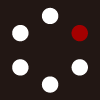
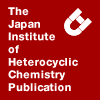
HETEROCYCLES
An International Journal for Reviews and Communications in Heterocyclic ChemistryWeb Edition ISSN: 1881-0942
Published online by The Japan Institute of Heterocyclic Chemistry
e-Journal
Full Text HTML
Received, 12th February, 2013, Accepted, 18th March, 2013, Published online, 28th March, 2013.
DOI: 10.3987/COM-13-S(S)1
■ Introduction of Heteroarene Functionality on the Bipedal-Thiol-Capped Gold Nanoparticle by Deprotonative C-H Coupling with Palladium Complex
Atsushi Sugie, Hiroki Yamauchi, Kei Miyamura, Kenta Kumazawa, Shota Tanaka, Kiyoshi Kanie, Atsushi Muramatsu, and Atsunori Mori*
Chemical Science & Technology, Graduate School of Engineering, Kobe University, 1-1 Rokkodai-cho, Nada-ku, Kobe 657-8501, Japan
Abstract
Gold nanoparticle stabilized by adsorption of bipedal thiol whose end is modified with bromoarene is prepared to undergo palladium-mediated deprotonative C-H arylation reaction. Heteroarenes are introduced onto the organic moiety of the nanoparticle.INTRODUCTION
Gold nanoparticle (AuNP) stabilized by adsorption of thiols has attracted considerable attention as a wide range of advanced materials.1,2 As the demand of AuNP increases as materials, precise modification by organic transformation on AuNP becomes a significant issue to introduce the functionality. Transition-metal-catalyzed cross-coupling reaction3 on AuNP can thus be a promising tool, which enables diversity-oriented construction of the functionalized AuNP.4 We have recently shown that Suzuki-Miyaura and Sonogashira coupling, which are cross-coupling employing a palladium complex, indeed, take place on the surface of AuNP, in which the aryl halide moiety is located at the end of alkanethiol. The reactions proceeded efficiently without fatal aggregation when 1,3-dithiol is employed for the ligand to improve stable adsorption to the gold atom that is tolerable to the reaction conditions of cross coupling, whereas the reaction with monodentate thiol has resulted in unsuccessful.5
On the other hand, we have been engaged in transition-metal-catalyzed deprotonative C-H arylation reactions at the C-H bond of thiophenes and thiazoles with aryl halides. We have revealed that lithium alkoxide6 and magnesium amide7 effectively deprotonate heteroarenes and couples with variety of organic halides catalyzed by transition metal showing advantages in step efficiency and operational simplicity compared with conventional cross-coupling reactions employing main-group organometallics such as boron, silicon, and tin. Accordingly, we considered that C-H arylation can also be employed for the modification protocol on AuNP as shown in Scheme 1. Herein, we describe that the C-H arylation reaction occurs at the bipedal-thiol capped AuNP and introduction of thiophene and thiazole moieties are performed on the AuNP surface.
RESULTS AND DISCUSSION
We first prepared the bipedal-thiol-capped AuNP. Bipedal thiol, whose terminal was modified by 4-bromophenoxy group, was chosen as a capping agent of AuNP.8 Synthesis of bipedal-thiol-capped AuNP 1 was carried out by treatment of a THF solution of HAuCl4·4H2O and the corresponding 1,3-dithiol with tBuNH2·BH3 as a reducing agent.9 TEM observation of the obtained AuNP 1 exhibited spherical nanoparticle with a diameter of 7.6±2.0 nm.
Palladium-catalyzed C-H arylation reaction with LiOtBu as a base was first employed to introduce a thiazole group as shown in Scheme 2. A THF solution of AuNP 1, benzothiazole, LiOtBu, and Pd(tBu3P)2 was stirred under reflux for 1 h. Unfortunately, it was found to disappear characteristic purple color of the solution derived from surface plasmon resonance1 suggesting unexpected aggregation of AuNP. However, the collected particle by the addition of ethanol to the solution was found to partially disperse to organic solvents such as THF and CHCl3. Nevertheless, the coupling reaction was found to take place on AuNP. Cleavage of the organic moiety from gold surface was carried out by treatment of the precipitated suspension with iodine10 in chloroform to afford 1,2-dithiolane 2 bearing a benzothiazole moiety. Analyses by 1H NMR and HRMS (ESI+) suggested conversion of the bromide into thiazole.
