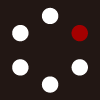
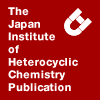
HETEROCYCLES
An International Journal for Reviews and Communications in Heterocyclic ChemistryWeb Edition ISSN: 1881-0942
Published online by The Japan Institute of Heterocyclic Chemistry
e-Journal
Full Text HTML
Received, 2nd July, 2013, Accepted, 25th July, 2013, Published online, 5th August, 2013.
DOI: 10.3987/COM-13-12766
■ NOVEL NITENPYRAM ANALOGUES WITH TETRAHYDROPYRIDONE-FIXED CIS-CONFIGURATION: SYNTHESIS, INSECTICIDAL ACTIVITIES, AND MOLECULAR DOCKING STUDIES
Chuan-Wen Sun,* Jing Wang, Ying Wu, Shi-Bin Nan, and Wang-Geng Zhang
College of Life and Environmental Science, Shanghai Normal University , No. 100 Guilin road, Shanghai 200234, China
Abstract
To make further researches on the diversity of nitenpyram analogues with a cis-nitromethylene configuration, a series of cis-nitenpyram analogues (3a-q) with tetrahydropyridone fixed cis-configuration were designed and synthesized. Preliminary bioassays showed that most of the designed nitenpyram analogues exhibited good insecticidal activity at 100 mg/L against Nilaparvata lugens and Myzus persicae, while analogues 3n afforded the best in vitro activity. Modeling the ligand-receptor complexes by molecular docking study revealed the analogues 3 with various substituents on phenyl show their different binding affinities to the insect nAChR, which also explained the structure-activity relationships observed in vitro.INTRODUCTION
Nowadays, neonicotinoids insecticides(NNSs) are the most important chemical class of insecticides introduced to the global market since the synthetic pyrethroids.1 Interacting with the insect nicotinic acetylcholine receptors (nAChRs),2-4 NNSs have a higher affinity for the insect receptor than for the mammalian and are relatively safe toward mammals and aquatic life.5-8 Although NNSs are fruitful in modern agricultural pest management and environmental protection, it is still essential to explore novel neonicotinoid candidates, because insecticide resistance and cross-resistance have been observed in a range of species after frequent field applications.9-16
According to the chemical structures of neonicotinoids developed in the past several decades, the nitro group play an important role in the activities of neonicotinoids and nitromethylene segments are in trans-configuration in commercialized neonicotinoids.17 In the recent years, a number of neonicotinoid analogues with cis-configuration have been reported and shown high insecticidal activities and gained valuable results in resistance management.17-19 But most of these cis-configuration neonicotinoid analogues are designed on cyclic neonicotinoid insecticides, such as imidacloprid, few studies have been focused on the structural modification of acyclic NNSs, such as nitenpyram and acctamiprid. Encouraged by this, we have designed and synthesized cis-nitenpyram analogues 2 by using a tetrahydropyrimidine ring to fix the the direction of nitro group of nitenpyram (Figure 1) in previous studies.20-23 Preliminary bioassays indicated that most of nitenpyram analogues 2 showed good insecticidal activities against Nilaparvata lugens. However, we found that analogues 2 have similar skeleton— tetrahydropyrimidine ring, this is not satisfactory in the research of the diversity of nitenpyram analogues. Therefore, to find the diverse skeleton of nitenpyram analogues with a cis nitromethylene configuration, our interest is to design novel nitenpyram analogues by introducing a new heterocycle ring into the nitenpyram.
The applications of Meldrum’s acid to the synthesis of heterocyclic compounds in recent years are reviewed. All these heterocyclic compounds are related to pyranoid, pyridine, furan rings, tetrahydropyridone rings and so on.24-28 Motivated by this, we using nitenpyram 1, aromatic aldehyde and Meldrum's acid as starting materials through a rapid microwave (MW)-promoted method (Scheme 1) to obtain new cis-nitenpyram analogues 3 by introducing a tetrahydropyridone ring into the nitenpyram (Figure 1). The preliminary insecticidal activities of the target compounds were tested, most of the designed nitenpyram analogues(3a-3q) showed good insecticide activities against Myzus persicae, Aphis medicaginis and Nilaparvata lugens. To further investigate their binding interactions, molecular docking simulations were carried out by docking the nitenpyram analogues 3 into the active site of nAChR.
RESULTS AND DISCUSSION
1. Chemistry
The nitenpyram analogues bearing a tetrahydropyridone ring (3a-q) were synthesized as shown in Scheme 1. Nitenpyram 1 and meldrum's acid 4 were prepared as described in the literatures,29-30 respectively. Three-component microwave-assisted reaction of nitenpyram, aromatic aldehyde and Meldrum's acid in ethanol gave good yields (59-89%).
Possible mechanism for the formation of the nitenpyram analogues is described in Scheme 2. The assembly of 7 can be explained via the initial Michael addition of enamine double bond in 1 to 6, leading to the formation of an acyclic intermediate 8, which cyclized into the intermediate 9 via nucleophilic attack of an NH group on a carbonyl carbon, then one molecule of acetone and carbon dioxide are split off and finally the tetrahydropyridine ring can be closed.27 It is an efficient and promising method to construct the tetrahydropyridone skeleton.
2. Crystal Structure Analysis
The structure of compounds 3g was unambiguously confirmed by X-ray crystallographic diffraction analysis. As compared with the trans configuration of nitromethylene in the crystal structure of nitenpyram,31 3g is obviously in the cis configuration as anticipated. From Figure 2, the tetrahydropyridone ring adopts a skew boat conformation and a N2/C9/C10/N4 dihedral angle of -20.2 (5)°. In the tetrahydropyridone moiety, C(13)–C(12), C(11)–C(10) and C(12)–C(11) bond lengths (1.484(4) Å, 1.501(4) Å and 1.533(4) Å) are slightly shorter than normal (1.54 Å). The C(13)–N(3), C(9)–N(3), and C(10)–N(4) bond lengths (1.390 Å(4), 1.405Å(3), and 1.412 Å(4), respectively) are slightly shorter than normal (1.47 Å), while the C(9)=C(10) bond length (1.371(4) Å) is slightly longer than normal (1.34 Å). Besides, the dihedral angle between the mean planes of the benzene and pryidine rings is 80.7 (3)°.
