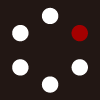
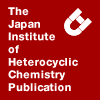
HETEROCYCLES
An International Journal for Reviews and Communications in Heterocyclic ChemistryWeb Edition ISSN: 1881-0942
Published online by The Japan Institute of Heterocyclic Chemistry
e-Journal
Full Text HTML
Received, 25th June, 2013, Accepted, 16th July, 2013, Published online, 26th July, 2013.
DOI: 10.3987/COM-13-S(S)48
■ Diversity Oriented Approach to Spirobarbituric Acid Derivatives via a [2+2+2] Cycloaddition and Diels‒Alder Reaction as Key Steps
Sambasivarao Kotha* and Rashid Ali
Department of Chemistry, Indian Institute of Technology Bombay, Powai, Mumbai - 400076, India
Abstract
Spirobarbituric acid derivatives are assembled via a [2+2+2] cycloaddition and the Diels-Alder (DA) reaction as key steps. A transient diene 13 containing spiribarbituric acid suitable for DA reaction has been generated in situ from the sultine derivative 12, which in turn was obtained by the usage of rongalite.Construction of spirocyclics1 is of substantial interest because of their presence in biologically relevant molecules and also their applications in medicinal chemistry. The term ‘spirocyclanes’ was introduced by Baeyer in 1900, to allocate a bicyclic hydrocarbon allied by a single carbon. The intricacy of these ring structures is signified by the quaternary carbon center2 and two fused rings. Due to the tetrahedral nature of a spiro center the rings are positioned approximately perpendicular to each other and the biological activities of these compounds is due to the asymmetric nature of the chiral spiro center.3 Several natural4 and non-natural5 products contain the spiro linkage6 as a core structural element.
Barbituric acid derivatives are extensively used in the engineering of textiles,7 food, nutrition, plastics,8 polymers,9 leather industries and pharmaceuticals.10 Recent reports indicate that some of the barbituric acid derivatives show antimicrobial, antifungal, antiviral, antitumor activities.11 Barbiturates exert a wide range of pharmacological properties such as hypnotics sedatives, anticonvulsants, general anesthesia and anxiolytic effects.
In addition to their pharmacological applications, they are also found to be useful building blocks in assembling supramolecular structures through noncovalent interactions.12 Medicinal properties of barbiturates are entirely dependent on the structure and the potency of these drugs dramatically dependent on the side chains added to the rings. Some biologically important barbiturates are shown in Figure 1.13
Several methods are available in the literature for the synthesis of spirocyclics. However, here we have conceived a new and simple approach to generate a spiro centre containing barbituric acid. Our strategy incorporates a number of diversity points and tolerates a variety of functional groups. To this end, we plan to synthesize hybrid molecules containing a spiro center and the barbituric acid by using an atom-economic [2+2+2] cycloaddition14 and the DA reaction as key steps. In this regard, we started our strategy with an inexpensive and readily available starting material such as 1,3-dimethylbarbituric acid 7. Rongalite has been used to generate a transient diene intermediate 13 involving a [2+2+2] cycloaddition reaction. The diene 13 is useful to design a variety of complex barbituric acid derivatives by application of the DA chemistry.
Our journey towards the synthesis of spirobarbituric acid derivatives started with the dipropargylation of commercially available 1,3-dimethylbarbituric acid 7 by using propargyl bromide and K2CO3 as a base in MeCN at room temperature (rt) to give the desired dipropargylated product 8 in 78% yield. Later, the compound 8 was subjected to a [2+2+2] cycloaddition reaction with the 2-butyne-1,4-diol in presence of Wilkinson’s catalyst and a catalytic amount of titanium isopropoxide15 [Ti(OiPr)4] in ethanol to give the desired diol 10 in 62% yield along with a small amount of the self-dimerised product 9 (7%) (Scheme 1). The structure of the compound 9 was confirmed on the basis of 1H NMR, 13C NMR, IR (sharp peak at 3306, C-H str of alkyne) and further confirmed by mass spectral data [M+Na]+ 487.1589.
