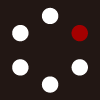
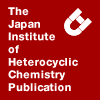
HETEROCYCLES
An International Journal for Reviews and Communications in Heterocyclic ChemistryWeb Edition ISSN: 1881-0942
Published online by The Japan Institute of Heterocyclic Chemistry
e-Journal
Full Text HTML
Received, 25th May, 2013, Accepted, 21st June, 2013, Published online, 1st July, 2013.
DOI: 10.3987/COM-13-S(S)18
■ Expedient Synthesis of 2-Oxopiperazines Using a SN2 / Cope-Type Hydroamination Sequence
Nicolas Das Neves, Michaël Raymond, and André M. Beauchemin*
Department of Chemistry, University of Ottawa, Ottawa Ontario, K1N 6N5, Canada
Abstract
An approach for the synthesis of 2-oxopiperazines from allylic 2-iodoacetamides and aqueous NH2OH has been developed. The reaction proceeds through a SN2 / intramolecular Cope-type hydroamination sequence yielding in 2-oxopiperazines with moderate to good yield.Nitrogen-containing heterocycles are an important class of molecules in natural products, pharmaceuticals, agrochemicals and dyes.1 Among those heterocycles, piperazines are found in a large number of biologically active molecules.2 More specifically, 2-oxopiperazines are important in drug discovery.3,4 They have also been studied as ligands in enantioselective syntheses5 and as conformationally restricted peptidomimetics.6 Their syntheses have evolved in parallel to these developments to achieve their formation with a high degree of control and efficiency.7 However the synthesis of 2-oxopiperazines using a hydroamination strategy has not been reported. In the course of our efforts on the development of Cope-type hydroamination sequences,8,9 we became interested in the synthesis of 2-oxopiperazines using hydroxylamine. The intramolecular version of Cope-type hydroaminations using hydroxylamines has been extensively studied and previous results had showed that the formation of a 6-membered ring is usually difficult:10 the key feature of the reaction would thus require a challenging intramolecular hydroamination step. Herein, we report on a SN2 / intramolecular Cope-type hydroamination reaction sequence for the synthesis of 2-oxopiperazines, using simple and easily synthesized allylic 2-iodoacetamides and thus avoiding the isolation of the polar hydroxylamine intermediate.
The optimization of this sequence was first performed using N,N-diallyl-2-iodoacetamide 1a in t-butanol at 50 °C using aqueous hydroxylamine (1.5 equiv.) and N,N-diisopropylethylamine (1.5 equiv.). A modest but encouraging 29% yield of the expected 2-oxopiperazine 2a was obtained (entry 1). The yield of the reaction could be improved to 68% when the reaction was run with excess NH2OH (3 equiv.) using 2-methyl-2-butanol as solvent and using microwave heating for 2 hours (entry 2). When the same reaction was run using t-butanol as a solvent, the 2-oxopiperazine 2a could be isolated in a 95% yield (entry 3). Changing the base to triethylamine (1.5 equiv.) allowed the isolation of 2-oxopiperazine 2a in a 84% yield (entry 4). Further optimization led us to discover that the use of 3 equivalents of hydroxylamine in combination to excess N,N-diisopropylethylamine (3 equiv.) under similar conditions yielded 2-oxopiperazine 2a in 99% yield (entry 5). In contrast, the parent N,N-diallyl-2-chloroacetamide proved reluctant to undergo the SN2 reaction under similar conditions.
With optimized conditions in hand, we could turn our attention to the exploration of the scope of this reaction sequence (Table 2). The synthesis of various 2-oxopiperazines was thus examined under conditions depicted in Equation 2.
Gratifyingly, the reaction was applicable to several tertiary amides and the 2-oxopiperazines 2a-e were obtained in moderate to good yields (Table 2). These results suggest that tertiary amides adopt a conformation allowing the challenging Cope-type hydroamination to proceed under mild conditions. The structure of the 2-oxopiperazine derivatives was unambiguously established using NMR and mass spectroscopy. More specifically, the 13C NMR of 2a exhibited a characteristic signal at 61.2 ppm corresponding to the CH2 α to the carbonyl group.
Two mechanistic pathways could be envisioned for the formation of 2-oxopiperazines 2 (Scheme 1). First, 2-iodoacetamide 1 could react through a SN2 reaction with hydroxylamine to form intermediate 3 followed by an intramolecular hydroamination to give 2 (pathway A). One could also consider that the mechanism could proceed through a directed intermolecular hydroamination, leading to intermediate 4, followed by an intramolecular SN2 reaction (pathway B). While we felt that pathway A was more likely, our recent results on hydrogen bonding-directed intermolecular Cope-type hydroaminations occurring on relatively similar conditions made us probe this possibility.11 We therefore subjected N,N-diallylacetamide to the reaction conditions and did not see any hydroamination, thus supporting a pathway that would involve an intramolecular hydroamination event (pathway A).
