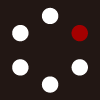
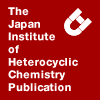
HETEROCYCLES
An International Journal for Reviews and Communications in Heterocyclic ChemistryWeb Edition ISSN: 1881-0942
Published online by The Japan Institute of Heterocyclic Chemistry
e-Journal
Full Text HTML
Received, 27th January, 2014, Accepted, 17th March, 2014, Published online, 26th March, 2014.
DOI: 10.3987/COM-14-S(K)4
■ Formation of 2-Acetamido-2-deoxy-D-glucopyranosidic Linkages via Glycosidation Using a Combination of Two Lewis Acids
Yoshiki Oda, Masanobu Midorikawa, and Takashi Yamanoi*
Research Department, The Noguchi Institute, 1-8-1, Kaga, Itabashi-ku, Tokyo 173-0003, Japan
Abstract
A mixed activation system composed of ytterbium(III) triflate and a catalytic boron trifluoride diethyl etherate complex efficiently promotes the glycosylation of various alcohol acceptors using 2-acetamido-3,4,6-tri-O-benzyl-2-deoxy-α-D-glucopyranosyl acetate in dichloromethane at room temperature to afford 2-acetamido-2-deoxy-D-glucopyranosides in good yields with significant formation of the α-isomers. Notably, stereoselective glycosylations of phenol derivatives as the acceptors afforded aryl 1,2-cis-α-glycosides without the formation of any β-isomers. This highly stereocontrolled 1,2-cis-α-glycosidation was applied to the synthesis of a novel hydroquinone α-glycoside.INTRODUCTION
Glycosidation is an indispensable tool for glycoside synthesis; however, identifying appropriate glycosyl donors and activating agents is a major challenge for the development of glycosidation methods. Lewis acids are probably the most widely used activators for glycosidation. Among them, ytterbium(III) triflate (Yb(OTf)3) is known to be a powerful activator for a few glycosidation reactions.1 We previously showed that Yb(OTf)3 is effective with several glycosyl acetates.2 Several glycosidation methods using a combination of two Lewis acids such as bismuth(III) triflate-boron trifluroride diethyletherate (BF3·OEt2),3 silver perchlorate-lithium perchlorate,4 and Yb(OTf)3-zinc chloride5 have been reported. In addition, we recently showed that a mixed activating system based on Yb(OTf)3 and a catalytic amount of BF3·OEt2 was useful for glycosidation using certain less reactive glycosyl acetates.6 When our attention was directed toward glycosidation using 2-acetamido-3,4,6-tri-O-benzyl-2-deoxy-α-D-glucopyranosyl acetate (1),7 which is a less reactive glycosyl donor, the use of the mixed Yb(OTf)3-BF3·OEt2 activating system exhibited interesting glycosyl acceptor specificities,8 i.e., the glycosidation using certain aliphatic alcohols afforded the corresponding 2-acetamido-2-deoxy-D-glucopyranosides with a considerable amount of formation of the α-isomers, while glycosidations of phenol derivatives proceeded stereoselectively, affording aryl α-glycosides without the formation of any β-isomers.
The 2-acetamido-2-deoxy-α-D-glucopyranosidic linkage is involved in some glycosidic natural products such as O-glycans of gastric mucins,9 lipopolysaccharides of bacteria,10 and tunicamycin11,12 Therefore, the development of a convenient method for the formation of the 1,2-cis-α-glycosidic linkage directly from 2-acetamido-2-deoxy-D-glucopyranose derivatives is an important goal in synthetic carbohydrate chemistry.13 Herein, we describe in detail the formation of the 2-acetamido-2-deoxy-α-D-glucopyranosidic linkage based on the glycosyl acceptor properties for glycosidations using 1 with a mixed activation system composed of Yb(OTf)3-BF3·OEt2. To demonstrate the applicability of the method, the synthesis of a novel hydroquinone α-glycoside was achieved using the highly stereocontrolled 1,2-cis-aryl-α-glycosidation.