Having the diol 10 in hand, it was converted to the dibromide 11 in 81% yield by treating with the phosphorous tribromide (PBr3) in benzene at rt. Subsequently, the dibromide 11 was converted to the sultine derivative 12 in 87% yield by treating with the rongalite in presence of phase–transfer catalyst such as tetrabutylammonim bromide (TBAB) in dimethylformamide (DMF) (Scheme 1). Later, the sultine 12 was reacted with various dienophiles in a [4+2] cycloaddition fashion to deliver the corresponding DA adducts under toluene reflux conditions. Thus, the transient diene 13 generated in situ was trapped with different dienophiles to give the DA adducts. Since these DA adducts were contaminated with aromatized product, we did not isolate the intermediate DA adducts and they were directly treated with the 2,3-dichloro-5,6-dicyano-1,4-benzoquinone (DDQ) under toluene reflux condition to deliver the desired aromatic products in good yields (Table 1).
In summary, we have demonstrated a highly efficient method for the synthesis of various spirobarbituric acid derivatives by using an inexpensive and a commercially available starting material 1,3-dimethylbarbituric acid and rongalite. By using this strategy we are able to produce several complex molecules in a diversity oriented16 manner. In view of the diversity points incorporated in our strategy, this methodology can deliver a library of barbituric acid derivatives containing a spiro centre. Our strategy is highly atom economic and green, which involves rongalite for sultine formation and the sultine derivative 12 acts as diene equivalent in the DA reaction which can be trapped with various dienophiles to deliver a number of intricate barbiturates by varying the dienophile component.
EXPERIMENTAL
All the commercial reagents were used without purification and reactions involving air sensitive reagents or catalysts were performed in degassed solvents. Transfer of moisture sensitive materials were accomplished by using standard syringe-septum techniques and the reactions were maintained under nitrogen atmosphere. All the reactions were monitored by employing TLC technique by using appropriate mixture of ethyl acetate and petroleum ether for development. Yields refer to the isolated yields after column chromatography technique. Wilkinson’s catalyst was purchased from Sigma Aldrich. Infrared (IR) spectra were recorded on Nicolet Impact-400 FT IR spectrometer in KBr. Proton nuclear magnetic resonance (1H NMR, 400 MHz) spectra and carbon nuclear magnetic resonance (13C NMR, 100 MHz) spectra were recorded on a Bruker spectrometer. The high-resolution mass measurements were carried out by using electrospray ionization (ESI), (Q-ToF) spectrometer. Melting points were recorded on a Büchi B-545 melting point apparatus.
Preparation of compound 8: To a solution of compound 7 (2 g, 12.8 mmol) in MeCN (20 mL), K2CO3 (8.84 g, 64.10 mmol) and tetrabutylammoniun hydrogen sulphate (TBAHS) (217 mg, 0.64 mmol) were added at rt. Then, the reaction mixture was stirred at rt for 15 min. Later, the propargyl bromide (3.7 mL, 48.31 mmol) was added slowly and the stirring was continued at rt for 14 h. At the conclusion of reaction (TLC monitoring), excess amount of K2CO3 was filtered through sintered funnel, solvent was concentrated at reduced pressure and product was purified by silica gel column chromatography (60% EtOAc-petroleum ether) to give white solid compound 8 (2.33 g, 78%). The 1H and 13C spectra matched with literature reported spectra.17
General procedure for a [2+2+2] cycloaddition: A solution of compound 8 (2 g, 8.62 mmol) and 2-butyne-1,4-diol (3.7 g, 43.02 mmol) in dry EtOH (50 mL) was degassed with nitrogen for 15 min later, Wilkinson’s catalyst (199 mg, 2.5 mol%) and Ti(OiPr)4 (488 mg, 20 mol%) was added and the reaction mixture was refluxed for 18 h. At the conclusion of the reaction (TLC monitoring), the solvent was concentrated at reduced pressure and the crude product was purified by silica gel column chromatography (50% EtOAc-petroleum ether) to give a white solid compound 9 (208 mg, 7%) and continued elution with (80% EtOAc-petroleum ether) gave a white solid compound 10 (1.7 g, 62%).