During our studies, we had also noted that 2-iodoacetamide 4a provided the intermediate 5a in a 91% yield (Equation 3), but that this intermediate failed to undergo an intramolecular hydroamination. The latter proved stable and did not react to give 2-oxopiperazine upon heating thus suggesting that conformational effects are very important and restrict the applicability of this reaction sequence. Indeed, as shown below, the s-cis conformation required for cyclization would be disfavored (due to lack of dipole minimization). Analogously, an unfavorable (steric) conformational bias was also observed with N-allyl-N-methylacetamide (4b). For this precursor, we observed less than 10% of the desired product (6b), along with a small amount of the hydroxylamine 5b and decomposition products.
In conclusion, we have shown that a several valuable 2-oxopiperazines can be synthesized in a one pot procedure from 2-iodoacetamides using hydroxylamine. The mechanism of the reaction proceeds through a SN2 reaction to form the hydroxylamine substrate in situ followed by a challenging intramolecular Cope-type hydroamination.
EXPERIMENTAL
All reactions were performed under an inert atmosphere of argon unless otherwise noted. All commercially available reagents (Aldrich and Strem) were used as received without further purification. Purification of reaction products was carried out by flash column chromatography using 40-63 μm silica gel. Analytical thin layer chromatography (TLC) was performed on aluminum sheets pre-coated with silica gel 60 F254. Visualization was accomplished with UV light followed by dipping in a potassium permanganate solution and heating. The deuterated solvents chloroform-d1 or benzene-d6 was used as received. NMR spectra were recorded on a Bruker 300 MHz Avance spectrometer. NMR chemical shifts (δ) were referenced to the residual solvent peaks (1H, 13C). All chemical shifts are reported in parts per million (ppm). HRMS was performed on a Kratos Concept IIH mass spectrometer with an electron beam of 70 ev. Infrared (IR) spectra were obtained as neat thin films on a sodium chloride disk and were recorded on a Bruker EQUINOX 55 Fourier transform infrared spectrometer (FTIR). Microwave heating was performed using a Biotage Initiator 8 microwave reactor.
Starting Materials
Hydroxylamine was used a 50% aqueous solution and was purchased from Sigma-Aldrich and used without any further purification. 2-Iodoacetamides were prepared from the corresponding 2-chloroacetamides (1.0 equiv.) in acetone (0.2 M) with sodium iodide (2.0 equiv.) stirring overnight away from light.
General procedure for the one pot synthesis of 2-oxopiperazines (GP 1): in a flame dried microwave vial was added a solution of alkyl iodide (1.0 equiv.) in tert-butanol (1M). Hydroxylamine (3.0 equiv.) and N,N-diisopropylethylamine (3.0 equiv.) were then successively added. The reaction mixture was then heated at 50 °C for 2 h under microwave irradiation. EtOAc was then added and the organic layer was washed once with brine and once with a saturated aqueous solution of NH4Cl (note: some products were found to be quite water soluble). The organic layer was then dried (Na2SO4), concentrated under reduced pressure and purified by column chromatography.
N,N-Diallyl-2-iodoacetamide (1a)
N,N-Diallyl-2-chloroacetamide (2.50 g, 14.4 mmol, 1.0 equiv), sodium iodide (21.6 g, 144 mmol, 10 equiv) and acetone (144 mL) were mixed together in a round bottom flask at room temperature for 20 h. EtOAc (450 mL) was added and the reaction mixture was washed three times with 100 mL of a (1:1) H2O/brine solution. The organic layer was then dried with sodium sulfate, filtered, concentrated under reduce pressure and purified using flash chromatography (30% EtOAc in hexane). The compound was obtained as a yellow oil (3.64 g, 95% yield). TLC Rf 0.29 (25% EtOAc in hexanes) 1H NMR (CDCl3, 300 MHz) δ 5.88-5.70 (m, 2H), 5.24-5.12 (m, 4H), 3.99-3.94 (m, 2H), 3.93-3.89 (m, 2H), 3.71-3.68 (m, 2H) 13C NMR (CDCl3, 100 MHz) δ 168.1 (C), 132.4 (CH), 132.1 (CH), 117.4 (CH2), 116.9 (CH2), 50.6 (CH2), 48.2 (CH2), -3.6 (CH2) IR (film) 3081, 2983, 2919, 1638, 1450, 1251, 1184, 993, 920 cm-1 HRMS (EI): Exact mass calcd for C8H12INO [M]+ : 264.9964, found : 264.9961.