RESULTS AND DISCUSSION
Glycosidation using 1 with the mixed activating system composed of Yb(OTf)3 (1 equiv.)-BF3·OEt2 (0.03 equiv.) was first investigated with phenethyl alcohol (2) as the substrate (Scheme 1). This reaction, which proceeded in CH2Cl2 overnight at room temperature, gave the desired phenethyl 2-acetamido-3,4,6-tri-O-benzyl-2-deoxy-D-glucopyranoside (3) in a high yield of 95% with an α/β ratio of 40/60 (Entry 1, Table 1). Interestingly, this reaction resulted in the formation of a considerable amount of α-glycoside. This finding that the 1,2-cis-α-glycosidic linkage was directly formed from a 2-acetamido-2-deoxy-D-glucopyranosyl donor was very rare. It is known that glycosidations using 2-acetamido-2-deoxy-D-glucopyranosyl donors bearing a natural N-acetyl protecting group typically form the corresponding β-glycosides via SN2-like nucleophilic substitution of alcohols with in situ generated oxazoline derivatives (or oxazolinium cation intermediates).14 Therefore, this unusual stereoselectivity of the glycosidation between 1 and 2 using our mixed activating system suggests that the reaction may proceed via a different pathway that does not involve the generation of an oxazoline intermediate.
A catalytic amount of BF3·OEt2 is essential for promoting the glycosidation because the reaction barely proceeds without added BF3·OEt2 (Entry 2). We speculate that the ROH-BF3 complex formed in situ between BF3·OEt2 and the alcohol acceptor acts as a Brønsted acid and influences the glycosidation reactivity.15 The effect of the Brønsted acids, acetic acid (AcOH) and triflic acid (TfOH), as additives was thus examined (Entries 3 and 4). The addition of 3 mol% of AcOH and TfOH to the glycosidation reaction using 1 (1.2 equiv.), phenethyl alcohol (1 equiv.), and Yb(OTf)3 (1 equiv.) in CH2Cl2 at room temperature produced 3 in 72% and 68% yield with α/β ratios of 49/51 and 47/53, respectively. The addition of AcOH and TfOH was also effective for the promotion of glycosidations using 1 and BF3·OEt2, and these reactions also afforded a considerable amount of the α-glycoside.
Next, we investigated the glycosidation of several types of alcohols under similar reaction conditions using 1 in order to examine the effect of the acceptor on the stereoselectivity of the reaction. Primary and secondary alkyl alcohols 2, 4–11 gave the corresponding glycosides 3, 17–24, respectively, in good yields with α/β ratios of ca. 1/1–1/5 (Entries 1 and 5–12, Table 1). Thus, each of these reactions gave a mixture of α- and β-glycosides. However, surprisingly, the reactions using phenol derivatives 12–16 gave aryl α-glycosides 25–29, respectively, in good yields with no formation of the β-glycosides (Entries 13–17). The steric and electronic effects of the phenol derivatives may strongly influence the α-stereoselectivity of the reaction. The configuration of the glycosidic bond of these glycosides was determined based on the coupling constant values in their 1H-NMR spectra (α form: J = 3.3–4.1 Hz; β form: J = 7.2–8.4 Hz).
In addition, we confirmed that the β-anomer of 25 was not isomerized to the α-anomer under acidic conditions in the presence of Yb(OTf)3 (1 equiv.) and BF3·OEt2 (0.03 equiv.) in CH2Cl2 at room temperature. Therefore, the α-glycosides formed in the glycosidation using 1 are not conversion products of in situ acid-catalyzed anomerization of the β-glycosides.
Furthermore, to clarify the difference in the reaction mechanism, we performed glycosidations using 3,4,6-tri-O-benzyl-1,2-oxazoline-glucopyranose (30),16 which appeared to at least partially proceed via an in situ generated intermediate under the same conditions (see Table 2). The reaction of 30 (1.2 equiv.) with 2 and 12 was examined in the presence of Yb(OTf)3 (1 equiv.) and BF3·OEt2 (0.03 equiv.) in CH2Cl2 at room temperature. Acceptor 2 afforded the corresponding β-glycoside in 53% yield with no formation of the α-glycoside (Entry 18), while phenol (12) did not react at all (Entry 19). Thus, the reactivity and stereoselectivity of the glycosidation using 30 were found to be quite different from those of the glycosidation using 1.
We speculate that the complexation of ytterbium metal to the carbonyl group of 1 may generate a glycosyl cation intermediate, as shown in Scheme 2. Formation of this complex would prevent the generation of the oxazoline derivative because of the decrease in the Lewis basicity of the oxygen atom of the carbonyl group, thus allowing the alcohol to attack the glycosyl cation intermediate from the α-face to form the 1,2-cis-α-glycosidic linkage.17 The formation of 1,2-trans-β-glycosides during the glycosidation using alkyl alcohols can be explained by the β-attack of the alcohol on the glycosyl cation intermediate or the in situ generated oxazoline derivative. However, we have not yet found a sufficient explanation for the high α-stereoselectivities of the glycosidations using phenol derivatives.
