CH3)3), 1.87 (2H, tt, J = 7, 6 Hz, -CH2CH2CH2-), 2.34 (3H, s, -Me), 3.68 (2H, t, J = 6 Hz, N-CH2-), 3.74 (2H, t, J = 7 Hz, O-CH2- ), 3.81 (2H, s, -CH2-CO-), 6.89 (1H, td, J = 8, 1 Hz, indole), 6.97 (1H, td, J = 8, 1 Hz, indole), 7.21 (1H, dd, J = 8, 1 Hz, indole), 7.33-7.43 (6H, m, TBDPS), 7.44 (1H, dd, J = 8, 1 Hz, indole), 7.63 (4H, d, J = 7 Hz, TBDPS); 13C NMR (CD3OD, 100 MHz) δ 11.6, 20.0, 27.4, 29.4, 30.9, 46.6, 62.7, 105.3, 111.2, 118.9, 119.5, 121.3, 128.8, 130.2, 130.8, 134.3, 134.9, 136.6, 137.0, 174.6; Anal. Calcd for C30H36N2O3Si: C, 71.96; H, 7.25; N, 5.59. Found: C, 71.95; H, 7.11; N, 5.48.
Sulfonate 6b: To a solution of hydroxamic acid 5b (150 mg, 0.30 mmol) and Et3N (83 µL, 0.60 mmol) in CH2Cl2 (1.5 mL) was added p-NsCl (66.0 mg, 0.30 mmol) at 0 °C. After being stirred at 0 °C for 5 min, the reaction mixture was directly purified by silica gel column chromatography (AcOEt:hexane = 1:5 to 1:2) to afford sulfonate 6b (192 mg, 94%) as a yellow oil.
IR (film) νmax 3403, 1702, 1535, 1191 cm–1; 1H NMR (CDCl3, 400 MHz) δ 1.01 (9H, s, -SiC(CH3)3), 1.86 (2H, brs, -CH2CH2CH2-), 2.23 (3H, s, -Me), 3.65 (2H, s, -CH2-CO-), 3.65 (2H, brs, O-CH2-), 4.00 (2H, brs, N-CH2-), 7.04 (1H, t, J = 7 Hz, indole), 7.12 (1H, t, J = 7 Hz, indole), 7.22 (1H, d, J = 7 Hz, indole), 7.25 (1H, d, J = 7 Hz, indole), 7.37-7.47 (6H, m, TBDPS), 7.63 (4H, d, J = 7 Hz, TBDPS), 7.94 (2H, d, J = 8 Hz, p-Ns), 7.97 (2H, d, J = 8 Hz, p-Ns); 13C NMR (CDCl3, 100 MHz) δ 11.7, 19.1, 26.8, 29.4, 29.6, 50.2, 60.6, 103.1, 110.5, 117.6, 119.7, 121.5, 123.7, 127.7, 127.8, 128.0, 129.8, 130.6, 133.0, 133.3, 134.9, 135.4, 139.2, 150.8, 173.8; HR-MS (ESI, positive): calcd. For C36H39N3O7NaSSi (M+Na), 708.2170; found, 708.2139.
N-Siloxypropyl β-lactam 7b: To a solution of sulfonate 6b (63.8 mg, 0.093 mmol) in THF (3.2 mL) was added LiHMDS (1.0 M in THF, 0.10 mL) at –78 °C. The mixture was stirred for 10 min, then allowed to warm to room temperature. After being stirred at room temperature for 1 h, the reaction mixture was poured into cold saturated NH4Cl aq. (10 mL), and the resulting mixture was extracted with AcOEt (5 mL x 3). The combined extracts were washed with H2O (20 mL x 2) and brine (20 mL), dried over Na2SO4, and concentrated under reduced pressure. The resulting residue was purified by silica gel column chromatography (AcOEt:hexane = 3:4) to afford N-siloxypropyl β-lactam 7b (11.6 mg, 26%) as a yellow oil.