N-Allyl-N-benzyl-2-iodoacetamide (1b)
N-Allyl-N-benzyl-2-chloroacetamide (1.05 g, 4.41 mmol) was diluted in acetone (20 mL) and sodium iodide (1.32 g, 8.83 mmol) was added. The flask was covered of aluminium foil and the reaction mixture was stirred overnight. The reaction mixture was then filtered over celite and washed with EtOAc. The organic layer was then washed with an aqueous solution of sodium thiosulfate and dried over Na2SO4. Evaporation under vacuum afforded N-allyl-N-benzyl-2-iodoacetamide as yellow oil in 98% yield (1.43 g, 4.34 mmol). Rf 0.75 (4% MeOH in CH2Cl2) 1H NMR (300 MHz, CDCl3) δ 7.20 - 7.51 (m, 5 H) 5.76 - 5.95 (m, 1 H) 5.16 - 5.35 (m, 2 H) 4.56 - 4.73 (m, 2 H) 4.08 (d, J = 5.7 Hz, 1 H) 3.93 (dt, J = 4.8, 1.6 Hz, 1 H) 3.70 - 3.88 (m, 2 H) 13C NMR (75 MHz, CDCl3) δ (mixture of two isomers) 168.5 (C) 168.3 (C*) 136.7 (C) 135.8 (C*) 132.2 (CH) 131.8 (CH*) 128.9 (CH) 128.6 (2 CH*) 127.9 (2 CH) 127.8 (CH*) 127.4 (2 CH*) 126.1 (CH) 117.6 (CH2*) 117.1 (CH2) 51.6 (CH2*) 50.3 (CH2) 48.5 (CH2) 48.3 (CH2*) -3.4 (CH2) -3.3 (CH2*) IR cm-1 (film) 2370, 2231, 1850, 1723, 1561, 1095 HRMS (EI): Exact mass calcd for C12H14N1O1 [M]+ = 315.012, found 315.0107.
N-Allyl-N-(2,2-diethoxyethyl)-2-iodoacetamide (1c)
N-Allyl-2-chloro-N-(2,2-diethoxyethyl)acetamide (1.27 g, 5.08 mmol) was then diluted in acetone (25 mL) and sodium iodide (1.53 g, 10.2 mmol) was added. The flask was covered of aluminium foil and the reaction mixture was stirred overnight. The reaction mixture was then filtered over celite and washed with EtOAc. The organic layer was then washed with an aqueous solution of sodium thiosulfate and dried over Na2SO4. Evaporation under vacuum afforded N-allyl-N-benzyl-2-iodoacetamide as yellow oil in 76% yield (1.32 g, 3.87 mmol). Rf 0.27 (20% EtOAc in Hexane) 1H NMR (300 MHz, C6D6, 353K) δ 5.55 (br. s., 1 H) 4.90 (br. s., 2 H) 2.86 - 4.67 (m, 11 H) 1.05 (br. s., 6 H) 13C NMR (75 MHz, C6D6, 353K) δ 168.2 (C) 134.1 (CH2) 116.8 (CH) 101.8 (CH) 63.7 (2 CH2) 53.6 (CH2) 50.9 (CH2) 15.9 (2 CH3) -2.7 (CH2) IR cm-1 (film) 2975, 2363, 1828, 1735, 1655, 1510, 1091 LRMS m/z (relative intensity): 380.06 (61%) [M+K]+ HRMS (EI): Exact mass calcd for C11H20NO3I [M]+ = 341.0488, found 341.0434.
N-Allyl-N-cyclohexyl-2-iodoacetamide (1d)
N-Allyl-2-chloro-N-cyclohexylacetamide (2.00 g, 9.27 mmol) was then diluted in acetone (50 mL) and sodium iodide (2.80 g, 18.5 mmol) was added. The flask was covered of aluminium foil and the reaction mixture was stirred overnight. The reaction mixture was then filtered over celite and washed with EtOAc. The organic layer was then washed with an aqueous solution of sodium thiosulfate and dried over Na2SO4. Evaporation under vacuum afforded N-allyl-N-cyclohexyl-2-iodoacetamide as yellow oil in 75% yield (2.10 g, 6.84 mmol). Rf 0.63 (4% MeOH in CH2Cl2) 1H NMR (300 MHz, CDCl3, 353K) δ 5.79 (dd, J= 10.9, 5.7 Hz, 1 H) 5.15 (d, J = 17.2 Hz, 2 H) 4.24 (br. s., 1 H) 3.78 - 4.06 (m, 2 H) 3.70 (br. s., 2 H) 1.08 -1.78 (m., 10 H) 13C NMR (75 MHz, CDCl3, 353K) δ 167.7 (C) 135.2 (CH) 115.9 (CH2) 54.8 (CH) 46.8 (CH2) 31.5 (CH2) 30.2 (CH2) 25.8 (2 CH2) 25.4 (CH2) -3.29 (CH2) IR cm-1 (film) 2359, 2339, 1844, 1739, 1574, 1421 HRMS (EI): Exact mass calcd for C11H18N1O1I [M]+ = 307.0433, found 307.0455.