3. Structure activity relationship
The insecticidal activities against Nilaparvata lugens, Myzus persicae and Aphis medicaginis of the title compounds were investigated using nitenpyram as a control, and the results are presented in Table 1. Most of our designed analogues showed better insecticide activities against Myzus persicae, Nilaparvata lugens and Aphis medicaginis (Table 1). Among these analogues, 3n afforded the best in vitro inhibitory activities and had 100% mortality at 20 mg/L against Myzus persicae and Nilaparvata lugens, the LC50 values of 3n against Myzus persicae and Nilaparvata lugens are 0.191 mg/L and 0.219 mg/L, respectively, that is very close to nitenpyram. In general, the analogues with different substituents on benzene ring exhibited equivalent activities at high dose (500 mg/L). However, the insecticidal activities showed significant differences when the doses were reduced to 100 and 20 mg/L.
As depicted in Table 1, against Nilaparvata lugens, compound 3a with no substitution on the phenyl group presented moderate insecticidal activity at 100 and 20 mg/L. Introducing electron-donating substituents on benzene ring were unfavorable to activities, most of these compounds (3b-e) exhibited unpleasing insecticidal effectiveness. For the effect of electron-withdrawing groups, introducing halogen atoms such as single fluro, chloro atom on benzene ring did not contribute to activity improvement of the corresponding compounds, insecticidal activities of compounds (3f-k) were little more than those with electron-donating substituents on benzene ring (3b-e). Interestingly, bring bromo atom in the benzene ring (3l) resulted in greatly increased insecticidal activity, whereas double chloro atoms on benzene ring (3p, 3q) also significantly increased activities. Among electron-withdrawing groups on benzene ring, trifluoromethyl (3n) was most prominent group in increasing activity. For Myzus persicae and Aphis medicaginis, all these cis-nitenpyram analogues have the similar structure activity relationship. Considering the discussion above, we found that insecticidal potencies of our designed nitenpyam analogues could be increased by the introduction of bromo, trifluoromethyl and dichloride substituents on benzene rings.
4. Molecular docking study
To further explore the structural features for better activities, models of these new compounds-receptor complexes were investigated by docking studies with CDOCKER.20-22 Since the amino acids forming the active sites are both structurally and functionally consistent in the diverse nAChRs and AchBPs, the published crystal structure of a Lymnaea stagnalis-AChBP (Ls-AChBP) co-crystallized with imidacloprid (PDB ID: 2zju)32 was used as the template of receptor. The docking study was performed by using the program CDOCK (Discovery Studio 3.1).
As a result, all active analogues exhibited significant hydrogen-bonding interactions with the nAChR target. As illustrated in Figure (3a), chloropyridine N of 3n exhibits a hydrogen bond with NH of Trp143 backbone on loop B, while the nitro O(32) interact with OH of Tyr192 on loop C, which suggest that chloropyridine N and nitro O play a key role in recognizing and interacting with the binding site. In addition, its binding conformation exhibits another two important hydrogen bonds: O(24) of its carbonyl interacts with SH of Cys187, and F(27) interacts with the side chain NH of Gln55. These observations have also explained why the analogue 3n attained the highest score.
Furthermore, most of the other active analogues shared a quite similar binding mode with analogue 3n and many of them exhibited more than two hydrogen-bonds with different amino acids of the active pocket between the nAChR subunits. However, not all carbonyl O(24) of analogues 3 can form hydrogen bond within the same hydrogen bond distance, such as the binding mode of analogue 3g (Figure 3(b)), O(24) of its carbonyl cannot interact with amino acid residues from the Ls-AChBP.
Moreover, we found that analogues offered high experimental activities when carbonyl can exhibit hydrogen-bond with different amino acids residues (Figure 3(a, c, d)). These observations have also explained the structure-activity relationships observed in vitro. Thereby, the newly introduced substituents of the designed analogues presumably played important roles in ligand recognition and binding interactions, which may further enhance their activities and contribute to the selectivity as well. Based on these, further target inhibitory tests and advanced insecticide design are underway.
CONCLUSION
In conclusion, a series of cis-nitenpyram analogues (3a-q) with tetrahydropyridone fixed cis-configuration were designed and synthesized. Preliminary bioassays showed that most of the designed nitenpyram analogues exhibited good insecticidal activity at 100 mg/L while the analogues 3n afforded the best in vitro activity. The docking results revealed that analogues 3 with various substituents on phenyl show their different binding affinities to the insect nAChR, which explained the SARs observed in vitro. Moreover, carbonyl O(24) of some analogues can exhibite hydrogen-bond with amino acid residues of receptor, which play a crucial role for their high insecticidal activities. The study herein has shed a light on the mechanism of action of these cis-nitenpyram analogues (3a-q) containing tetrahydropyridone, further researches on much more test objects and structural modification of nitenpyram are underway.
EXPERIMENTAL
Melting points were measured using an uncorrected RK-1 microscopic melting-point apparatus. 1H and 13C NMR spectra were obtained on a Bruker Avance-400 at 400 and 100 MHz, respectively. 1HNMR and 13CNMR spectra were record with DMSO-d6 or CDCl3 as an internal standard, coupling constants (J) were recorded in Hz. The IR spectra were obtained from KBr discs in the range 4000-400cm-1 on a Nicolet 5DXFT-IR spectrophotometer. Mass spectra were in general recorded on an AMD 402/3 or a HP 5989A mass selective detector. Combustion analyses for elemental composition were made with a Perkin-Elmer 2400 instrument. All microwave experiments were performed using YL8023B1 microwave reactor possessing a single-mode microwave cavity producing controlled irradiation at 2.45GHz. Unless otherwise noted, reagents and solvents were of analytical reagent grade or were chemically pure and used as received without further purification.