The similar reaction was carried out with several heteroarenes (Table 1). HRMS (ESI+) analysis of the organic moiety of the obtained precipitates suggested undergoing coupling of two thiazole derivatives and 2-methylthiophene with aryl bromide on AuNP albeit aggregation was also observed.
We then examined application of deprotonative C-H arylation reaction using magnesium amide as a base. We have been reported the reaction of thiophene with Knochel-Hauser base TMPMgCl·LiCl11 (TMP = 2,2,6,6-tetramethylpiperidine) induces the metalation at the C-H bond adjacent to the sulfur atom and following arylation of the metalated thiophene proceeded smoothly by transition-metal catalyzed cross coupling.7 With such reaction conditions, the reaction of thiophene with aryl bromide on AuNP was examined. The reaction procedure follows: A THF solution of TMPMgCl·LiCl was stirred with thiophene derivative at room temperature for 3 h to complete metalation. To the resulting solution were added AuNP 1, palladium complex with NHC ligand (PEPPSI-IPr), and additional THF. After stirring for 24 h at room temperature, the reaction was quenched by addition of aqueous NH4Cl. Ethanol was then added to the separated organic layer to form precipitates which were purified by iterative centrifugation and precipitation to give AuNP 3a. When the reaction was carried out by 3-hexylthiophene, which is known to be metalated at the 5-position regioselectively by treatment of TMPMgCl·LiCl,7 purple color of the mixture was observed throughout the reaction. The isolated nanoparticle obtained by the addition of ethanol was found to be redispersible to CHCl3 and THF. The result suggests that the bipedal-thiol-capped AuNP is tolerable to the reaction conditions of the coupling reaction despite the presence of highly reactive carbanion. Formation of the carbon-carbon bond was confirmed by comparison of 1H NMR spectra of AuNP 1 (A) and 3a (B) shown in Figure 1.12 The signal of bromophenoxy group of AuNP 1 at 6.75 ppm in the spectrum (A) disappeared in (B), suggesting that the reaction proceeded in high conversion. New signals at 7.46, 7.02, 6.86, 6.78 ppm, which are assigned as arylthiophene moiety, were observed in (B). Additional signals corresponding to the hexyl group were also observed at 2.59 and 0.86 ppm (See supporting information). Since TEM image of AuNP 3a showed spherical nanoparticles with an average diameter of 7.6±2.0 nm, no problematic morphological change of the nanoparticle was suggested to occur during the reaction.
The similar reaction was carried out with thiophene dendrimers 3T and 7T13 as shown in Table 2. The reactions proceeded smoothly to afford the corresponding AuNP 3b and 3c in high conversions, respectively. Conversion of the reaction to 3b and 3c was confirmed by 1H NMR comparing chemical shifts of the methylene group adjacent to the phenoxy group (See supporting information). TEM observation exhibited that the size of AuNP 3b and 3c were 7.3±1.2 and 7.9±1.4 nm, which were comparable with that of AuNP 1 (Table 3). It should be pointed out that introduction of bulky dendrons onto AuNP induced increase in mean interparticle distance compared to that of AuNP 1 in TEM observation. Although Advincula and co-workers recently reported synthesis of thiophene dendrimer-capped AuNPs, the thiophene-dendrimer moiety had been introduced into the capping thiol prior to the modification of AuNP.14 Notably, the present introduction protocol of thiophene dendron is coupling at the C-H bond of thiophene on the nanoparticle, the reaction of which is a complimentary pathway for the functionalization of gold nanoparticles. In contrast with the conventional top-down method to prepare functionalized AuNPs, bottom-up-type fabrication by the reaction at the bipedal-thiol-capped organic moiety would suit to the diversity-oriented AuNPs synthesis.
In summary, we have shown that introduction of thiophene moiety onto bipedal-thiol-capped gold nanoparticle was accomplished by C-H coupling reaction employing TMPMgCl·LiCl as deprotonating agent in the presence of a palladium complex. Although highly reactive carbanion was involved in the reaction system, the nanoparticle was tolerant toward aggregation. Thus, the established protocol would be a choice for the synthesis of thiophene-functionalized gold nanoparticles.