N-Benzyl-2-iodo-N-(2-methylallyl)acetamide (1e)
N-Benzyl-2-chloro-N-(2-methylallyl)acetamide (1.05 g, 4.41 mmol) was then diluted in acetone (20 mL) and sodium iodide (1.32 g, 8.83 mmol) was added. The flask was covered of aluminium foil and the reaction mixture was stirred overnight. The reaction mixture was then filtered over celite and washed with EtOAc. The organic layer was then washed with an aqueous solution of sodium thiosulfate and dried over Na2SO4. Evaporation under vacuum afforded N-benzyl-2-iodo-N-(2-methylallyl)acetamide as yellow oil in 98% yield (1.43 g, 4.34 mmol). Rf 0.45 (20% EtOAc in Hexane) 1H NMR (300 MHz, CDCl3) δ 7.09 - 7.47 (m, 5 H) 4.84 - 4.95 (m, 2 H) 4.61 (s, 1 H) 4.54 (s, 1 H) 4.00 (s, 1 H) 3.77 (s, 2 H) 3. 74 (m, 1 H) 1.73 (s, 3 H) 13C NMR (75 MHz, CDCl3) (mixture of two isomers) δ 168.8 (C) 168.5 (C*) 140.3 (C) 139.5 (C*) 136.8 (C) 135.9 (C*) 129.0 (CH*) 128.6 (CH) 127.9 (CH) 127.8 (CH*) 127.4 (CH) 126.0 (CH*) 112.8 (CH2) 111.4 (CH2) 53.5 (CH2) 51.1 (CH2) 50.8 (CH2) 48.7 (CH2) 20.1 (CH3) -3.4 (CH2) -3.5 (CH2*) IR cm-1 (film) 2363, 2331, 1844, 1735, 1651, 1562, 1095 HRMS (EI): Exact mass calcd for C13H16N1O1 [M]+ = 329.0277, found 329.0338.
4-Hydroxy-5-methyl-1-(prop-2-en-1-yl)piperazin-2-one (2a)
According to general procedure GP 1: N,N-diallyl-2-iodoacetamide (0.265 g, 1.00 mmol), was dissolved in t-BuOH (1 mL), aqueous hydroxylamine (50%) (0.19 mL, 3.00 mmol) and N,N-diisopropylethylamine (0.52 mL, 3.00 mmol) were added. Stirring at 50 °C (under microwave irradiation) for 2 h followed by flash chromatography (5.5% MeOH in CH2Cl2) afforded 4-hydroxy-5-methyl-1-(prop-2-en-1-yl)- piperazin-2-one in 99% yield (0.169 g, 0.990 mmol). TLC Rf 0.34 (8% MeOH in CH2Cl2) 1H NMR (Benzene-d6, 500 MHz, 351 K) δ 5.85-5.34 (m, 2H), 5.04-4.85 (m, 2H), 3.92-3.69 (m, 3H), 3.44-3.33 (m, 1H), 3.15-2.69 (m, 1H), 2.66-2.47 (m, 2H), 0.92 (d, J = 6.0 Hz, 3H) 13C NMR (Benzene-d6, 75 MHz, 351 K) δ 165.9 (C), 133.2 (CH), 117.1 (CH2), 61.2 (CH2), 57.3 (CH), 49.1 (CH2), 48.5 (CH2), 16.0 (CH3) IR (film) 3295, 2977, 2919, 1641, 1497, 1439, 1357, 1278, 1190, 1093, 992, 932, 809, 732, 495 cm-1 HRMS (EI): Exact mass calcd for C8H14N2O2 [M]+ : 170.1055, found : 170.1053.
1-Benzyl-4-hydroxy-5-methylpiperazin-2-one (2b)
According to general procedure GP 1: N-allyl-N-benzyl-2-iodoacetamide (0.315 g, 1.00 mmol) was dissolved in t-BuOH (1 mL), aqueous hydroxylamine (50%) (0.190 mL, 3.00 mmol) and N,N-diisopropylethylamine (0.52 mL, 3.00 mmol) were added. Stirring at 50 °C (under microwave irradiation) for 2 h followed by flash chromatography (4% MeOH in CH2Cl2) afforded 1-benzyl-4-hydroxy-5-methylpiperazin-2-one in 95% yield (0.209 g, 0.95 mmol). Rf 0.31 (4% MeOH in CH2Cl2) 1H NMR (300 MHz, C6D6, 353K) δ 6.96 - 7.29 (m, 5 H), 4.51 - 4.37 (m, 2 H), 3.96 (d, J = 16.6 Hz, 1 H), 3.52 (dd, J = 16.6, 2.6 Hz, 1 H), 2.95 (br. s., 1 H), 2.49 - 2.79 (m, 2 H), 0.92 (dd, J = 5.6, 2.89 Hz, 3 H) 13C NMR (75 MHz, C6D6, 353K) δ 167.2 (C), 137.9 (C), 129.2 (2 CH), 128.8 (2 CH), 127.9 (CH), 61.6 (CH2), 57.7 (CH2), 50.0 (CH2), 49.5 (CH2), 16.4 (CH3) IR cm-1 (film) 3308, 2977, 2932, 1631, 1497, 1448, 1358 LRMS m/z (relative intensity): 220.12 (54%) HRMS (EI): Exact mass calcd for C12H16N2O2 [M]+ = 220.1212, found 220.1188.