General Synthetic Procedures for Compounds 3a-3q
A solution of aromatic aldehyde (15 mmol), Meldrum's acid (15 mmol) and Nitenpyram 1 (10 mmol) in EtOH (30 mL), piperidine (0.1 mmol) used as catalyst was added dropwise. The mixture solution theated to 65 °C for 30 min in a microwave reactor and cooled to room temperature. The reaction mixture was concentrated under reduced pressure and treated with 20 mL of water. Then, the solution was extracted three times with CH2Cl2, and the combined extracts were dried over MgSO4. The organic phase was evaporated under reduced pressure and crude product was subjected to flash chromatography on silica gel, eluting with EtOAc /petroleum ether (1:1) to afford pure products.
6-[N-(6-Chloro-3-pyridinylmethyl)-N-ethyl]amino-1-methyl-4-phenyl-5-nitro-1,2,3,4-tetrahydropyridin-2-one (3a): yield 67%; a yellow solid; mp 163-164 °C; IR (KBr, cm-1) 2981, 2934, 1703, 1584, 1273, 1207, 1099; 1H NMR (400 MHz, CDCl3) δ 8.23 (s, 1H, Py-H), 7.40 (s, 1H, Py-H), 7.19-7.28 (m, 4H, Py-H and benzene-H), 6.99 (s, 2H, benzene-H), 4.68 (t, J = 3.8 Hz, 1H, benzene-CH), 4.39 (d, J = 14.7 Hz, 1H, Py-CH2), 4.18 (d, J = 14.7 Hz, 1H, Py-CH2), 3.13-3.15 (m, 2H), 3.09 (d, J = 4.0 Hz, 2H), 3.04 (s, 3H, N-CH3), 1.29 (t, J = 6.9 Hz, 3H, NCH2CH3); 13C NMR (100 MHz, CDCl3) δ 170.3, 151.7, 149.8, 146.2, 140.3, 139.2, 131.4, 128.4, 127.9, 125.7, 123.5, 98.8, 53.5, 40.8, 35.9, 29.4, 27.1, 13.8; MS (ESI) m/z: 401 ([M+H]+). Anal. Calcd for C20H21ClN4O3; C, 59.92; H, 5.28; N, 13.98. Found: C, 59.90; H, 5.25; N, 13.95.
6-[N-(6-Chloro-3-pyridinylmethyl)-N-ethyl]amino-1-methyl-4-(4-methylphenyl)-5-nitro-1,2,3,4-tetrahydropyridin-2-one (3b): yield 62%; a yellow solid; mp 192-193 °C; IR (KBr, cm-1) 2993, 2970, 1706, 1572, 1438, 1276, 1098; 1H NMR (400 MHz, CDCl3) δ 8.15 (s, 1H, Py-H), 7.32 (s, 1H, Py-H), 7.13 (s, 1H, Py-H), 6.79-6.99 (m, 4H, benzene-H), 4.56 (s, 1H, benzene-CH), 4.32 (d, J = 14.6 Hz, 1H), 4.11 (d, J = 14.6 Hz, 1H, Py-CH2), 3.06-3.15 (m, 2H), 2.97-3.00 (m, 5H), 2.24 (s, 3H), 1.22 (t, J = 6.6 Hz, 3H, NCH2CH3); 13C NMR (100 MHz, CDCl3) δ 173.2, 155.2, 149.2, 144.3, 139.2, 135.1, 133.4, 130.2, 129.1, 127.8, 125.3, 96.8, 52.7, 40.1, 36.1, 29.1, 27.0, 20.9, 12.9; MS (ESI) m/z: 415 ([M+H]+). Anal. Calcd for C21H23ClN4O3; C, 60.79; H, 5.59; N, 13.50. Found: C, 60.78; H, 5.60; N, 13.57.
6-[N-(6-Chloro-3-pyridinylmethyl)-N-ethyl]amino-1-methyl-4-(4-isopropylphenyl)-5-nitro-1,2,3,4-tetrahydropyridin-2-one (3c): yield 72%; a yellow solid; mp 164-165 °C; IR (KBr, cm-1) 2992, 2980, 1704, 1578, 1431, 1273, 1090; 1H NMR (400 MHz, CDCl3) δ 8.20 (s, 1H, Py-H), 7.35 (s, 1H, Py-H), 7.12-7.15 (m, 1H, Py-H), 7.02-7.04 (m, 2H, benzene-H), 6.79 (s, 2H, benzene-H), 4.56 (t, J = 3.5 Hz, 1H, benzene-CH), 4.33 (d, J = 14.6 Hz, 1H, Py-CH), 4.13 (d, J = 14.6 Hz, 1H, Py-CH), 3.06-3.08 (m, 2H), 2.99 (s, 2H), 2.98 (s, 3H), 2.76-2.83 (m, 1H, CH(CH3)2), 1.23 (t, J = 7.0 Hz, 3H, NCH2CH3), 1.16 (d, J = 6.9 Hz, 6H, CH (CH3)2); 13C NMR (100 MHz, CDCl3) δ 171.2, 151.9, 147.2, 146.1, 145.4, 140.1, 137.4, 131.8, 127.6, 126.2, 123.8, 97.4, 53.5, 40.1, 35.1, 31.7, 28.2, 27.0, 24.4, 13.5; MS (ESI) m/z: 443 ([M+H]+). Anal. Calcd for C23H27ClN4O3; C, 62.37; H, 6.14; N, 12.65. Found: C, 62.35; H, 6.10; N, 12.66.