Finally, the 1,2-cis-α-aryl-D-glycosidation method was applied to the synthesis of the novel hydroquinone α-glycoside 4-hydroxyphenyl 2-acetamido-2-deoxy-α-D-glucopyranoside (31)18; 31 is an analogue of arbutin (4-hydroxyphenyl D-glucopyranoside) (32), which exhibits inhibitory activity against tyrosinase, a key enzyme for the synthesis of melanin, the overproduction of which results in hyperpigmentation in the epidermis. Notably, the α-anomeric isomer of 32 has stronger inhibitory activity against mammalian tyrosinases than the β-anomeric isomer.19 As a result, the synthesis of α-arbutin analogues and the investigation of their performance as skin-lightening agents has recently attracted attention.20
Hydroquinone (33) has been utilized as a glycosyl acceptor for the preparation of several hydroquinone glycosides using both enzymatic and chemical glycosylation methods.21 We conducted a detailed investigation of the glycosidation of 33 using 1, including evaluation of the by-products formed in the reaction (Scheme 3). When the Yb(OTf)3 (1 equiv.)-BF3·OEt2 (0.03 equiv.) mixed activating system was used in CH2Cl2 for 1 h at room temperature, the desired hydroquinone α-glycoside 35 was obtained in only 18% yield. However, the production of its β-anomer isomer was not observed at all. Further, 1 was recovered in 45% yield, and 30 was produced in 15% yield (Entry 1, Table 2). These results suggested that the low yield was due to the poor solubility of 33 in CH2Cl2. However, when the reaction was run in a mixed CH2Cl2/Et2O (v/v= 1/1) solvent in which 33 was soluble, only a trace amount of the desired 35 was formed (Entry 2). Thus, the use of Et2O inhibited the glycosylation.
Next, 4-tert-butoxyphenol (34) was utilized as the glycosyl acceptor; 34 was observed to be moderately soluble in CH2Cl2, and the Lewis acid glycosylation promoter was expected to cleave the tert-butyl group during the reaction. Rewardingly, the desired 35 was obtained in 66% yield using Yb(OTf)3 (1 equiv.)-BF3·OEt2 (0.03 equiv.) in CH2Cl2 for 1 h at room temperature (Entry 3). Interestingly, the glycoside 36 with the tert-butyl group retained was also obtained in 8% yield. Thus, the total yield of the glycoside products in this reaction reached 74%. In addition, 30 was formed in 21% yield. When the reaction was allowed to proceed for 6 h, no 36 remained; however, 30 and 37 were produced as byproducts in 22% and 10% yields, respectively (Entry 4). The deprotection of the benzyl groups of 35 using Pd(OH)2 in THF-MeOH yielded an excellent quantity of desired 31.
In summary, several 2-acetamido-2-deoxy-D-glucopyranosides were directly synthesized from glycosyl acetate 1 in good yields with the formation of a considerable amount of α-isomers using a Yb(OTf)3-catalytic BF3·OEt2 mixed activating system. Notably, reactions of phenol derivatives as acceptors only afforded aryl α-glycosides, with no formation of β-glycosides. The synthesis of the novel hydroquinone 2-acetamido-2-deoxy-α-D-glucopyranoside as an α-arbutin analogue was also successfully achieved using the highly stereocontrolled 1,2-cis-α-aryl-D-glycosidation method.
EXPERIMENTAL
1H-NMR (600 MHz) and 13C-NMR (150 MHz) spectra were recorded using a JEOL ECA-600 spectrometer. Melting points were determined using B-545 (BÜCHI Labortechnik AG) and are uncorrected. Optical rotations were recorded using a JASCO DIP-360 digital polarimeter. Preparative TLC was performed on Merck silica gel 60GF254. Column chromatography was conducted using silica gel 60N (40–50 μm, Kanto Chemical Co. Inc.). All anhydrous solvents were purified according to standard methods.