1-(2,2-Diethoxyethyl)-4-hydroxy-5-methylpiperazin-2-one (2c)
According to general procedure GP 1: N-allyl-N-benzyl-2-iodoacetamide (0.170 g, 0.500 mmol) was dissolved in t-BuOH (0.5 mL), aqueous hydroxylamine (50%) (0.095 mL, 1.500 mmol) and N,N-diisopropylethylamine (0.26 mL, 1.50 mmol) were added. Stirring at 50 °C (under microwave irradiation) for 1 h followed by flash chromatography (3% MeOH in CH2Cl2) afforded 1-(2,2-diethoxyethyl)-4-hydroxy-5-methylpiperazin-2-one in 56% yield (0.069 g, 0.280 mmol). Rf 0.42 (4% MeOH in CH2Cl2) 1H NMR (300 MHz, C6D6, 353K) δ 4.67 - 4.79 (m, 1 H) 3.90 (d, J = 16.6 Hz, 1 H) 3.33 - 3.70 (m, 7 H) 3.24 (br. s., 1 H) 3.03 (d, J = 11.2 Hz, 1 H) 2.64 - 2.86 (m, 1 H) 0.95 - 1.16 (m, 9 H) 13C NMR (75 MHz, C6D6, 353K) δ 167.2 (C) 101.9 (CH) 63.5 (2 CH2) 61.6 (CH2) 58.0 (CH) 52.3 (CH2) 50.6 (CH2) 16.28 (CH3) 15.87 (CH3) IR cm-1 (film) 3341, 2979 2339, 1824, 1731, 1534, 1417 LRMS m/z (relative intensity): 247.21 (29%) [M+H]+ HRMS (EI): Exact mass calcd for C11H22N2O4 [M-C2H6O]+ = 200.1161, found 200.1160.
1-Cyclohexyl-4-hydroxy-5-methylpiperazin-2-one (2d)
According to general procedure GP 1: N-allyl-N-cyclohexyl-2-iodoacetamide (0.307 g, 1.00 mmol) was dissolved in t-BuOH (1 mL), aqueous hydroxylamine (50%) (0.190 mL, 3.00 mmol) and N,N-diisopropylethylamine (0.52 mL, 3.00 mmol) were added. Stirring at 50 °C (under microwave irradiation) for 2 h followed by flash chromatography (4% MeOH in CH2Cl2) afforded 1-cyclohexyl-4-hydroxy-5-methylpiperazin-2-one in 62% yield (0.131 g, 0.620 mmol). Rf 0.21 (4% MeOH in CH2Cl2) 1H NMR (300 MHz, C6D6, 353K) δ 4.44 (br. s., 1 H) 3.96 (d, J = 16.5 Hz, 1 H) 3.53 (d, J = 16.6 Hz, 1 H) 2.62 - 3.02 (m, 3 H) 1.58 - 0.87 (m, 14 H) 13C NMR (75 MHz, C6D6, 353K) δ 166.4 (C) 61.9 (CH2) 58.1 (CH) 53.0 (CH) 45.1 (CH2) 30.2 (CH2) 30.1 (CH2) 26.5 (CH2) 26.4 (CH2) 26.3 (CH2) 16.5 (CH3) IR cm-1 (film) 2931, 2854, 2863, 2335, 1844, 1735, 1699, 1454, 1087 HRMS (EI): Exact mass calcd for C11H20N2O2 [M]+ = 212.1525, found 212.1541.
1-Benzyl-4-hydroxy-5,5-dimethylpiperazin-2-one (2e)
N-Benzyl-2-iodo-N-(2-methylallyl)acetamide (0.165 g, 0.500 mmol) was dissolved in t-BuOH (0.5 mL). Aqueous hydroxylamine (50%) (0.063 mL, 2.00 mmol) and triethylamine (0.140 mL, 2.00 mmol) were then successively added. After stirring at 50 °C (under microwave irradiation) for 1 h followed by flash chromatography (100% CH2Cl2 to 96/4 CH2Cl2/MeOH) afforded 1-benzyl-4-hydroxy- 5,5-dimethylpiperazin-2-one in 75% yield (0.088 g, 0.370 mmol). Rf 0.33 (4% MeOH in DCM) 1H NMR (300 MHz, C6D6, 353K) δ 6.93 - 7.24 (m, 5 H) 4.44 (s, 2 H) 3.77 (s, 2 H) 2.84 (s, 2 H) 0.91 (s, 6 H) 13C NMR (75 MHz, C6D6, 353K) δ 167.2 (C) 138.0 (C) 129.1 (2 CH) 128.9 (2 CH) 127.9 (CH) 57.9 (CH2) 57.2 (C) 53.9 (CH2) 50.2 (CH2) 22.2 (2 CH3) IR cm-1 (film) 3295, 2371, 1848, 1651, 1534, 1454, 1240, 1091 HRMS (EI): Exact mass calcd for C13H18N2O2 [M]+ = 234.1368, found 234.1383.