6-[N-(6-Chloro-3-pyridinylmethyl)-N-ethyl]amino-1-methyl-4-(2-methoxylphenyl)-5-nitro-1,2,3,4-tetrahydropyridin-2-one (3d): yield 78%; a yellow solid; mp 182-183 °C; IR (KBr, cm-1) 2990, 2968, 1708, 1580, 1430, 1281, 1089; 1H NMR (400 MHz, CDCl3) δ 8.38 (s, 1H, Py-H), 7.53 (s, 1H, Py-H), 7.27-7.29 (m, 1H, Py-H), 7.20-7.22 (m, 1H, benzene-H), 6.74-6.87 (m, 2H, benzene-H), 6.35 (s, 1H, benzene-H), 4.84 (s, 1H, benzene-CH), 4.45 (d, J = 14.6 Hz, 1H, Py-CH2), 4.23 (d, J = 14.6 Hz, 1H, Py-CH2), 3.82 (s, 3H), 3.16-3.19 (m, 2H), 3.11 (s, 3H, OCH3), 2.94-3.05 (m, 2H), 1.34 (t, J = 7.2 Hz, 3H, NCH2CH3); 13C NMR (100 MHz, CDCl3) δ 170.7, 159.8, 151.9, 149.1, 146.9, 138.8, 130.5, 128.9, 126.7, 125.8, 123.5, 120.7, 114.3, 98.9, 56.1, 53.9, 40.2, 35.8, 27.0, 19.5, 14.0; MS (ESI) m/z: 431 ([M+H]+). Anal. Calcd. for C21H23ClN4O4; C, 58.54; H, 5.38; N, 13.00. Found: C, 58.50; H, 5.36; N, 13.06.
6-[N-(6-Chloro-3-pyridinylmethyl)-N-ethyl]amino-1-methyl-4-(4-methoxylphenyl)-5-nitro-1,2,3,4-tetrahydropyridin-2-one (3e): yield 69%; a yellow solid; mp 176-177 °C; IR (KBr, cm-1) 2971, 2931, 1703, 1584, 1445, 1209, 1095; 1H NMR (400 MHz, CDCl3) δ 8.24 (s, 1H, Py-H), 7.20-7.42 (m, 2H, Py-H), 6.76-6.86 (m, 4H, benzene-H), 4.60 (s, 1H, benzene-CH), 4.39 (d, J = 14.6 Hz, 1H, Py-CH2), 4.19 (d, J = 14.6Hz, 1H, Py-CH2), 3.79 (s, 3H, OCH3), 3.10-3.14 (m, 2H), 3.05(s, 5H), 1.29 (t, J = 6.4 Hz, 3H, NCH2CH3); 13C NMR (100 MHz, CDCl3) δ 170.9, 161.4, 151.6, 148.1, 146.1, 138.2, 132.5, 130.8, 125.7, 120.9, 114.0, 97.9, 56.0, 53.4, 40.6, 35.2, 29.0, 26.8, 14.1; MS (EI) m/z: 431 ([M+H]+). Anal. Calcd for C21H23ClN4O4; C, 58.54; H, 5.38; N, 13.00. Found: C, 58.58; H, 5.34; N, 13.02.
6-[N-(6-Chloro-3-pyridinylmethyl)-N-ethyl]amino-1-methyl-4-(2-fluorophenyl)-5-nitro-1,2,3,4-tetra- hydropyridin-2-one (3f): yield 81%; yellow solid; mp 136-138 °C; IR (KBr, cm-1) 2992, 2970, 1706, 1572, 1436, 1279, 1091; 1H NMR (400 MHz, CDCl3) δ 8.25 (s, 1H, Py-H), 7.45 (s, 1H, Py-H), 7.18-7.26 (m, 3H, Py-H and benzene-H), 7.10 (s, 1H, benzene-H), 6.84 (s, 1H, benzene-H), 4.66-4.67 (m, 1H, benzene-CH), 4.41 (d, J = 14.7 Hz, 1H, Py-H), 4.16 (d, J = 14.7 Hz, 1H, Py-H), 3.13-3.14 (m, 2H), 3.02-3.11 (m, 5H), 1.30 (t, J = 7.5 Hz, 3H, NCH2CH3); 13C NMR (100 MHz, CDCl3) δ 169.8, 160.7, 151.8, 149.9, 148.2, 140.1, 130.8, 128.4, 127.9, 127.0, 124.0, 123.8, 115.4, 98.1, 53.1, 40.9, 35.1, 27.7, 18.6, 13.3; MS (ESI) m/z: 419 ([M+H]+). Anal. Calcd for C20H20ClFN4O3; C, 57.35; H, 4.81; N, 13.38. Found: C, 57.33; H, 4.80; N, 13.36.
6-[N-(6-Chloro-3-pyridinylmethyl)-N-ethyl]amino-1-methyl-4-(3-fluorophenyl)-5-nitro-1,2,3,4-tetra- hydropyridin-2-one (3g): yield 80%, a yellow solid; mp 146-148 °C; IR (KBr, cm-1) 2992, 2970, 1711, 1534, 1436, 1256, 1091; 1H NMR (400 MHz, CDCl3) δ 8.23 (s, 1H, Py-H), 7.42 (s, 1H, Py-H), 7.22-7.24 (m, 2H, Py-H and benzene-H), 6.92-6.97 (m, 1H, benzene-H), 6.76-6.78 (m, 2H, benzene-H), 4.68 (s, 1H, benzene-CH), 4.40 (d, J = 14.7 Hz, 1H, Py-CH2), 4.18 (d, J = 14.7 Hz, 1H, Py-CH2), 3.08-3.14 (m, 4H), 3.06 (s, 3H), 1.30 (t, J = 7.0 Hz, 3H, NCH2CH3); 13C NMR (100 MHz, CDCl3) δ 170.7, 162.0, 151.5, 149.9, 146.5, 141.8, 138.4, 131.8, 130.0, 124.2, 123.5, 114.9, 112.7, 98.0, 53.5, 40.5, 35.9, 28.7, 27.5, 13.2; MS (ESI) m/z: 419 ([M+H]+). Anal. Calcd for C20H20ClFN4O3; C, 57.35; H, 4.81; N, 13.38. Found: C, 57.31; H, 4.80; N, 13.35.