IR (film) νmax 3314, 1764 cm–1; 1H NMR (C6D6, 400 MHz) δ 1.07 (9H, s, -SiC(CH3)3), 1.30 (2H, tt, J = 7, 6 Hz, -CH2CH2CH2-), 1.85 (3H, s, -Me), 2.55 (1H, d, J = 14 Hz, CHAHB), 2.62 (1H, d, J = 14 Hz, CHAHB), 2.93 (2H, td, J = 7, 4 Hz, O-CH2-), 3.32 (2H, td, J = 6, 4 Hz, N-CH2- ), 6.75 (1H, dd, J = 8, 1 Hz, indolenine), 6.86 (1H, t, J = 8 Hz, indolenine), 7.04 (1H, td, J = 8, 1 Hz, indolenine), 7.20-7.25 (6H, m, TBDPS), 7.56 (1H, d, J = 8 Hz, indolenine), 7.63-7.66 (4H, m, TBDPS); 13C NMR (C6D6, 100 MHz) δ 14.5, 19.3, 27.0, 31.0, 38.8, 45.7, 61.1, 68.8, 121.1, 122.3, 126.0, 128.5, 129.96, 129.97, 130.1, 134.0, 135.4, 135.89, 135.91, 155.1, 165.0, 180.6; Anal. Calcd for C30H34N2O2Si: C, 74.65; H, 7.10; N, 5.80. Found: C, 74.63; H, 7.07; N, 5.75.
Alcohol 9 and N,O-Acetal 9': To a solution of N-siloxypropyl β-lactam 7b (26.9 mg, 0.056 mmol) in THF (1.1 mL) was added a mixture of TBAF (1.0 M in THF, 0.056 mL) and AcOH (3.1 µL, 0.056 mmol). After being stirred at room temperature for 6 h, the reaction was quenched with H2O (2 mL) and the resulting mixture was neutralized by saturated NaHCO3 aq. and extracted with AcOEt (3 mL x 7). The combined extracts were dried over Na2SO4 and concentrated under reduced pressure. The resulting residue was purified by silica gel column chromatography (AcOEt:MeOH = 20:1) to afford alcohol 9 and N,O-acetal 9' (9.9 mg, 72%, 9:9' = 75:25) as a yellow oil.
Alcohol 9: IR (film) νmax 3339, 1752 cm–1; 1H NMR (CD3OD, 400 MHz) δ 1.47 (2H, tt, J = 7, 6 Hz, -CH2CH2CH2-), 2.38 (3H, s, -Me), 3.04 (1H, dt, J = 14, 7 Hz, NCHAHB), 3.12 (1H, dt, J = 14, 7 Hz, NCHAHB), 3.30 (1H, d, J = 15 Hz, -CHCHDCO), 3.42 (1H, d, J = 15 Hz, -CHCHDCO), 3.44 (2H, td, J = 6, 1.5 Hz, -CH2OH), 7.31 (1H, td, J = 7, 1.5 Hz, indolenine), 7.44 (1H, td, J = 7, 1.5 Hz, indolenine), 7.47 (1H, dd, J = 7, 1.5 Hz, indolenine), 7.53 (1H, dd, J = 7, 1.5 Hz, indolenine); 13C NMR (CD3OD, 100 MHz) δ 14.9, 31.8, 40.2, 46.3, 60.1, 70.3, 121.2, 124.0, 127.8, 131.5, 135.5, 154.5, 168.5, 183.6; HR-MS (ESI, positive): calcd. For C14H16N2O2Na (M+Na), 267.1104; found, 267.1120.
N,O-Acetal 9': 1H NMR (CD3OD, 400 MHz) δ 1.50 (3H, s, Me), 1.51 (1H, ddq, J = 13, 3, 2.5 Hz, -CH2CHAHBCH2-), 1.81 (1H, dtdd, J = 14, 13, 5, 3 Hz, -CH2CHAHBCH2-), 2.46 (1H, td, J = 14, 3 Hz, -NCHCHD-), 3.04 (1H, d, J = 15 Hz, -CHEHFCO-), 3.42 (1H, d, J = 15 Hz, -CHEHFCO-), 3.67 (1H, dq, J = 13, 2.5 Hz, -OCHGHH-), 3.75 (1H, ddt, J =14, 5, 2.5 Hz, -NCHCHD-), 3.96 (1H, td, J = 13, 2.5 Hz, -OCHGHH-), 6.64 (1H, dd, J = 8, 1 Hz, aromatic), 6.76 (1H, td, J = 8, 1 Hz, aromatic), 7.11 (1H, dd, J = 8, 1 Hz, aromatic), 7.14 (1H, td, J = 8, 1 Hz, aromatic).