N-Allyl-N-tosyl-2-iodoacetamide (4a)
N-Allyl-2-chloro-N-tosylacetamide (1.37 g, 4.83 mmol) was then diluted in acetone (25 mL) and sodium iodide (1.53 g, 10.2 mmol) was added. The flask was covered of aluminium foil and the reaction mixture was stirred overnight. The reaction mixture was then filtered over celite and washed with EtOAc. The organic layer was then washed with an aqueous solution of sodium thiosulfate and dried over Na2SO4. Evaporation under vacuum afforded N-allyl-N-tosyl-2-iodoacetamide as yellow oil in quantitative yield (1.83 g, 4.83 mmol). Rf 0.34 (20% EtOAc in Hexane) 1H NMR (300 MHz, CDCl3) δ 7.84 (m, J = 8.4 Hz, 2 H) 7.35 (m, J = 7.9 Hz, 2 H) 5.86 (ddt, J = 17.2, 10.4, 5.3 Hz, 1 H) 5.16 - 5.35 (m, 2 H) 4.46 (dt, J = 5.3, 1.4 Hz, 2 H) 4.06 (s, 2 H) 2.44 (s, 3 H) 13C NMR (75 MHz, CDCl3) δ 167.1 (C) 145.2 (C) 135.3 (C) 132.0 (CH) 129.7 (2 CH) 128.1 (2 CH) 118.3 (CH2) 49.3 (CH2) 21.6 (CH3) -1.6 (CH2) IR cm-1 (film) 2359, 1840, 1707, 1635, 1433, 1361 HRMS (EI): Exact mass calcd for C12H14N1O3IS [M]+ = 378.9739, found 378.9711.
N-Allyl-2-(hydroxyamino)-N-tosylacetamide (5a)
According to general procedure GP 1: N-allyl-N-tosyl-2-iodoacetamide (0.379 g, 1.00 mmol) was dissolved in t-BuOH (1 mL), aqueous hydroxylamine (50%) (0.190 mL, 3.00 mmol) and N,N-diisopropylethylamine (0.52 mL, 3.00 mmol) were added. Stirring at 50 °C (under microwave irradiation) for 2 h followed by flash chromatography (4% MeOH in CH2Cl2) afforded N-allyl-2-(hydroxyamino)-N-tosylacetamide in 91% yield (0.260 g, 0.910 mmol). Rf 0.73 (4% MeOH in DCM) 1H NMR (300 MHz, CDCl3) δ 7.76 (d, J = 8.3 Hz, 2 H) 7.30 (s, 2 H) 5.56 - 5.80 (m, 1 H) 5.33 (t, J = 6.1 Hz, 1 H) 4.97 - 5.25 (m, 2 H) 3.54 (t, J = 6.0 Hz, 2 H) 2.40 (s, 3 H) 13C NMR (75 MHz, CDCl3) δ 143.2 (2 C) 136.7 (C) 132.8 (CH) 129.5 (2 CH) 126.9 (2 CH) 117.2 (CH2) 45.5 (2 CH2) 21.3 (CH3) IR cm-1 (film) 3438, 2098, 1643, 1152 HRMS (EI): Exact mass calcd for C12H16N2O4S = 284.0831. Not found. Exact mass calcd for C7H7O2S [M-C5H9N2O2]+ = 155.0167 found 155.0182, exact mass calcd for C5H9N2O2 [M-C7H7O2S]+ = 129.0664, found 129.0545.
ACKNOWLEDGEMENTS
We thank the University of Ottawa, NSERC (Discovery Grant, Discovery Accelerator Supplement, CRD and CREATE grants to A.M.B.), the Canadian Foundation for Innovation, and the Ontario Ministry of Research and Innovation for generous financial support. Indirect support of this work by AstraZeneca Canada, and OmegaChem is also gratefully acknowledged. We also thank Francis Loiseau for helpful discussions.
References
1. J. A. Joule and K. Mills, Heterocyclic Chemistry, 4th ed., Blackwell, Oxford, 2000; T. Eicher and S. Hauptmann, The Chemistry of Heterocycles, Wiley-VCH, Weinheim, 2003; CrossRef A. R. Katrizky and A. F. Pozharskii, Handbook of Heterocyclic Chemistry, 2nd Ed, Pergamon, Amsterdam, 2000.