6-[N-(6-Chloro-3-pyridinylmethyl)-N-ethyl]amino-1-methyl-4-(4-fluorophenyl)-5-nitro-1,2,3,4-tetra- hydropyridin-2-one (3h): yield 84%; a yellow solid; mp 168-170 °C; IR (KBr, cm-1) 2994, 2973, 1702, 1578, 1432, 1274, 1090; 1H NMR (400MHz, CDCl3) δ 8.15 (s, 1H, Py-H), 7.32-7.40 (s, 1H, Py-H), 7.13-7.20 (m, 1H, Py-H), 6.80-6.88 (m, 4H, benzene-H), 4.56 (s, 1H, benzene-CH), 4.32 (d, J = 14.7 Hz, 1H, Py-CH2), 4.12 (d, J = 14.7 Hz, 1H, Py-CH2), 3.08 (s, 2H), 2.99-3.07 (m, 5H), 1.22 (t, J = 6.9 Hz, 3H, NCH2CH3); 13C NMR (100 MHz, CDCl3) δ 170.4, 159.3, 150.9, 148.9, 146.8, 139.2, 135.8, 131.5, 129.5, 123.5, 115.4, 98.5, 53.8, 40.9, 35.2, 29.8, 27.0, 13.4; MS (ESI) m/z: 419 ([M+H]+). Anal. Calcd for C20H20ClFN4O3; C, 57.35; H, 4.81; N, 13.38. Found: C, 57.38; H, 4.80; N, 13.37.
6-[N-(6-Chloro-3-pyridinylmethyl)-N-ethyl]amino-1-methyl-4-(2-chlorophenyl)-5-nitro-1,2,3,4-tetra- hydropyridin-2-one (3i): yield 71%, a yellow solid; mp 188-189 °C; IR (KBr, cm-1) 2993, 2971, 1706, 1576, 1436, 1274, 1091; 1H NMR (400MHz, CDCl3) δ 8.38 (s, 1H, Py-H), 7.54 (s, 1H, Py-H), 7.39 (d, J = 7.9 Hz, 1H, Py-H), 7.28 (d, J = 8.1 Hz, 1H, benzene-H), 7.19 (t, J = 7.7 Hz, 1H, benzene-H), 7.05 (t, J = 7.5 Hz, 1H, benzene-H), 6.25 (s, 1H, benzene-H), 4.92-4.94 (m, 1H, benzene-CH), 4.46 (d, J = 14.6 Hz, 1H, Py-CH2), 4.27 (d, J = 14.6 Hz, 1H, Py-CH2), 3.20-3.22 (m, 2H), 3.15 (s, 3H), 2.98-3.12 (m, 2H), 1.34 (t, J = 7.1 Hz, 3H, NCH2CH3); 13C NMR (100 MHz, CDCl3) δ 172.3, 152.1, 149.9, 146.1, 140.6, 139.1, 133.1, 131.2, 129.3, 128.8, 127.1, 126.5, 123.8, 98.1, 53.9, 40.1, 35.6, 27.5, 20.4, 13.2; MS (ESI) m/z: 435 ([M+H]+). Anal. Calcd for C20H20Cl2N4O3; C, 55.18; H, 4.63; N, 12.87. Found: C, 55.12; H, 4.64; N, 12.88.
6-[N-(6-Chloro-3-pyridinylmethyl)-N-ethyl]amino-1-methyl-4-(3-chlorophenyl)-5-nitro-1,2,3,4-tetra- hydropyridin-2-one (3j): yield 59%; a yellow solid; mp 56-57 °C; IR (KBr, cm-1) 2991, 2971, 1706, 1580, 1440, 1200, 1085; 1H NMR (400MHz, CDCl3) δ 8.25 (s, 1H, Py-H), 7.45 (s, 1H, Py-H), 7.18-7.26 (m, 3H, Py-H and benzene), 7.10 (s, 1H, benzene-H), 6.84 (s, 1H, benzene-H), 4.66-4.67 (m, 1H, benzene-CH), 4.41 (d, J = 14.7 Hz, 1H, Py-CH2), 4.16 (d, J = 14.7 Hz, 1H, Py-CH2), 3.13-3.14 (m, 2H), 3.02-3.11 (m, 5H), 1.30 (t, J = 7.5 Hz, 3H, NCH2CH3); 13C NMR (100 MHz, CDCl3) δ 168.7, 152.3, 148.4, 145.8, 141.6, 139.2, 133.7, 131.8, 129.9, 128.4, 126.5, 126.0, 121.2, 98.2, 53.9, 40.5, 35.8, 28.9, 27.5, 13.0; MS (ESI) m/z: 435 ([M+H]+). Anal. Calcd for C20H20Cl2N4O3; C, 55.18; H, 4.63; N, 12.87. Found: C, 55.17; H, 4.67; N, 12.88.6-[N-(6-Chloro-3-pyridinylmethyl)-N-ethyl]amino-1-methyl-4-(4-chlorophenyl)-5-nitro-1,2,3,4-tetra- hydropyridin-2-one (3k): yield 75%; a yellow solid; mp 180-182 °C; IR (KBr, cm-1) 2991, 2970, 1708, 1577, 1436, 1274, 1095; 1H NMR (400MHz, CDCl3) δ 8.15 (s, 1H, Py-H), 7.31 (s, 1H, Py-H), 7.15-7.20 (m, 3H, Py-H and benzene-H), 6.85 (s, 2H, benzene-H), 4.57 (s, 1H, benzene-CH), 4.32 (d, J = 14.7 Hz, 1H, Py-CH2), 4.12 (d, J = 14.7 Hz, 1H, Py-CH2), 3.05-3.08 (m, 2H), 2.96-3.00 (m, 5H), 1.22 (t, J = 6.2 Hz, 3H, NCH2CH3); 13C NMR (100 MHz, CDCl3) δ 170.2, 150.8, 148.6, 145.8, 140.2, 138.3, 130.8, 131.0, 129.4, 128.8, 123.9, 98.6, 53.2, 40.0, 35.2, 29.2, 27.8, 14.3; MS (ESI) m/z: 435 ([M+H]+). Anal.Calcd for C20H20Cl2N4O3; C, 55.18; H, 4.63; N, 12.87. Found: C, 55.16; H, 4.60; N, 12.87.