Typical glycosidation procedure
Yb(OTf)3 (98.2 mg, 0.2 mmol) was added to a solution of 1 (101.4 mg, 0.2 mmol), 2 (19.34 mg, 0.2 mmol), and BF3·OEt2 (47 μL, 0.005 mmol) in CH2Cl2 (3 mL) at 0 °C, and the resulting mixture was stirred for 16 h at room temperature. The reaction was then quenched by the addition of sat. aq. NaHCO3 solution (5 mL), and the reaction mixture was extracted with CH2Cl2. The organic layer was then washed with water and sat. aq. NaCl solution and dried over Na2SO4, the solvent. The crude product obtained after evaporation of the solvent under reduced pressure was purified via preparative silica gel TLC (EtOAc/hexane = 2/1) to give 3 (95% yield, α form; 35.7 mg, β form; 53.7 mg, α/β ratio = 40/60) as white crystals.
Phenethyl 2-Acetamido-3,4,6-tri-O-benzyl-2-deoxy-D-glucopyranoside (3)
α Form; Rf 0.48 (EtOAc/hexane = 2/1); mp 152.5–154.5 °C; [α]25D +72.5 (c 1.0, CHCl3); 1H NMR (600 MHz, CDCl3): δ 1.62 (3H, s, CH3), 2.84 (2H, m, CH2CH2Ph), 3.35 (1H, dt, J = 6.7 Hz, J = 8.9 Hz, CHaHbCH2Ph), 3.63–3.65 (2H, m, H-3, Ha-6), 3.69–3.72 (3H, m, H-4, H-5, Hb-6), 3.94 (1H, dt, J = 5.7 Hz, J = 9.6 Hz, CHaHbCH2Ph), 4.18 (1H, dt, J = 3.6 Hz, J = 10.0 Hz, H-2), 4.35–4.84 (6H, m, CH2Ph), 4.68 (1H, d, J = 3.6 Hz, H-1), 4.93 (1H, d, J = 9.5 Hz, NH), 7.10–7.31 (20H, m, Ph); 13C NMR (150 MHz, CDCl3): δ 23.3 (CH3), 35.8 (CH2CH2Ph), 52.3 (C-2), 68.2 (CH2CH2Ph), 68.5 (C-6), 71.0 (C-5), 73.4–74.9 (CH2Ph), 78.3 (C-4), 80.1 (C-3), 97.7 (C-1), 126.3 (Ph), 127.6-128.8 (Ph), 128.8 (Ph), 138.9 (Ph), 169.6 (C=O), 138.0–138.4 (Ph); HRMS (ESI): m/z calcd for C37H41NO6Na+: 618.2826; found: 618.2828. β Form; Rf 0.65 (EtOAc/hexane = 2/1); mp 154.7–157.5 °C; [α]25D +10.3 (c 1.0, CHCl3); 1H NMR (600 MHz, CDCl3): δ 1.73 (3H, s, CH3), 2.87 (2H, m, CH2CH2Ph), 3.44 (1H, m, H-2), 3.56 (1H, m, H-5), 3.62 (1H, t, J = 8.9 Hz, H-4), 3.65–3.75 (3H, m, H-6, CHaHbCH2Ph), 4.05 (1H, t, J = 8.9 Hz, H-3), 4.10 (1H, dt, J = 6.5 Hz, J = 8.9 Hz, CHaHbCH2Ph), 4.51–4.80 (6H, m, CH2Ph), 4.76 (1H, d, J = 7.6 Hz, H-1), 5.45 (1H, d, J = 7.6 Hz, NH), 7.18–7.33 (20H, m, Ph); 13C NMR (150 MHz, CDCl3): δ 23.5 (CH3), 36.0 (CH2CH2Ph), 56.7 (C-2), 69.0 (C-6), 70.0 (CH2CH2Ph), 73.4–74.5 (CH2Ph), 74.7 (C-5), 78.5 (C-4), 80.4 (C-3), 99.8 (C-1), 126.1 (Ph), 127.6-128.7 (Ph), 129.0 (Ph), 138.8 (Ph), 170.2 (C=O), 138.0-138.4 (Ph); HRMS (ESI): m/z calcd for C37H41NO6Na+: 618.2826; found: 648.2823.