EXPERIMENTAL
General. 1H NMR (300 MHz) and 13C NMR (75 MHz) spectra were measured on Varian Gemini 300 as a CDCl3 solution unless noted. The chemical shifts were expressed in ppm with CHCl3 (7.26 ppm for 1H) or CDCl3 (77.0 ppm for 13C) as internal standards. IR spectra were recorded on PERKIN ELMER FT-IR Spectrometer SPECTRUM 1000. High resolution mass spectra (HRMS) were measured by JEOL JMS-700 MStation (EI) at the Graduate School of Material Science, Nara Institute of Science and Technology and JEOL JMS-T100LP AccuTOF LC-Plus (ESI). Transmission electron microscopic (TEM) observations were performed by using JEOL JEM-1230 BU and JEOL JEM-1200EX II with an acceleration voltage of 100 and 120 kV, respectively. TEM samples were prepared by dropping of the THF or toluene/hexane solution of gold nanoparticle (AuNP) on 150 mesh carbon coated copper grids, which were purchased from Okensyoji Co. Ltd. HAuCl4·4H2O was purchased from Tanaka Kikinzoku Kogyo Co. Ltd. Pd(tBu3P)2 and PEPPSI-IPr were purchased from Sigma-Aldrich Co. Ltd. TMPMgCl·LiCl was prepared by following the literature procedure11h and stored in the freezer as 1.0 M THF solution. THF was purchased from Kanto Chemical Co. Inc. as an anhydrous grade and stored in a Schlenk tube under nitrogen atmosphere. Other chemicals were purchased and used as such. Preparation of thiophene dendrimer 3T and 7T was performed by the method shown in the literature.13
Synthesis of dithiol-capped AuNP 1: To a screw-capped test tube equipped with a magnetic stirring bar were added HAuCl4·4H2O (41.2 mg, 0.1 mmol), the bipedal thiol (43.4 mg, 0.1 mmol) and 5 mL of THF and the resulting solution was stirred at 40 °C for 3 h. THF (5 mL) solution of tBuNH2·BH3 (8.7 mg, 0.1 mmol) was then added to the reaction mixture at 55 °C. After stirring for 0.5 h at 55 °C, EtOH was added to the solution to precipitate gold nanoparticle, which was centrifuged to isolate the dithiol-capped gold nanoparticle 1 (22.6 mg) as a dark brown powder.
C-H coupling reaction of benzothiazole with AuNP 1 in the presence of LiOtBu:
To a Schlenk tube equipped with a magnetic stirring bar were added AuNP 1 (60.0 mg) and 6 mL of THF at rt. The mixture was sonicated to form a homogeneous purple solution. To the resulting mixture were added benzothiazole (33 µL, 0.3 mmol), Pd(tBu3P)2 (15.3 mg, 0.03 mmol), and THF solution of LiOtBu (2.2 M, 135 µL). After stirring for 1 h under reflux, EtOH was added to the mixture to obtain precipitate (53.0 mg) as dark brown powder which did not dispersible to organic solvents such as CHCl3 and THF.
The precipitate was then subjected to the reaction with iodine to remove the organic moiety from the gold surface.10 The precipitate (53.0 mg) was added to 5 mL of THF to form suspension. To the resulting suspension was added I2 (53.0 mg). After stirring 30 minutes at rt, the mixture was centrifuged and the supernatant was collected. Concentration of the solution under reduced pressure left a crude mixture, which was passed through a silica gel column to leave the organic moiety of the precipitate as 1,2-dithiolane 2. The compound 2 was subjected to 1H NMR measurement as a CDCl3 solution suggesting that aryl bromide was coupled with benzothiazole to form the corresponding product (See below). Result of HRMS (ESI+) measurement of 2 also suggested formation of the corresponding product (Calcd for C27H36NOS3 [M+H] +: 486.1959; found: m/z 486.1961).
C-H coupling reaction of 5-{4-(trifluoromethyl)phenyl}thiazole with AuNP 1 in the presence of LiOtBu: The reaction was carried out in a similar manner as above using AuNP 1 (20 mg), 5-{4-(trifluoromethyl)phenyl}thiazole (22.9 mg, 0.1 mmol), THF solution of LiOtBu (2.2 M, 45 µL), Pd(tBu3P)2 (5.1 mg, 0.01 mmol) in 2 mL of cyclopentyl methyl ether. Treatment of the obtained nanoparticle with iodine was also carried out in the similar manner. Result of HRMS (ESI+) measurement of the obtained organic compound suggested formation of the corresponding dithiorane (Calcd for C30H37NOS3 [M+H] +: 580.1989; found: m/z 580.1989).