2. S. E. Ward, F. P. Harrington, L. J. Gordon, S. C. Hopley, C. M. Scott, and J. M. Watson, J. Med. Chem., 2005, 48, 6478; M. Leopoldo, E. Lacivita, N. A. Calobufo, M. Contino, F. Berardi, and R. Perrone, J. Med. Chem., 2005, 48, 7919; CrossRef D. Askin, K. K. Eng, K. Rossen, R. M. Purick, K. M. Welss, R. P. Volante, and P. J. Reider, Tetrahedron Lett., 1994, 35, 673. CrossRef
3. B. M. Cochran and F. E. Michael, Org. Lett., 2008, 10, 329; CrossRef J. S. Nakhla and J. P. Wolfe, Org. Lett., 2007, 9, 3279; CrossRef J. Seigel, D. Brown, A. Amour, S. J. Macdonald, N. J. Oldham, and C. J. Schofield, Bioorg. Med. Chem. Lett., 2003, 13, 387; CrossRef C. J. Dinsmore, J. M. Bergman, D. D. Wei, C. Blair Zartman, J. P. Davide, I. B. Greenberg, D. Liu, T. J. O’Neill, J. B. Gibbs, K. S. Koblan, N. E; Kohl, R. B. Lobell, I-W. Chen, D. A. McLoughlin, T. V. Olah, S. L. Graham, G. D. Hartman, and T. M. Williams, Bioorg. Med. Chem. Lett., 2001, 11, 537; CrossRef J. M. Bergman, M. T. Abrams, J. P. Davide, I. B. Greenberg, R. G. Robinson, C. A. Buser, H. E. Huber, K. S. Koblan, N. E. Kohl, R. B. Lobell, S. L. Graham, G. D. Hartman, T. M. Williams, and C. J. Dinsmore, Bioorg. Med. Chem. Lett., 2001, 11, 1411; CrossRef S. Kitamura, H. Fukushi, T. Miyawaki, M. Kawamura, N. Konishi, Z.-i. Terashita, and T. Naka, J. Med. Chem., 2001, 44, 2438; CrossRef D. A. Horton, G. T. Bourne, and M. L. Smythe, Chem. Rev., 2003, 103, 893; CrossRef M. Tullberg, M. Grøtli, and K. Luthman, J. Org. Chem., 2003, 103, 893; N. Meddad-Belhabich, D. Aoun, A. Djimé, C. Redeuilh, G. Dive, F. Massicot, F. Chau, F. Heymans, and A. Lamouri, Bioorg. Med. Chem., 2010, 18, 3588; CrossRef T. E. Fischer, B. Kim, D. D. Staas, T. A. Lyle, S. D. Young, J. P. Vacca, M. M. Zrada, D. J. Hazuda, P. J. Felock, W. A. Schleif, L. J. Gabryelski, M. R. Anari, C. J. Kochansky, and J. S. Wai, Bioorg. Med. Chem., 2007, 17, 6511. CrossRef
4. K. M. Gurjar, S. Karmakar, D. K. Mohapatra, and U. D. Phalgune, Tetrahedron Lett., 2002, 43, 1897; CrossRef T. Shioiri and N. Irako, Chem. Lett., 2002, 130; CrossRef F. A. Davis and J. Deng, Org. Lett., 2005, 7, 621; CrossRef B. M. Trost and G. Dong, J. Am. Chem. Soc., 2009, 128, 6054; CrossRef S. P. Govek and L. E. Overman, J. Am. Chem. Soc., 2001, 123, 9468; CrossRef D. E. N. Jacquot, M. Zöllinger, and T. Lindel, Angew. Chem. Int. Ed., 2005, 44, 2295. CrossRef
5. J. Eriksson, P. I. Arvidsson, and O. Davidsson, Chem. Eur. J., 1999, 5, 2356; CrossRef D. Nakamura, K. Kakiuchi, K. Koga, and R. Shirai, Org. Lett., 2006, 8, 6139; CrossRef S. Itsuno, T. Matsumoto, D. Sato, and T. Inoue, J. Org. Chem., 2000, 65, 5879; CrossRef Z. Wang, M. Cheng, P. Wu, S. Wei, and J. Sun, Org. Lett., 2006, 8, 3045. CrossRef
6. J. A. Gonzalez-Vera, M. T. Garcia-Lopez, and R. Herranz, J. Org. Chem., 2005, 70, 3660; CrossRef F. Rübsam, R. Mazitschek, and A. Giannis, Tetrahedron, 2000, 56, 8481; CrossRef A. Nefzi, M. A. Giulianotti, and R. A. Houghten, Tetrahedron, 2000, 56, 3319; CrossRef Y. Tong, Y. M. Fobian, M. Wu, N. D. Boyd, and K. D. Moeller, Bioorg. Med. Chem. Lett., 1998, 8, 1679; CrossRef J. DiMaio and B. Belleau, J. Chem. Soc., Perkin Trans. 1, 1989, 1687; CrossRef Y. Tong, Y. M. Fobian, M. Wu, N. D. Boyd, and K. D. Moeller, J. Org. Chem., 2000, 65, 284; CrossRef E. O’Reilly, L. Pes, and F. Paradisi, J. Org. Chem., 2010, 51, 1696; C. J. Dinsmore, J. M. Bergman, M. J. Bogusky, J. C. Culberson, K. A. Hamilton, and S. L. Graham, Org. Lett., 2001, 3, 865; CrossRef H. Peng, D. Carrico, V. Thai, M. Blakovich, C. Bucher, E. E. Pusateri, S. M. Sebti, and A. D. Hamilton, Org. Biomol. Chem., 2006, 4, 1768; CrossRef C. J. Dinsmore, C. B. Zartman, J. M. Bergman, M. T. Abrams, C. A. Buser, J. C. Culberson, J. P. Davide, M. Ellis-Huychings, C. Fernandes, S. L. Graham, G. D. Hartman, H. E. Huber, R. B. Lobell, S. D. Mosser, R. G. Robinson, and T. M. Williams, Bioorg. Med. Chem. Lett., 2004, 14, 639; CrossRef J. Seibel, D. Brown, A. Amour, S. J. Macdonald, N. J. Oldham, and C. J. Schofield, Bioorg. Med. Chem. Lett., 2003, 13, 387; CrossRef T. Su, H. Yang, D. Volkots, J. Woolfrey, S. Dam, P. Wong, U. Sinha, R. M. Scarborough, and B.