Propyl 2-Acetamido-3,4,6-tri-O-benzyl-2-deoxy-D-glucopyranoside (17)
Using the same procedure as described above for 3, Yb(OTf)3 (123.3 mg, 0.2 mmol) was added to a solution of 1 (127.3 mg, 0.2 mmol), 4 (12.0 mg, 0.2 mmol), and BF3·OEt2 (60 μL, 0.006 mmol) in CH2Cl2 (3 mL) to give 17 (PTLC; EtOAc/hexane = 2/1, 95% yield, α form; 16.9 mg, β form; 71.5 mg, α/β ratio = 19/81) as white crystals. α Form; Rf 0.50 (EtOAc/hexane = 2/1); mp 142.0–142.1 °C; [α]26D +102.7 (c 0.34, CHCl3); 1H NMR (600 MHz, CDCl3): δ 0.90 (3H, t, J = 7.4 Hz, CH2CH3), 1.57 (2H, m, CH2CH3), 1.84 (3H, s, CH3), 3.34 (1H, dt, J = 6.6 Hz, J = 9.8 Hz, CHaHbCH2CH3), 3.61 (1H, dt, J = 6.8 Hz, J = 9.6 Hz, CHaHbCH2CH3), 3.68 (1H, dd, J = 1.5 Hz, J = 10.4 Hz, Ha-6), 3.69 (1H, t, J = 9.5 Hz, H-3), 3.70 (1H, t, J = 9.2 Hz, H-4), 3.78 (1H, dd, J = 1.8 Hz, J = 11.3 Hz, Hb-6), 3.79 (1H, m, H-5), 4.26 (1H, dt, J = 3.7 Hz, J = 9.9 Hz, H-2), 4.51–4.86 (6H, m, CH2Ph), 4.78 (1H, d, J = 3.6 Hz, H-1), 5.28 (1H, d, J = 9.5 Hz, NH), 7.17-7.35 (15H, m, Ph); 13C NMR (150 MHz, CDCl3): δ 10.6 (CH2CH3), 22.7 (CH2CH3), 23.5 (CH3), 52.6 (C-2), 68.6 (C-6), 69.4 (CH2CH2CH3), 71.0 (C-5), 73.5–75.1 (CH2Ph), 78.5 (C-4), 80.5 (C-3), 97.5 (C-1), 127.6–128.5 (Ph), 138.1-138.5 (Ph), 169.6 (C=O); HRMS (ESI): m/z calcd for C32H39NO6Na+: 556.2670; found: 556.2668. β Form; Rf 0.57 (EtOAc/hexane = 2/1); mp 138.2–138.4 °C; [α]26D +25.7 (c 1.1, CHCl3); 1H NMR (600 MHz, CDCl3): δ 0.89 (3H, t, J = 7.5 Hz, CH2CH3), 1.58 (2H, m, CH2CH3), 1.85 (3H, s, CH3), 3.38 (1H, q, J = 8.2 Hz, H-2), 3.42 (1H, dt, J = 6.8 Hz, J = 9.1 Hz, CHaHbCH2CH3), 3.59 (1H, m, H-5), 3.62 (1H, t, J = 8.6 Hz, H-4), 3.71 (1H, dd, J = 4.7 Hz, J = 10.4 Hz, Ha-6), 3.76 (1H, bd, J = 12.4 Hz, Hb-6), 3.81 (1H, td, J = 6.8 Hz, J= 9.5 Hz, CHaHbCH2CH3), 4.13 (1H, t, J = 8.9 Hz, H-3), 4.54–4.84 (6H, m, CH2Ph), 4.81 (1H, d, J = 8.4 Hz, H-1), 5.58 (1H, d, J = 7.4 Hz, NH), 7.19–7.34 (15H, m, Ph); 13C NMR (150 MHz, CDCl3): δ 10.4 (CH2CH3), 22.8 (CH2CH3), 23.5 (CH3), 57.1 (C-2), 69.0 (C-6), 71.1 (CH2CH2CH3), 73.4–74.5 (CH2Ph), 74.8 (C-5), 78.7 (C-4), 80.3 (C-3), 99.8 (C-1), 127.5–128.4 (Ph), 138.1-138.5 (Ph), 170.2 (C=O); HRMS (ESI): m/z calcd for C32H39NO6Na+: 556.2670; found: 556.2708.
Benzyl 2-Acetamido-3,4,6-tri-O-benzyl-2-deoxy-D-glucopyranoside (18)22-24
Using the same procedure as described above for 3, Yb(OTf)3 (125.1 mg, 0.2 mmol) was added to a solution of 1 (129.0 mg, 0.2 mmol), 5 (22.0 mg, 0.2 mmol), and BF3·OEt2 (60 μL, 0.006 mmol) in CH2Cl2 (3 mL) to give 18 (PTLC; EtOAc/hexane = 2/1, 86% yield, α form22; 52.1 mg, β form23, 24; 49.6 mg, α/β ratio = 51/49) as white crystals.