C-H coupling reaction of 2-methylthiophene with AuNP 1 in the presence of LiOtBu: The reaction was carried out in a similar manner as above using AuNP 1 (20 mg), 2-methylthiophene (9.7 µL, 0.1 mmol), THF solution of LiOtBu (2.2 M, 45 µL), Pd(tBu3P)2 (5.1 mg, 0.01 mmol) in 2 mL of cyclopentyl methyl ether. Treatment of the obtained nanoparticle with iodine was also carried out in the similar manner. Result of HRMS (ESI+) measurement of the obtained organic compound suggested formation of the corresponding dithiorane (Calcd for C25H36NaOS3 [M+Na]+: 471.1826; found: m/z 471.1823).
General procedure for C-H coupling of thiophenes with AuNP 1 in the presence of TMPMgCl·LiCl: To a Schlenk tube equipped with a magnetic stirring bar were added THF solution of TMPMgCl·LiCl (1 M, 0.12 mL, 0.12 mmol) and thiophene derivative (0.1 mmol). After stirring for 3 h at rt, AuNP 1 (20 mg), PEPPSI-IPr (8.2 mg, 0.012 mmol), and THF (2 mL) were added to the mixture. After stirring for 24 h at rt, aqueous NH4Cl was added to quench the reaction. EtOH was then added to the separated organic layer to precipitate AuNP 3a-c as dark brown powder. Measurement of the 1H NMR spectrum was carried out as a CDCl3 solution to estimate the conversion of aryl bromide to the corresponding thienylaryl group. In case that the resolution of the spectrum was insufficient, AuNP was subjected to the treatment of iodine in a similar manner to the case of the reaction of AuNP 1 with benzothiazole.
ACKNOWLEDGEMENTS
The authors thank Professors Yasukiyo Ueda and Hideto Minami of Kobe University, who kindly provided an opportunity for TEM observation.
References
1. For reviews: a) M.-C. Daniel and D. Astruc, Chem. Rev., 2004, 104, 293; CrossRef b) J. C. Love, L. A. Estroff, J. K. Kriebel, R. G. Nuzzo, and G. M. Whitesides, Chem. Rev., 2005, 105, 1103; CrossRef c) S. K. Ghosh and T. Pal, Chem. Rev., 2007, 107, 4797; CrossRef d) G. J. Hutchings, Chem. Commun., 2008, 1148. CrossRef
2. Selected examples: a) H. Ahn, A. Chandekar, B. Kang, C. Sung, and J. E. Whitten, Chem. Mater., 2004, 16, 3274; CrossRef b) M. Minamoto, M. M. Matsushita, and T. Sugawara, Polyhedron, 2005, 24, 2263; CrossRef c) Z. Zhang, F. Wang, F. Chen, and G. Shi, Mater. Lett., 2006, 60, 1039; CrossRef d) M. Tanaka, R. Fujita, and H. Nishide, Polymer, 2007, 48, 5884; CrossRef e) G. Zotti and B. Vercelli, Chem. Mater., 2008, 20, 397. CrossRef
3. F. Diederich and P. J. Stang, Eds. Metal-Catalyzed Cross-Coupling Reaction; Wiley-VCH: Weinheim, 1998. CrossRef
4. a) K. J. Watson, J. Zhu, S. T. Nguyen, and C. A. Mirkin, J. Am. Chem. Soc., 1999, 121, 462; CrossRef b) J. P. Collman, N. K. Debaraj, and C. E. D. Chidsey, Langmuir, 2004, 20, 1051; CrossRef c) C. Xue, G. Arumugam, K. Palaniappan, S. A. Hackney, H. Liu, and J. Liu, Chem. Commun., 2005, 1055; CrossRef d) W. J. Sommer and M. Weck, Langmuir, 2007, 23, 11991; CrossRef e) E. Boisselier, L. Salmon, J. Ruiz, and D. Astruc, Chem. Commun., 2008, 5788; CrossRef f) M. Taniguchi, N. Nojima, K. Yokota, J. Terao, K. Sato, N. Kambe, and T. Kawai, J. Am. Chem. Soc., 2006, 128, 15062; CrossRef g) M. Tanaka, R. Fujita, and H. Nishide, J. Nanosci. Nanotechnol., 2009, 9, 634; CrossRef h) Y. Fang, Y. Li, H. Xi, and M. Sun, Langmuir, 2010, 26, 7737; CrossRef i) C. I. Müller and C. Lambert, Langmuir, 2011, 27, 5029. CrossRef