Compound 9: White solid; Mp 217-218 °C; Rf = 0.60 (silica gel, 50% EtOAc-petroleum ether); 1H NMR (400 MHz, CDCl3) δ = 2.01 (t, J = 2.44 Hz, 1H), 2.98 (d, J = 2.40 Hz, 2H), 3.17 (s, 6H), 3.18 (s, 2H), 3.32 (s, 6H), 3.51 (s, 4H), 6.81-6.85 (m, 2H), 7.03 (d, J = 7.72 Hz, 1H); 13C NMR (100 MHz, CDCl3) δ = 27.94, 28.55, 29.23, 43.58, 44.54, 45.09, 56.44, 58.60, 72.07, 78.27, 124.31, 124.98, 127.96, 133.69, 139.14, 140.57, 150.41, 151.46, 170.38, 171.88; IR (KBr): υmax = 1681, 1750, 2400, 2934, 2961, 3020 cm-1; “accurate mass” electrospray ionization (ESI), (Q-ToF) calculated for C24H24N4NaO6 [M+Na]+ 487.1588, found: 487.1589.
Compound 10: White solid; Mp 202-204 °C; Rf = 0.20 (silica gel, 50% EtOAc-petroleum ether); 1H NMR (400 MHz, CD3OD) δ = 3.23 (s, 6H), 3.50 (s, 4H), 4.62 (br, 2H) 4.63 (s, 4H), 7.19 (s, 2H); 13C NMR (100 MHz, CD3OD) δ = 29.28, 45.08, 57.68, 63.08, 125.00, 139.55, 140.82, 153.21, 174.34; IR (KBr): υmax = 1676, 2038, 2225, 2835, 2945, 3391 cm-1; “accurate mass” electrospray ionization (ESI), (Q-ToF) calculated for C16H18N2NaO5 [M+Na]+ 341.1108, found: 341.1104.
Preparation of 11: To a solution of compound 10 (1 g, 3.14 mmol) in dry benzene (25 mL), was added a solution of PBr3 (0.9 mL, 9.4 mmol) in benzene (10 mL) dropwise by using dropping funnel at 0 °C. The reaction mixture was stirred at rt for 15 h. At the conclusion of the reaction (TLC monitoring), the reaction mixture was poured into ice-cooled water then, aq. layer was extracted with CH2Cl2, the solvent was concentrated at reduced pressure and the crude product was purified by silica gel column chromatography (50% EtOAc-petroleum ether) to give 11 as a white solid (1.13 g, 81%).
Mp 212-214 °C; Rf = 0.56 (silica gel, 35% EtOAc-petroleum ether); 1H NMR (400 MHz, CDCl3) δ = 3.31 (s, 6H), 3.35 (s, 4H), 4.63 (s, 4H), 7.21 (s, 2H), 13C NMR (100 MHz, CDCl3) δ = 29.16, 30.31, 44.01, 56.10, 126.77, 135.92, 141.05, 151.24, 171.78; IR (KBr): υmax = 1671, 1744, 2939, 3027 cm-1; “accurate mass” electrospray ionization (ESI), (Q-ToF) calculated for C16H1779Br81BrN2O3 [M+H]+ 444.9600, found: 444.9580 other isotope peaks are 442.9599 and 446.9563.
Preparation of 12: To a solution of compound 11 (300 mg, 0.68 mmol) and tetrabutylammonium bromide (TBAB) (218 mg, 0.68 mmol) in DMF (10 mL) was added rongalite at 0 °C. The reaction mixture was stirred at 0 °C for 3 h and at rt for another 3 h. At the conclusion of the reaction (TLC monitoring), the compound was extracted with EtOAc and the organic layer was washed with water 4-5 times to remove excess of DMF. The solvent was concentrated at reduced pressure and the crude product was purified by silica gel column chromatography (80% EtOAc-petroleum ether) to give sultine18 12 as a white solid (205 mg, 87%).