β-Lactam syn-10 and anti-10: To a solution of N-(4-silyl-2-butenyl) β-lactam 8 (16.6 mg, 0.053 mmol) in THF (5.3 mL) was added TBAF (1.0 M in THF, 0.053 mL) at 0 °C. After being stirred at 0 °C for 10 min, the reaction was quenched with H2O (10 mL) and the resulting mixture was extracted with AcOEt (10 mL x 3). The combined extracts were washed with brine, dried over Na2SO4 and concentrated under reduced pressure. The resulting residue was purified by preparative TLC (AcOEt:hexane = 1:3) to afford syn-10 (3.1 mg, 24%) as a colorless oil and anti-10 (2.4 mg, 19%) as a colorless oil. Stereochemistries of syn-10 and anti-10 were determined by NOESY correlations.
syn-10: IR (film) νmax 3340, 1757 cm–1; 1H NMR (CDCl3, 400 MHz) δ 1.22 (3H, s, -Me), 2.98 (1H, td J = 9, 8 Hz -CH-CH=CH2), 3.07 (1H, dd, J = 11, 8 Hz, -NCHAHB-), 3.16 (1H, d, J = 17 Hz, -CHCHD-CO-), 3.18 (1H, d, J = 17 Hz, -CHCHD-CO-), 3.37 (1H, dd, J = 11, 9 Hz, -NCHAHB-), 4.20 (1H, brs, -NH), 5.13 (1H, dd, J = 17, 2 Hz, -CH=CHEHF), 5.19 (1H, dd, J = 10, 2 Hz, -CH=CHEHF), 5.77 (1H, ddd, J = 17, 10, 9 Hz -CH=CH2), 6.69 (1H, dd, J = 7, 1 Hz, aromatic), 6.87 (1H, td, J = 7, 1 Hz, aromatic), 7.14 (1H, td, J = 7, 1 Hz, aromatic), 7.35 (1H, dd, J = 7, 1 Hz, aromatic); 13C NMR (CDCl3, 100 MHz) δ 16.7, 44.4, 47.9, 58.4, 70.1, 72.0, 111.1, 118.8, 120.0, 124.8, 128.2, 130.1, 134.4, 148.7, 175.6; HR-MS (ESI, positive): calcd. For C15H16N2ONa (M+Na), 263.1154; found, 263.1164.
anti-10: IR (film) νmax 3354, 1759 cm–1; 1H NMR (CDCl3, 400 MHz) δ 1.37 (3H, s, -Me), 2.65 (1H, ddd J = 11, 8, 6 Hz, -CH-CH=CH2), 2.73 (1H, t, J = 11 Hz, -NCHAHB-), 3.26 (1H, d, J = 17 Hz, -CHCHD-CO-), 3.60 (1H, d, J = 17 Hz, -CHCHD-CO-), 3.79 (1H, dd, J = 11, 6 Hz, -NCHAHB-), 5.22 (1H, dd, J = 18, 1 Hz, -CH=CHEHF), 5.25 (1H, dd, J = 10, 1 Hz, -CH=CHEHF), 5.77 (1H, ddd, J = 18, 10, 8 Hz -CH=CH2), 6.63 (1H, dd, J = 8, 1 Hz, aromatic), 6.81 (1H, t, J = 8 Hz, aromatic), 7.17 (1H, td, J = 8, 1 Hz, aromatic), 7.31 (1H, d, J = 8 Hz, aromatic); 13C NMR (CDCl3, 100 MHz) δ 22.2, 43.2, 50.4, 58.5, 71.8, 73.3, 109.6, 118.9, 119.4, 124.4, 126.3, 130.4, 134.5, 163.3, 177.0; HR-MS (ESI, positive): calcd. For C15H17N2O (M+H), 241.1335; found, 241.1347.
Indolenine 11: To a solution of 9 and 9' (8.1 mg, 0.033 mmol) in CH2Cl2 (0.66 mL) was added Dess-Martin periodinane (16.9 mg, 0.040 mmol). After being stirred at room temperature for 1 h, the reaction was diluted with Et2O (1.5 mL) and the resulting mixture was quenched with saturated NaHCO3 aq. (0.5 mL) and saturated Na2S2O3 aq. (0.5 mL). After being stirred for 30 min, H2O (3 mL) was added and the mixture was neutralized with saturated NH4Cl aq. The resulting mixture was extracted with AcOEt (3 mL x 3). The combined extracts were dried over Na2SO4 and concentrated under reduced pressure. The resulting residue was purified by preparative TLC (AcOEt:MeOH = 20:3) to afford indolenine 11 (2.5 mg, 31%, dr = >95:<5 determined by 1H NMR analysis) as a yellow oil.