Allyl 2-Acetamido-3,4,6-tri-O-benzyl-2-deoxy-D-glucopyranoside (19)25,26
Using the same procedure as described above for 3, Yb(OTf)3 (126.4 mg, 0.2 mmol) was added to a solution of 1 (130.5 mg, 0.2 mmol), 6 (11.8 mg, 0.2 mmol), and BF3·OEt2 (61 μL, 0.006 mmol) in CH2Cl2 (3 mL) to give 19 (PTLC; EtOAc/hexane = 2/1, 102.1 mg, 94% yield, α/β ratio = 37/63) as white crystals. α25,26 And β form mixture; Rf 0.58 (EtOAc/hexane = 2/1); 1H NMR (600 MHz, CDCl3): δ 1.84 (3H, s, α-CH3), 1.85 (3H, s, β-CH3), 3.46 (1H, q, J = 8.4 Hz, β-H-2), 3.59 (1H, dt, J = 2.2 Hz, J = 6.9 Hz, β-H-5), 3.63–3.78 (7H, m, α-CH2=CHCH2, β-CH2=CHCH2, α-H-3, α-H-4, β-H-4), 3.80 (1H, dd, J = 2.2 Hz, J = 9.7 Hz , α-H-5), 3.94 (1H, dd, J = 6.1 Hz, J = 13.0 Hz, α-Ha-6), 4.06 (1H, dd, J = 6.7 Hz, J = 13.4 Hz, β-Ha-6), 4.10 (1H, t, J = 9.0 Hz, β-H-3), 4.14 (1H, dd, J = 5.2 Hz, J = 12.9 Hz, α-Hb-6), 4.28 (1H, td, J = 3.7 Hz, J = 9.8 Hz, α-H-2), 4.32 (1H, dd, J = 5.2 Hz, J = 12.9 Hz, β-Hb-6), 4.80 (1H, d, J =3.6 Hz, α-H-1), 4.50–4.86 (12H, m, CH2Ph), 4.85 (1H, d, J =7.6 Hz, β-H-1), 5.17 (2H, bdd, J = 10.5 Hz, J = 14.4 Hz, α-CHaHb=CHCH2, β-CHaHb=CHCH2), 5.24 (2H, td, J = 0.8 Hz, J = 10.4 Hz, J = 17.2 Hz, α-CHaHb=CHCH2, β-CHaHb=CHCH2), 5.31 (1H, d, J = 9.5 Hz, α-NH), 5.60 (1H, d, J = 7.7 Hz, β-NH), 5.86 (2H, m, α-CH2=CHCH2, β-CH2=CHCH2), 7.17–7.35 (30H, m, Ph); 13C NMR (150 MHz, CDCl3): δ 23.4 (α-CH3), 23.6 (β-CH3), 52.5 (α-C-2), 56.8 (β-C-2), 68.1 (α-C-6), 68.5 (α-CH2=CHCH2), 69.0 (β-C-6), 69.8 (β-CH2=CHCH2), 71.1 (α-C-5), 73.5–75.1 (CH2Ph), 74.8 (β-C-5), 78.4 (α-C-4), 78.5 (β-C-4), 80.2 (β-C-3), 80.4 (α-C-3), 96.9 (α-C-1), 99.0 (β-C-1), 117.3 (α-CH2=CHCH2), 117.6 (β-CH2=CHCH2), 127.6-128.5 (Ph), 133.7 (α-CH2=CHCH2), 134.1 (β-CH2=CHCH2), 138.0-138.5 (Ph), 169.7 (α-C=O), 170.3 (β-C=O); HRMS (ESI): m/z calcd for C32H37NO6Na+: 554.2513; found: 554.2509.