Mp 270-272 °C; Rf = 0.40 (silica gel, 50% EtOAc-petroleum ether); 1H NMR (400 MHz, CDCl3) δ = 3.30 (s, 3H), 3.31 (s, 3H), 3.50 (d, J = 15.24 Hz, 1H), 3.59 (s, 4H), 4.41 (d, J = 15.33 Hz, 1H), 4.92 (d, J = 13.52 Hz, 1H), 5.23 (d, J = 13.48 Hz, 1H), 7.08 (s, 1H), 7.12 (s, 1H); 13C NMR (100 MHz, CDCl3) δ = 29.04, 29.08, 43.93, 56.14, 57.58, 63.67, 121.65, 125.23, 125.78, 133.39, 139.46, 140.26, 151.22, 171.74, 171.87; IR (KBr): υmax = 754, 1104, 1675, 1744, 2851, 2927 cm-1; “accurate mass” electrospray ionization (ESI), (Q-ToF) calculated for C16H16N2NaO5S [M+Na]+ 371.0672, found: 371.0671.
General procedure for DA reaction of 12 and subsequent aromatization: A solution of compound 12 and 1.5 equivalents of dienophiles in toluene (20 mL) was heated at 110-120 °C for 12-15 h. Then, the solvent was concentrated at reduced pressure and the crude product was purified by silica gel column chromatography (50% EtOAc-petroleum ether) to afford the DA adducts. Later, aromatization of the DA adduct was carried out with 4 equivalents of DDQ in toluene (20 mL) by refluxing for 24 h. The solvent was removed at reduced pressure and the crude product was purified by column chromatography (50% EtOAc-petroleum ether) to afford the aromatized product.
Compound 14: White solid; Mp 221-223 °C; Rf = 0.62 (silica gel, 50% EtOAc-petroleum ether); 1H NMR (400 MHz, CDCl3) δ = 3.34 (s, 6H), 3.76 (s, 4H), 3.95 (s, 6H), 7.71 (s, 2H), 8.16 (s, 2H); 13C NMR (100 MHz, CDCl3) δ = 29.26, 43.90, 52.83, 56.74, 123.30, 128.25, 129.97, 133.44, 141.88, 151.34, 168.37, 171.74; IR (KBr): υmax = 1678, 1720, 2851, 2953, 3022 cm-1; “accurate mass” electrospray ionization (ESI), (Q-ToF) calculated for C22H20N2NaO7 [M+Na]+ 447.1163, found: 447.1166.
Compound 15: Yellow solid; Mp 208-209 °C; Rf = 0.68 (silica gel, 50% EtOAc-petroleum ether); 1H NMR (400 MHz, CDCl3) δ = 3.36 (s, 6H), 3.80 (s, 4H), 7.05 (s, 2H), 7.87 (s, 2H), 8.54 (s, 2H); 13C NMR (100 MHz, CDCl3) δ = 29.33, 44.04, 56.73, 124.87, 128.34, 128.68, 134.99, 140.23, 143.22, 151.29, 171.67, 184.96; IR (KBr): υmax = 1591, 1679, 2846, 2925, cm-1; “accurate mass” electrospray ionization (ESI), (Q-ToF) calculated for C22H16N2NaO5 [M+Na]+ 411.0951, found: 411.0959.
Compound 16: White solid; Mp 290-291 °C; Rf = 0.64 (silica gel, 50% EtOAc-petroleum ether); 1H NMR (400 MHz, CDCl3) δ = 3.35 (s, 6H), 3.61 (s, 4H), 3.80 (s, 4H), 7.07 (s, 2H); 13C NMR (100 MHz, CDCl3) δ = 29.35, 35.89, 38.60, 44.05, 56.22, 110.64, 124.49, 124.78, 141.69, 151.24, 171.76; IR (KBr): υmax = 1671, 2395, 2961, 3019 cm-1; “accurate mass” electrospray ionization (ESI), (Q-ToF) calculated for C22H16N6NaO3 [M+Na]+ 435.1176, found: 435.1175.