IR (film) νmax 3348, 1761 cm–1; 1H NMR (CD3OD, 400 MHz) δ 1.84 (1H, ddt, J = 14, 3, 1.5 Hz, -NCH2CHAHBCH(OH)), 2.07 (1H, ddt, J = 14, 13, 3 Hz, -NCH2CHAHBCH(OH)), 2.60 (1H, ddt, J = 15, 13, 1.5 Hz, -NCHCHD), 2.96 (1H, dd, J = 13, 1.5 Hz, -CHEHFCH(OH)), 3.14 (1H, ddd, J = 13, 6, 1.5 Hz, -CHEHFCH(OH)), 3.33 (1H, d, J = 15 Hz, -CHGHHCO-), 3.50 (1H, d, J = 15 Hz, -CHGHHCO-), 3.55 (1H, dt, J = 15, 3 Hz, -NCHCHD), 4.29 (1H, ddt, J = 6, 3, 1.5 Hz, CH-OH), 7.29 (1H, td, J = 7, 1 Hz, indolenine), 7.42 (1H, td, J = 7, 1 Hz, indolenine), 7.47 (1H, dd, J = 7, 1 Hz, indolenine), 7.52 (1H, dd, J = 7, 1 Hz, indolenine); 13C NMR (CD3OD, 100 MHz) δ 36.4, 37.6, 37.7, 47.6, 65.3, 70.6, 121.3, 123.8, 127.7, 131.3, 137.0, 154.9, 169.7, 183.5; HR-MS (ESI, positive): calcd. For C14H14N2O2Na (M+Na), 265.0948; found, 265.0948.
ACKNOWLEDGEMENTS
This work was financially supported by a Grant-in-Aid for Scientific Research (B) and a Grant-in-Aid for Young Scientists (B) from MEXT, the Yamada Science Foundation, and the Nagase Science and Technology Foundation.
References
1. For reviews of β-lactam antibiotics, see: (a) A. H. Berks, Tetrahedron, 1996, 52, 331; CrossRef (b) M. Sunagawa and A. Sasaki, Heterocycles, 2001, 54, 497. CrossRef
2. (a) R. Kumar, S. Giri, and Nizamuddin, J. Agric. Food Chem., 1989, 37, 1094; CrossRef (b) R. J. Shah, N. R. Modi, M. J. Patel, L. J. Patel, B. F. Chauhan, and M. M. Patel, Med. Chem. Res., 2011, 20, 587. CrossRef
3. For chartelline marine alkaloids, see: (a) J. S. Carlé and C. Christophersen, J. Am. Chem. Soc., 1979, 101, 4012; CrossRef (b) J. S. Carlé and C. Christophersen, J. Org. Chem., 1980, 45, 1586; CrossRef (c) P. B. Holst, U. Anthoni, C. Christophersen, and P. H. Nielsen, J. Nat. Prod., 1994, 57, 997; CrossRef For isolation and structural determination of chartellamides A and B, see: (d) U. Anthoni, K. Bock, L. Chevolot, C. Larsen, P. H. Nielsen, and C. Christophersen, J. Org. Chem., 1987, 52, 5638. CrossRef
4. (a) T. Nishikawa, S. Kajii, and M. Isobe, Chem. Lett., 2004, 33, 440; CrossRef (b) T. Nishikawa, S. Kajii, and M. Isobe, Synlett, 2004, 2025. CrossRef
5. Related intermolecular reactions of 3,3-disubstituted 2-methylindolenines were reported. For alkylation of 2-substituted indolenine with Grignard reagent, see: (a) J. G. Rodríguez, A. Urrutia, J. E. de Diego, M. Paz Martínez-Alcazar, and I. Fonseca, J. Org. Chem., 1998, 63, 4332; CrossRef For enamine aldol reaction, see: (b) S. F. Vice, E. A. Gross, R. W. Friesen, and G. I. Dmitrienko, Tetrahedron Lett., 1982, 22, 829; CrossRef (c) S. F. Vice, R. W. Friesen, and G. I. Dmitrienko, Tetrahedron Lett., 1985, 26, 165. CrossRef
6. The indolenine β-lactam formation gave the desired compound 7 along with some unidentified products.
7. Cross metathesis to synthesize substituted allylsilanes, see: S. Thibaudeau and V. Gouverneur, Org. Lett., 2003, 5, 4891, and references therein. CrossRef
8. For the synthetic study on chartellamides A and B, see: J. L. Pinder and S. M. Weinreb, Tetrahedron Lett., 2003, 44, 4141. CrossRef