5. a) A. Sugie, K. Kumazawa, T. Hatta, K. Kanie, A. Muramatsu, and A. Mori, Chem. Lett., 2011, 40, 1450; CrossRef See also b) A. Sugie, T. Somete, K. Kanie, A. Muramatsu, and A. Mori, Chem. Commun., 2008, 33, 3882; CrossRef c) A. Sugie, T. Hatta, K. Kanie, A. Muramatsu, and A. Mori, Chem. Lett., 2009, 38, 562; CrossRef d) A. Sugie, T. Yamanaka, K. Kumazawa, T. Hatta, Y. Ueda, K. Kanie, A. Muramatsu, and A. Mori, Chem. Lett., 2010, 39, 319. CrossRef
6. S. Tamba, Y. Okubo, S. Tanaka, D. Monguchi, and A. Mori, J. Org. Chem., 2010, 75, 6998. CrossRef
7. a) S. Tamba, K. Shono, A. Sugie, and A. Mori, J. Am. Chem. Soc., 2011 133, 9700; CrossRef b) S. Tanaka, S. Tamba, D. Tanaka, A. Sugie, and A. Mori, J. Am. Chem. Soc., 2011, 133, 16734; CrossRef c) A. Mori, J. Synth. Org. Chem. Jpn., 2011, 69, 1202; CrossRef d) S. Tanaka, D. Tanaka, A. Sugie, and A. Mori, Tetrahedron Lett., 2012, 53, 1173; CrossRef e) S. Tamba, S. Mitsuda, F. Tanaka, A. Sugie, and A. Mori, Organometallics, 2012, 31, 2263; CrossRef f) S. Tanaka, S. Tamba, A. Sugie, and A. Mori, Heterocycles, 2012, 86, 255. CrossRef
8. Synthesis of the bipedal thiol 1 was carried out in a manner similar to the bipedal thiol bearing iodophenoxy group shown in Ref. 5. See SI for details.
9. N. Zheng, J. Fan, and G. D. Stucky, J. Am. Chem. Soc., 2006, 128, 6550. CrossRef
10. A. C. Templeton, M. J. Hostetler, C. T. Craft, and R. W. Murray, J. Am. Chem. Soc., 1998, 120, 1906. CrossRef
11. a) A. Krasovskiy, V. Krasovskaya, and P. Knochel, Angew. Chem., Int. Ed., 2006, 45, 2958; CrossRef b) W. Lin, O. Baron, and P. Knochel, Org. Lett., 2006, 8, 5673; CrossRef c) M. Mosrin and P. Knochel, Org. Lett., 2008, 10, 2497; CrossRef d) G. C. Clososki, C. J. Rohbogner, and P. Knochel, Angew. Chem., Int. Ed., 2007, 46, 7681; CrossRef e) C. J. Rohbogner, G. C. Clososki, and P. Knochel, Angew. Chem., Int. Ed., 2008, 47, 1503; CrossRef f) F. M. Piller and P. Knochel, Org. Lett., 2009, 11, 445; CrossRef g) C. R. Hauser and H. G. Walker, J. Am. Chem. Soc., 1947, 69, 295; CrossRef h) C. R. Hauser and F. C. Frostick, J. Am. Chem. Soc., 1949, 71, 1350; CrossRef i) H.-Q. Do and O. Daugulis, J. Am. Chem. Soc., 2009, 131, 17052. CrossRef
12. Assignment of the signals was carried out by comparison of 1H NMR spectrum of 5-(4-methoxylphenyl)-3-hexylthiophene which was prepared by the similar reaction using TMPMgCl•LiCl (See Ref 7).
13. S. Tanaka, D. Tanaka, G. Tatsuta, K. Murakami, S. Tamba, A. Sugie, and A. Mori, Chem. Eur. J., 2013, 19, 1658. CrossRef
14. S. Deng, T. M. Fulghum, G. Krueger, D. Patton, J.-Y. Park, and R. C. Advincula, Chem. Eur. J., 2011, 17, 8929. CrossRef