Compound 17: Yellow solid; Mp 250-252 °C; Rf = 0.60 (silica gel, 50% EtOAc-petroleum ether); 1H NMR (400 MHz, CDCl3) δ = 2.93 (d, J = 12.88 Hz, 2H), 3.21 (d, J = 14.48 Hz, 2H), 3.32 (s, 6H), 3.44 (t, J = 2.28 Hz, 2H), 3.54 (s, 4H), 6.83 (d, J = 7.32 Hz, 2H), 7.03 (s, 2H), 7.30-7.34 (m, 1H), 7.37-7.40 (m, 2H); 13C NMR (100 MHz, CDCl3) δ = 29.16, 29.17, 30.34, 40.49, 44.07, 56.55, 123.76, 126.90, 128.84, 129.32, 131.85, 134.34, 138.73, 151.51, 171.76, 172.18, 178.79; IR (KBr): υmax = 1598, 1717, 1772, 2851, 2928, 3021 cm-1; “accurate mass” electrospray ionization (ESI), (Q-ToF) calculated for C26H23N3NaO5 [M+Na]+ 480.1530, found: 480. 1528.
ACKNOWLEDGEMENTS
R. A. thanks University Grants Commission, New Delhi for the award of a research fellowship. S. K. thanks the Department of Science and Technology for the award of a J. C. Bose fellowship. We also thank DST-New Delhi for the financial support.
References
1. (a) M. Sannigrahi, Tetrahedron, 1999, 55, 9007; CrossRef (b) D. J. Halt, W. D. Barker, P. R. Jenkins, J. Panda, and S. Ghosh, J. Org. Chem., 2000, 65, 482; CrossRef (c) S. Kotha, E. Manivannan, T. Ganesh, N. Sreenivasachary, and A. C. Deb, Synlett, 1999, 1618; CrossRef (d) S. Kotha and K. Mandal, Tetrahedron Lett., 2004, 45, 1391; CrossRef (e) S. Kotha, A. C. Deb, and S. Chattopadhyay, Lett. Org. Chem., 2006, 3, 39; CrossRef (f) S. Kotha, M. K. Dipak, and S. M. Mobin, Tetrahedron, 2011, 67, 2543; (g) S. Kotha and A. C. Deb, Indian J. Chem., 2008, 47B, 1120; (h) S. Kotha and E. Manivannan, ARKIVOC, 2003, 67; CrossRef (i) S. Kotha, A. C. Deb, and R. V. Kumar, Bioorg. Med. Chem., 2005, 15, 1039; CrossRef (j) P. Singh and K. Paul, J. Heterocycl. Chem., 2006, 43, 607. CrossRef
2. T. Hudlicky and J. W. Reed, The Way of Synthesis; Wiley-VCH: Weinheim, 2007, p. 98.
3. R. Pradhan, M. Patra, A. K. Behera, B. K. Mishra, and R. K. Behera, Tetrahedron, 2006, 62, 779. CrossRef
4. (a) A. V. Rama Rao, A. K. Singh, B. V. Rao, and K. M. Reddy, Tetrahedron Lett., 1993, 34, 2665; CrossRef (b) J. A. Marshall, Prog. Chem. Nat. Prod., 1974, 31, 283; (c) W. D. Fessner, H. Prinzbach, and G. Rihs, Tetrahedron Lett., 1983, 24, 5857; CrossRef (d) Y. Kita, K. Higuchi, Y. Yoshida, K. Lio, S. Kitagaki, K. Ueda, A. Shuji, and H. Fujioka, J. Am. Chem. Soc., 2001, 123, 3214; CrossRef (e) J. D. White, J. F. Ruppert, M. A. Avery, S. Torii, and J. Nokami, J. Am. Chem. Soc., 1981, 103, 1813; CrossRef (f) C. Patil, A. Roy, and