6-[N-(6-Chloro-3-pyridinylmethyl)-N-ethyl]amino-1-methyl-4-(4-bromophenyl)-5-nitro-1,2,3,4-tetra-hydropyridin-2-one (3l): yield 87%; a yellow solid; mp 205-206 °C; IR (KBr, cm-1) 2991, 2970, 1704, 1579, 1436, 1273, 1095; 1H NMR (400MHz, CDCl3) δ 8.25 (s, 1H, Py-H), 7.45 (s, 1H, Py-H), 7.18-7.26 (m, 3H, Py-H and benzene-H), 7.10 (s, 1H, benzene-H), 6.84 (s, 1H, benzene-H), 4.66-4.67 (m, 1H, benzene-CH), 4.41 (d, J = 14.7 Hz, 1H, Py-CH2), 4.16 (d, J = 14.7 Hz, 1H, Py-CH2), 3.13-3.14 (m, 2H), 3.02-3.11 (m, 5H), 1.30 (t, J = 7.5 Hz, 3H, NCH2CH3); 13C NMR (100 MHz, CDCl3) δ 170.9, 152.6, 150.2, 149.4, 146.1, 140.3, 132.2, 131.4, 130.5, 123.8, 120.5, 98.0, 53.5, 40.1, 35.7, 29.2, 27.9, 13.0; MS (ESI) m/z: 479 ([M+H]+). Anal. Calcd for C20H20BrClN4O3; C, 50.07; H, 4.20; N, 11.68. Found: C, 50.06; H, 4.20; N, 11.67.
6-[N-(6-Chloro-3-pyridinylmethyl)-N-ethyl]amino-1-methyl-4-(4-cyanophenyl)-5-nitro-1,2,3,4-tetra- hydropyridin-2-one(3m): yield 71%; a yellow solid; mp 96-98 °C; IR (KBr, cm-1) 2995, 2978, 1702, 1578, 1438, 1211, 1065; 1H NMR (400MHz, CDCl3) δ 8.18 (s, 1H, Py-H), 7.35-7.56 (m, 3H, Py-H and benzene-H), 7.07-7.19 (m, 3H, benzene-H), 4.70 (s, 1H, benzene-CH), 4.37 (d, J = 14.6 Hz, 1H, Py-CH2), 4.23 (d, J = 14.6 Hz, 1H, Py-CH2), 3.16-3.20 (m, 2H), 3.09-3.12 (m, 5H), 1.31 (t, J = 6.5 Hz, 3H, NCH2CH3); 13C NMR (100 MHz, CDCl3) δ 170.2, 151.8, 149.8, 145.4, 144.3, 140.2, 131.4, 129.4, 128.6, 125.7, 116.5, 109.8, 99.1, 53.5, 40.8, 36.2, 28.8, 28.2, 14.3; MS (ESI) m/z: 426 ([M+H]+). Anal. Calcd for C21H20ClN5O3; C, 59.23; H, 4.73; N, 16.44. Found: C, 59.26; H, 4.72; N, 16.45.
6-[N-(6-Chloro-3-pyridinylmethyl)-N-ethyl]amino-1-methyl-4-(4-trifluoromethylphenyl)-5-nitro-1,2,3,4-tetrahydropyridin-2-one (3n): yield 86 %; a yellow solid; mp 152-153 °C; IR (KBr, cm-1) 2992, 2970, 1706, 1572, 1436, 1279, 1091; 1H NMR (400MHz, CDCl3) δ 8.45 (s, 1H, Py-H), 7.75 (s, 1H, Py-H), 7.51-7.5 4 (m, 2H, Py-H and benzene-H), 7.35-7.39 (s, 1H, benzene-H), 7.08-7.20 (m, 2H, benzene-H), 4.72 (s, 1H, benzene-CH), 4.40 (d, J = 14.8 Hz, 1H, Py-CH2), 4.22 (d, J = 14.8 Hz, 1H, Py-CH2), 3.11-3.12 (m, 2H), 3.06-3.07 (m, 5H), 1.26 (t, J = 7.1 Hz, 3H, NCH2CH3); 13C NMR (100 MHz, CDCl3) δ 171.5, 150.2, 148.3, 146.1, 143.5, 139.1, 131.8, 128.4, 127.9, 125.2, 122.8, 119.3, 98.1, 53.4, 40.6, 35.8, 29.8, 27.5, 13.9; MS (ESI) m/z: 469 ([M+H]+). Anal. Calcd for C21H20ClF3N4O3; C, 53.30; H, 4.30; N, 11.95. Found: C, 53.35; H, 4.31; N, 11.98.