5-Benzyloxypenthyl 2-Acetamido-3,4,6-tri-O-benzyl-2-deoxy-D-glucopyranoside (20)
Using the above same procedure as described above for 3, Yb(OTf)3 (142.5 mg, 0.2 mmol) was added to a solution of 1 (147.2 mg, 0.3 mmol), 7 (44.6 mg, 0.2 mmol), and BF3·OEt2 (69 μL, 0.007 mmol) in CH2Cl2 (3 mL) to give 20 (PTLC; EtOAc/hexane = 2/1, 71% yield, α form; 41.8 mg, β form; 69.5 mg, α/β ratio = 38/62) as white crystals. α From; Rf 0.48 (EtOAc/hexane = 2/1); mp 84.0–84.8 °C; [α]26D +70.0 (c 0.64, CHCl3); 1H NMR (600 MHz, CDCl3): δ 1.41 (2H, m, BnOCH2CH2CH2), 1.57 (2H, q, J = 7.4 Hz, BnOCH2CH2), 1.62 (2H, q, J = 7.1 Hz, BnOCH2CH2CH2CH2), 1.81 (3H, s, CH3), 3.36 (1H, td, J = 6.5 Hz, J = 9.8 Hz, BnOCH2CH2CH2CH2CHaHb), 3.46 (2H, t, J = 6.5 Hz, BnOCH2), 3.66 (1H, dt, J = 6.4 Hz, J = 9.8 Hz, BnOCH2CH2CH2CH2CHaHb), 3.67 (1H, bd, J = 10.3 Hz, Ha-6), 3.69 (1H, t, J = 10.0 Hz, H-3), 3.74 (1H, t, J = 9.0 Hz, H-4), 3.76 (1H, bd, J = 9.6 Hz, Hb-6), 3.77 (1H, m, H-5), 4.26 (1H, dt, J = 3.7 Hz, J = 9.9 Hz, H-2), 4.49–4.84 (8H, m, CH2Ph), 4.76 (1H, d, J = 3.8 Hz, H-1), 5.31 (1H, d, J = 9.5 Hz, NH), 7.16–7.33 (20H, m, Ph); 13C NMR (150 MHz, CDCl3): δ 22.9 (BnOCH2CH2CH2), 23.4 (CH3), 29.1 (BnOCH2CH2), 29.4 (BnOCH2CH2CH2CH2), 52.5 (C-2), 67.7 (BnOCH2), 68.6 (C-6), 70.1 (BnOCH2CH2CH2CH2CH2), 70.9 (C-5), 72.9–75.0 (CH2Ph), 78.4 (C-4), 80.5 (C-3), 97.6 (C-1), 127.5– 128.5 (Ph), 138.0-138.5 (Ph), 169.6 (C=O); HRMS (ESI): m/z calcd for C41H49NO7Na+: 690.3401; found: 690.3380. β Form; Rf 0.65 (EtOAc/hexane = 2/1); mp 83.2–85.5 °C; [α]26D +10.1 (c 1.0, CHCl3); 1H NMR (600 MHz, CDCl3): δ 1.41 (2H, q, J = 7.7 Hz, BnOCH2CH2CH2), 1.57 (2H, q, J = 7.3 Hz, BnOCH2CH2CH2CH2), 1.61 (2H, q, J = 7.1 Hz, BnOCH2CH2), 1.81 (3H, s, CH3), 3.37 (1H, dt, J = 7.9 Hz, J = 9.6 Hz, H-2), 3.44 (2H, t, J = 6.6 Hz, BnOCH2), 3.43-3.47 (1H, m, BnOCH2CH2CH2CH2CHaHb), 3.57 (1H, ddd, J = 2.3 Hz, J = 4.6 Hz, J = 9.3 Hz, H-5), 3.60 (1H, t, J = 8.8 Hz, H-4), 3.70 (1H, dd, J = 4.5 Hz, J = 10.7 Hz, Ha-6), 3.75 (1H, dd, J = 2.4 Hz, J = 10.7 Hz, Hb-6), 3.86 (1H, dt, J = 6.4 Hz, J = 9.6 Hz, BnOCH2CH2CH2CH2CHaHb), 4.10 (1H, dd, J = 8.3 Hz, J = 9.6 Hz, H-3), 4.79 (1H, d, J = 7.7 Hz, H-1), 5.53 (1H, d, J = 7.9 Hz, NH), 4.47–4.82 (8H, m, CH2Ph), 7.19–7.34 (20H, m, Ph); 13C NMR (150 MHz, CDCl3): δ 22.7 (BnOCH2CH2CH2), 23.5 (CH3), 29.3 (BnOCH2CH2), 29.5 (BnOCH2CH2CH2CH2), 57.1 (C-2), 69.1 (C-6), 69.4 (BnOCH2CH2CH2CH2CH2), 70.3 (BnOCH2), 72.9–74.6 (CH2Ph), 74.8 (C-5), 78.7 (C-4), 80.4 (C-3), 99.8 (C-1), 127.5–128.5 (Ph), 138.1–138.6 (Ph), 170.3 (C=O); HRMS (ESI): m/z calcd for C41H49NO7Na+: 690.3401; found: 690.3389.