D. Mukherjee, Tetrahedron, 2002, 58, 1773; CrossRef (g) B. K. Trivedi, A. Holmes, T. S. Purchase, A. D. Essenurg, K. L. Hamelehle, B. R. Krause, M. K. S. Hes, and R. L. Stanfield, Bioorg. Med. Chem. Lett., 1995, 5, 2229; CrossRef (h) T. R. Kelly, N. Ohashi, R. J. Armstrong-Chorig, and S. H. Bell, J. Am. Chem. Soc., 1986, 108, 7100; CrossRef (i) G. Mehta and D. Subrahmanyam, Tetrahedron Lett., 1987, 28, 479; CrossRef (j) Y. Kita, K. Higuchi, Y. Yoshida, K. Lio, S. Kitagaki, S. Akai, and H. Fujioka, Angew. Chem. Int. Ed., 1999, 38, 683; CrossRef (k) Y. Takemoto, S. Kuraoka, T. Ohru, Y. Yonetoku, and C. Iwata, Tetrahedron, 1997, 53, 603; CrossRef (l) A. Murali, S. Sato, and T. Masamune, Tetrahedron Lett., 1981, 22, 1033; CrossRef (m) B. M. Trost, K. Hiroi, and H. Holy, J. Am. Chem. Soc., 1975, 97, 5873; CrossRef (n) G. L. Lange, W. L. Orram, and D. J. Wallace, Can. J. Chem., 1978, 56, 1628. CrossRef
5. (a) M. Thommen and R. Keese, Synlett, 1997, 231; CrossRef (b) D. Kuck, Top. Curr. Chem., 1998, 196, 168; CrossRef (c) W.-D. Fessner, H. Prinzbach, and C. Rihs, Tetrahedron Lett., 1983, 24, 5857; CrossRef (d) T. Widjaja, L. Fitjer, K. Meindl, and R. Herbst-Irmer, Tetrahedron, 2008, 64, 4304; CrossRef (e) L. Fitjer, U. Klages, D. Wehle, M. Giersig, N. Schormann, W. Clegg, D. S. Stephenson, and G. Binsch, Tetrahedron, 1988, 44, 405; CrossRef (f) D. Kuck and H. Bögge, J. Am. Chem. Soc., 1986, 108, 8107; CrossRef (g) D. Kuck, Chem. Ber., 1994, 127, 409; CrossRef (h) M. Thommen and R. Keese, Synlett, 1997, 231; CrossRef (i) R. Keese, Chem. Rev., 2006, 106, 4787. CrossRef
6. S. Kotha, A. C. Deb, K. Lahiri, and E. Manivannan, Synthesis, 2009, 165. CrossRef
7. R. Bartzatt, J. Pharm. Biomed. Anal., 2002, 29, 909. CrossRef
8. D. Thetford, A. P. Chorlton, and J. Hardman, Dyes Pigments., 2003, 59, 185. CrossRef
9. (a) R. Andreu, J. Garin, J. Orduna, R. Alcala, and B. Villacumpa, Org. Lett., 2003, 5, 3143; CrossRef (b) N. D. McClenaghan, C. Absalon, and D. M. Bassani, J. Am. Chem. Soc., 2003, 125, 13004. CrossRef
10. (a) M. Meusel, A. Ambrozak, T. K. Hecker, and M. Gutschow, J. Org. Chem., 2003, 68, 4684; (b) H. Brunner, K. P. Ittner, D. Lunz, S. Schmatloch, T. Schmidt, and M. Zabel, Eur. J. Org. Chem., 2003, 855; CrossRef (c) D. M. Neumann, B. S. Jursic, and K. L. Martin, Tetrahedron Lett., 2002, 43, 1603; CrossRef (d) B. S. Jursic and D. M. Neumann, Tetrahedron Lett., 2002, 42, 8435. CrossRef