6-[N-(6-Chloro-3-pyridinylmethyl)-N-ethyl]amino-1-methyl-4-(4-nitrophenyl)-5-nitro-1,2,3,4-tetra- hydropyridin-2-one (3o): yield 89%; a yellow solid; mp 200-201 °C; IR (KBr, cm-1) 2990, 2975, 1702, 1578, 1432, 1274, 1092; 1H NMR (400MHz, CDCl3) δ 8.19 (s, 1H, Py-H), 8.13 (s, 1H, Py-H), 8.11 (s, 1H, Py-H), 7.39 (s, 1H, benzene-H), 7.17-7.19 (m, 3H, benzene-H), 4.75 (s, 1H, benzene-CH), 4.41 (d, J = 14.7 Hz, 1H, Py-CH2), 4.23 (d, J = 14.7 Hz, 1H, Py-CH2), 3.13-3.20 (m, 4H), 3.10 (s, 3H), 1.31 (t, J = 7.2 Hz, 3H, NCH2CH3); 13C NMR (100 MHz, CDCl3) δ 172.5, 151.8, 149.9, 146.8, 146.2, 145.6, 139.2, 131.2, 128.4, 123.7, 123.1, 98.2, 53.6, 40.9, 35.8, 29.2, 27.8, 13.2; MS (ESI) m/z: 446 ([M+H]+). Anal. Calcd for C20H20ClN5O5; C, 53.88; H, 4.52; N, 15.71. Found: C, 53.85; H, 4.55; N, 15.77.
6-[N-(6-Chloro-3-pyridinylmethyl)-N-ethyl]amino-1-methyl-4-(2,4-dichlorophenyl)-5-nitro-1,2,3,4- tetrahydropyridin-2-one (3p): yield 76%; a yellow solid; mp 178-180 °C; IR (KBr, cm-1) 2998, 2973, 1702, 1578, 1432, 1270, 1092; 1H NMR (400 MHz, CDCl3) δ 8.38 (s, 1H, Py-H), 7.51 (s, 1H, Py-H), 7.41 (s, 1H, Py-H), 7.03-7.30 (m, 2H, benzene-H), 6.07-6.09 (s, 1H, benzene-H), 4.85 (s, 1H, benzene-CH), 4.46 (d, J = 14.6 Hz, 1H, Py-CH2), 4.28 (d, J = 14.6 Hz, 1H, Py-CH2), 3.21-3.28 (m, 2H), 3.16 (s, 3H), 3.08 (d, J = 16.7 Hz, 1H), 2.97 (d, J = 16.7 Hz, 1H), 1.35 (t, J = 7.1 Hz, 3H, NCH2CH3); 13C NMR (100 MHz, CDCl3) δ 171.2, 152.1, 149.2, 145.2, 139.2, 138.7, 134.6, 132.4, 131.1, 130.7, 129.2, 126.9, 121.6, 100.4, 54.8, 41.2, 35.6, 26.5, 20.3, 13.2; MS (ESI) m/z: 469 ([M+H]+). Anal. Calcd for C20H19Cl3N4O3; C, 51.14; H, 4.08; N, 11.93. Found: C, 51.15; H, 4.10; N, 11.96.
6-[N-(6-Chloro-3-pyridinylmethyl)-N-ethyl]amino-1-methyl-4-(3,4-dichlorophenyl)-5-nitro-1,2,3,4-tetrahydropyridin-2-one (3q): yield 83%; a yellow solid; mp 157-158 °C; IR (KBr, cm-1) 2994, 2973, 1702, 1578, 1432, 1274, 1090; 1H NMR (400 MHz, CDCl3) δ 8.17 (s, 1H, Py-H), 7.36 (s, 1H, Py-H), 7.27 (d, J = 8.3 Hz, 1H, Py-H), 7.14-7.20 (m, 2H, benzene-H), 6.73 (s, 1H, benzene-H), 4.56-4.58 (m, 1H, benzene-CH), 4.34 (d, J = 14.7 Hz, 1H, Py-CH2), 4.09 (d, J = 14.7 Hz, 1H, Py-CH2), 3.05-3.07 (m, 2H), 2.98-3.02 (m, 5H), 1.23 (t, J = 7.2Hz, 3H, NCH2CH3); 13C NMR (100 MHz, CDCl3) δ 171.5, 153.9, 149.2, 145.8, 139.7, 138.0, 133.5, 132.4, 131.6, 130.2, 129.7, 127.4, 122.6, 99.3, 53.5, 42.2, 35.3, 28.9, 26.4, 13.2; MS (ESI) m/z: 469 ([M+H]+). Anal. Calcd for C20H19Cl3N4O3; C, 51.14; H, 4.08; N, 11.93. Found: C, 51.13; H, 4.11; N, 11.96.
X-Ray Crystallography
A yellow crystal of compound 3g was recrystallized by slow evaporation from a mixed solution of EtOAc/petroleum ether (v:v = 1:5), with dimensions of 0.16 mm x 0.12 mm x 0.10 mm was mounted on a glass fiber for data collection which was made on a BRUKER SMART APEX 1000 CCD diffractometer equipped with a graphite-monochromatic MoKα radiation (λ = 0.71073 Å) by using a φ-ω scan mode in the range of 1.82≤θ≤25.50° (-10≤h≤13, -23≤k≤23, -10≤l≤10) at 298(2) K. A total of 10184 reflections were collected with 3672 unique ones (Rint = 0.0565), of which 2051 with I > 2σ(I) were considered as observed and used in the succeeding refinements. The intensity data were corrected for Lp factors and empirical absorption. The final R = 0.0594, wR = 0.1275 (w = 1/[σ2(Fo2) + (0.0621P)2 + 0.000P], where P = (Fo2 + 2Fc2)/3), S = 1.000, (Δ/σ)max = 0.001, (Δρ)max = 0.351 and (Δρ)min = –0.266 e/Å3. The structural graphics was drawn with SHELXTL-97 software package. Other details of the structure have been deposited with the Cambridge CrystallographicData Centre, CCDC 825017.