-Y. Zhu, Bioorg. Med. Chem. Lett., 2003, 13, 729; CrossRef D. D. Holsworth, N. A. Powell, D. M. Downing, C. Cai, W. L. Cody, J. M. Ryan, R. Ostroski, M. Jalaie, J. W. Bryant, and J. J. Edmunds, Bioorg. Med. Chem. Lett., 2005, 13, 2657. CrossRef
7. For a review see: C. De Risi, M. Pelà, G. P. Pollini, C. Trapella, and V. Zanirato, Tetrahedron: Assymmetry, 2010, 21, 255; CrossRef C. J. Dinsmore and D. C. Beshore, Tetrahedron, 2002, 58, 3297; CrossRef C. L. Lencina, A. Dassonville-Klimpt, and P. Sonnet, Tetrahedron: Asymmetry, 2008, 19, 1689; CrossRef Z. Chen and A. S. Kende, Synth. Commun., 2006, 36, 473; CrossRef A. Viso, R. F. Pradilla, A. Flores, A. Garcia, M. Tortosa, and M. L. Lopez-Rodrigues, J. Org. Chem., 2006, 71, 1442; CrossRef A. Pohlmann, V. Schanen, D. Guillaume, J. C. Quirion, and H. P. Husson, J. Org. Chem., 1997, 62, 1016; CrossRef V. Schanen, C. Riche, A. Chiaroni, J. C. Quirion, and H. P. Husson, Tetrahedron Lett., 1994, 35, 2533. CrossRef
8. J. Bourgeois, I. Dion, P. H. Cebrowski, F. Loiseau, A.-C. Bédard, and A. M. Beauchemin, J. Am. Chem. Soc., 2009, 131, 874; CrossRef T. Rizk, E. J.-F. Bilodeau, and A. M. Beauchemin, Angew. Chem. Int. Ed., 2009, 48, 8325; CrossRef For an entry in the Cope-type hydroamination literature, see: J. Moran, S. I. Gorelsky, E. Dimitrijevic, M.-E. Lebrun, A.-C. Bédard, C. Seguin, and A. M. Beauchemin, J. Am. Chem. Soc., 2008, 130, 17893 and reference 10. CrossRef
9. For examples of hydroamination sequences, see: Y.-P. He, D.-F. Chen, J. Yu, and L.-Z. Gong, Chem. Eur. J., 2013, 19, 5232; CrossRef A. Duris, D. M. Barber, H. J. Sanganee, and D. J. Dixon, Chem. Commun., 2013, 49, 2777; CrossRef D. M. Barber, H. J. Sanganee, and D. J. Dixon, Org. Lett., 2012, 14, 5290; CrossRef A. Pews-Davtyan and M. Beller, Chem. Commun., 2011, 47, 2152; CrossRef A. Heutling, F. Pohlki, I. Bytschkov, and S. Doye, Angew. Chem. Int. Ed., 2005, 44, 2951; CrossRef L. Ackermann, L. T. Kaspar and C. J. Gschrei, Chem. Commun., 2004, 24, 2824; CrossRef P. Subramanian and K. P. Kaliappan, Eur. J. Org. Chem., 2013, 595; CrossRef W. C. Liu and Z. Q. Cui, Org. Biomol. Chem., 2011, 19, 6484; CrossRef V. Baxter, M. Jenny, and L. James, Org. Lett., 2011, 15, 4056; CrossRef H. Cao, T. O. Vieira, and H. Alper, Org. Lett., 2011, 13, 11; CrossRef R. Severin, J. Reimer, and S. Sven, Eur. J. Org. Chem., 2010, 51; CrossRef Y.-P. He, H. Wu, D.-F. Chen, J. Yu, and L.-Z. Gong, Chem. Eur. J., 2013, 19, 5232; CrossRef Y.-Q. Zhang, D.-Y. Zhu, B.-S. Li, Y.-Q. Tu, J.-X. Liu, Y. Lu, and S.-H. Wang, J. Org. Chem., 2012, 77, 4167; CrossRef H.-F. Wang, T. Yang, P.-F. Xu, and D. J. Dixon, Chem. Commun., 2009, 26, 3916; CrossRef R. Lebeuf, F. Robert, K. Schenk, and Y. Landais, Org. Lett., 2006, 21, 4755; CrossRef For a review, see: X. Zeng, Chem. Rev., 2013, DOI: 10.1021/cr400082n and references therein. CrossRef
10. N. J. Cooper and D. W. Knight, Tetrahedron, 2004, 60, 243; CrossRef E. H. Krenske, E. C. Davison, I. T. Forbes, J. A. Warner, A. L. Smith, A. B. Holmes, and K. N. Houk, J. Am. Chem. Soc., 2012, 134, 2434. CrossRef
11. S. Zhao, E. Bilodeau, V. Lemieux, and A. M. Beauchemin, Org. Lett., 2012, 14, 5082. CrossRef