11. H. M. Faidallah and K. A. Khan, J. Fluorene Chem., 2012, 142, 96.
12. (a) L. J. Prins, K. A. Jolliffe, R. Hulst, P. Timmerman, and D. N. Reinhoudt, J. Am. Chem. Soc., 2000, 122, 3617; CrossRef (b) P. Timmerman and L. J. Prins, Eur. J. Org. Chem., 2001, 3191. CrossRef
13. (a) J. C. Ruble, A. R. Hurd, T. A. Johnson, D. A. Sherry, M. R. Barbachyn, P. L. Toogood, G. L. Bundy, D. R. Graber, and G. M. Kamilar, J. Am. Chem. Soc., 2009, 131, 3991; CrossRef (b) L. Lomlim, J. Einsiedel, F. W. Heinemann, K. Meyer, and P. Gmeiner, J. Org. Chem., 2008, 73, 3608; CrossRef (c) A. Renard, J. Lhomme, and M. Kotera, J. Org. Chem., 2002, 67, 1302. CrossRef
14. (a) S. Kotha and E. Manivannan, J. Chem. Soc., Perkin Trans 1., 2001, 2543; CrossRef (b) S. Kotha, K. Mohanraja, and S. Durani, Chem. Commun., 2000, 1909; (c) S. Saito and Y. Yamamoto, Chem. Rev., 2000, 100, 2901; CrossRef (d) S. Kotha, E. Brahmachary, and K. Lahiri, Eur. J. Org. Chem., 2005, 4741; CrossRef (e) S. Kotha and N. Sreenivasacharry, Bioorg Med. Chem. Lett., 2000, 10, 1413; CrossRef (f) S. Kotha and E. Brahmachary, Tetrahedron Lett., 1997, 38, 3561; CrossRef (g) R. Grigg, R. Scott, and P. Stevenson, J. Chem. Soc., Perkin Trans 1., 1988, 1357. CrossRef
15. (a) A. Fürstner and K. Langemann, J. Am. Chem. Soc., 1997, 119, 9130; CrossRef (b) N. Buschmann, N. A. Ruckert, and S. Blechert, J. Org. Chem., 2002, 67, 4325; CrossRef (c) F. A. Davis and B. J. Yang, J. Am. Chem. Soc., 2005, 127, 8398. CrossRef
16. (a) S. Kotha, M. Mandal, A. Tiwari, and S. M. Mobin, Chem. Eur. J., 2006, 12, 8024; CrossRef (b) S. Kotha and K. Lahri, Eur. J. Org. Chem., 2007, 1221; CrossRef (c) S. Kotha and P. Khedkar, Eur. J. Org. Chem., 2009, 730; (d) S. Kotha, S. Misra, N. G. Krishna, and D. Nagaraju, Heterocycles, 2010, 80, 847; CrossRef (e) S. Kotha and S. Vittal, Synlett, 2011, 2329; CrossRef (f) S. Kotha, S. Misra, and S. Venu, Eur. J. Org. Chem., 2012, 4052; CrossRef (g) S. Kotha and G. T. Wagule, J. Org. Chem., 2012, 77, 6314; CrossRef (h) S. G. Modha, A. Kumar, D. D. Vachhani, J. Jacobs, S. K. Sharma, V. S. Parmar, L. V. Meervelt, and E. V. Van der Eycken, Angew. Chem. Int. Ed., 2012, 51, 9572. CrossRef
17. K. Kase, A. Goswami, K. Ohtaki, E. Tanabe, N. Saoko, and S. Okamoto, Org. Lett., 2007, 9, 931. CrossRef
18. (a) S. Kotha and P. Khedkhar, Chem. Rev., 2012, 112, 1650; CrossRef (b) J. L. Segura and N. Martin, Chem. Rev., 1999, 99, 3199; CrossRef (c) M. D. Hoey and D. C. Dittmer, J. Org. Chem., 1991, 56, 1947; CrossRef (d) S. Kotha, T. Ganesh, and A. K. Ghosh, Bioorg. Med. Chem. Lett., 2000, 10, 1755; CrossRef (e) C.-C. Chi, I.-F. Pia, and W.-S. Chung, Tetrahedron, 2004, 60, 10869; CrossRef (f) S. Kotha and A. K. Ghosh, Tetrahedron, 2004, 60, 10833; CrossRef (g) S. Kotha, P. Khedkhar, and A. K. Ghosh, Eur. J. Org. Chem., 2005, 3581; CrossRef (h) S. Kotha and A. S. Chavan, J. Org. Chem., 2010, 75, 4319. CrossRef