ACKNOWLEDGEMENTS
We are grateful for the insecticidal activity test of the target compounds from Bioassay Department, Branch of National Pesticide R&D South Center, Hangzhou and the support from Branch of National Pesticide R&D South Center. This work was supported by the National Natural Science Foundation of China (21102092), the Key Scientific "Twelfth Five-Year" National Technology Support Program (2011BAE06B01-17), the Innovation Project of Shanghai Education Commission (12YZ078), the Program of the Food Safety and Nutrition Innovation Team of Shanghai Normal University (DXL123), Shanghai Key Laboratory of Rare Earth Functional Materials, Shanghai Normal University (07dz22303).
References
1. P. Jeschke, R. Nauen, M. Schindler, and A. Elbert, J. Agric. Food Chem., 2011, 59, 2897. CrossRef
2. M. Tomizawa and J. E. Casida, Annu. Rev. Pharmacol. Toxicol., 2005, 45, 247. CrossRef
3. K. Matsuda, M. Shimomura, M. Ihara, M. Akamatsu, and D. B. Sattelle, Biosci. Biotechnol. Biochem., 2005, 69, 1442. CrossRef
4. I. Ohno, M. Tomizawa, K. A. Durkin, Y. Naruse, J. E. Casida, and S. Kagabu, Chem. Res.Toxicol., 2009, 22, 476. CrossRef
5. D. Bai, S. C. R. Lummis, W. Leicht, H. Breer, and D. B. Sattelle, Pest. Manage. Sci., 1991, 33, 197.. CrossRef
6. M. Liu and J. E. Casida, Pestic. Biochem. Physiol., 1993, 46, 40. CrossRef
7. K. Nishimura, Y. Kanda, A. Okazawa, and T. Ueno, Pestic. Biochem. Physiol., 1994, 50, 51. CrossRef
8. K. Mori, T. Okumoto, N. Kawahara, and Y. Ozoe, Pest. Manage. Sci., 2001, 58, 190. CrossRef
9. R. Nauen and I. Denholm, Insect. Biochem. Physiol., 2005, 58, 200. CrossRef
10. R. Nauen and A. Elbert, Pest. Manag. Sci., 2000, 56, 60. CrossRef
11. M. Reyes, P. Franck, P. J. Charmillot, C. Ioriatti, J. Olivares, E. Pasqualini, and B. Sauphanor, Pest. Manag. Sci., 2007, 63, 890. CrossRef
12. Z. W. Liu, Z. J. Han, Y. C. Wang, L. C. Zhang, H. W. Zhang, and C. Liu, Pest. Manag. Sci., 2003, 59, 1355. CrossRef
13. K. D. Ninsin, Pest. Manag. Sci., 2004, 60, 839. CrossRef
14. D. M. Sanchez, R. M. Hollingworth, E. J. Grafius, and D. D. Moyer, Pest. Manag. Sci., 2006, 62, 30. CrossRef
15. K. Gorman, G. Devine, J. Bennison, P. Coussons, N. Punchard, and L. Denholm, Pest. Manag. Sci., 2007, 63, 555. CrossRef
16. M. Kristensen and J. B. Jespersen. Pest. Manag. Sci., 2008, 64, 126. CrossRef
17. X. S. Shao, W. W. Zhang, Y. Q. Peng, Z. Li, Z. Z. Tian, and X. H. Qian, Bioorg. Med. Chem. Lett., 2008, 18, 6513. CrossRef
18. X. S. Shao, Z. Li, X. H. Qian, and X. Y. Xu, J. Agric. Food Chem., 2009, 57, 951. CrossRef
19. X. S. Shao, H. Fu, X. Y. Xu, X. L. Xu, Z. W. Liu, Z. Li, and X. H. Qian, J. Agric. Food Chem., 2010, 58, 2696. CrossRef
20. C. W. Sun, D. R. Yang, J. H. Xing, H. F. Wang, J. Jin, and J. Zhu, J. Agric. Food Chem., 2010, 58, 3415. CrossRef
21. C. W. Sun, X. Xu, Y. H. Xu, D. L.Yang, T. Fang, and T. Y. Liu. J. Agric. Food Chem., 2011, 59, 4828. CrossRef
22. C. W. Sun, J. Jin, D. R. Yang, J. H. Xing, H. F. Wang, and J. Zhu, Bioorg. Med. Chem. Lett., 2010, 20, 3301. CrossRef
23. X. B. Ma, A. Q. Yin, and S. J. Xue, J. Shanghai Normal University (Natural science), 2011, 40, 41.
24. A. S. Ivanov, Chem. Soc. Rev., 2008, 37, 789. CrossRef
25. A. M. Dumas and E. Fillion. Acc. Chem. Res., 2010, 43, 440. CrossRef
26. J. Quiroga, A. Hormaza, and B. Insuasty, J. Heterocycl. Chem., 1997, 34, 521. CrossRef
27. S. J. Tu, S. L. Zhu, Z. Shao, X. Zou, S. J. Ji, and Y. Zhong, Chin. J. Org. Chem., 2005, 25, 987.
28. Z. G. Han, C. B. Miao, F. Shi, N. Ma, G. Zhang, and S. J. Tu, J. Comb. Chem., 2010, 12, 16. CrossRef
29. S. Y. Lu, X. S. Shao, Z. Li, Z. P. Xu, S. S. Zhao, Y. L. Wu, and X. Y. Xu, J. Agric. Food Chem., 2012, 60, 322. CrossRef
30. A. C. Shaikh and C. P. Chen, Bioorg. Med. Chem. Lett., 2010, 20, 3664. CrossRef
31. L. Z. Xu, Z. Yang, X. Yi, and G. W. An, Acta. Cryst., 2008, E64, o1074. CrossRef
32. M. Ihara, T. Okajima, and A. Yamashita, Invertebr. Neurosci., 2008, 8, 71. CrossRef