3-β-Cholestanyl 2-Acetamido-3,4,6-tri-O-benzyl-2-deoxy-D-glucopyranoside (21)
Using the same procedure as described above for 3, Yb(OTf)3 (129.0 mg, 0.2 mmol) was added to a solution of 1 (133.2 mg, 0.2 mmol), 8 (80.8 mg, 0.1 mmol), and BF3·OEt2 (62 μL, 0.006 mmol) in CH2Cl2 (3 mL) to give 21 (PTLC; EtOAc/hexane = 2/1, 70% yield, α form; 28.8 mg, β form; 96.4 mg, α/β ratio = 23/77) as white crystals. α Form; Rf 0.28 (EtOAc/hexane = 2/1); mp 164.4–166.0 °C; [α]27D +82.6 (c 1.0, CHCl3); 1H NMR (600 MHz, CDCl3): δ 1.86 (3H, s, CH3), 0.57-1.97 (47H, m, 3-β-cholestanyl), 3.50 (1H, dt, J = 5.4 Hz, J= 10.9 Hz, CH), 3.68 (1H, t, J = 9.5 Hz, H-3), 3.67 (1H, dd, J = 1.9 Hz, J = 10.9 Hz, Ha-6), 3.72 (1H, t, J = 9.3 Hz, H-4), 3.76 (1H, dd, J = 4.3 Hz, J = 8.8 Hz, Hb-6), 3.89 (1H, ddd, J = 1.9 Hz, J = 4.1 Hz, J = 9.4 Hz, H-5), 4.24 (1H, dt, J = 3.8 Hz, J = 9.8 Hz, H-2), 4.50–4.85 (6H, m, CH2Ph), 4.91 (1H, d, J = 3.8 Hz, H-1), 5.32 (1H, d, J = 9.3 Hz, NH), 7.16–7.35 (15H, m, Ph); 13C NMR (150 MHz, CDCl3): δ 23.5 (CH3), 12.1–56.4 (3-β-cholestanyl), 52.5 (C-2), 68.7 (C-6), 70.9 (C-5), 73.4–75.0 (CH2Ph), 77.0 (CH), 78.5 (C-4), 80.8 (C-3), 96.2 (C-1), 169.6 (C=O), 127.6–128.5 (Ph), 138.1-138.6 (Ph); HRMS (ESI): m/z calcd for C56H79NO6Na+: 884.5800; found: 884.5843. β Form; Rf 0.41 (EtOAc/hexane = 2/1); mp 164.0–165.3 °C; [α]26D +28.8 (c 1.0, CHCl3); 1H NMR (600 MHz, CDCl3): δ 1.84 (3H, s, CH3), 0.63-1.97 (47H, m, 3-β-cholestanyl), 3.19 (1H, td, J = 7.9 Hz, J = 9.6 Hz, H-2), 3.54–3.59 (3H, m, H-4, H-5, CH), 3.68 (1H, dd, J = 4.1 Hz, J = 10.7 Hz, Ha-6), 3.75 (1H, dd, J = 1.0 Hz, J = 10.7 Hz, Hb-6), 4.25 (1H, dd, J = 9.6 Hz, J = 8.3 Hz, H-3), 4.54–4.83 (6H, m, CH2Ph), 5.00 (1H, d, J = 7.9 Hz, H-1), 5.57 (1H, d, J = 7.2 Hz, NH), 7.20-7.34 (15H, m, Ph); 13C NMR (150 MHz, CDCl3): δ 23.6 (CH3), 12.1–56.5 (3-β-cholestanyl), 58.2 (C-2), 69.2 (C-6), 73.4–74.7 (CH2Ph), 74.7 (C-5), 78.8 (C-4), 79.1 (CH), 80.4 (C-3), 98.1 (C-1), 127.5-128.4 (Ph), 138.1-138.6 (Ph), 170.3 (C=O); HRMS (ESI): m/z calcd for C56H79NO6Na+: 884.5800; found: 884.5833.
Cyclohexyl 2-Acetamido-3,4,6-tri-O-benzyl-2-deoxy-D-glucopyranoside (22)27
Using the above same procedure as described above for 3, Yb(OTf)3 (130.0 mg, 0.2 mmol) was added to a solution of 1 (134.2 mg, 0.2 mmol), 9 (21 mg, 0.3 mmol), and BF3·OEt2 (63 μL, 0.006 mmol) in CH2Cl2 (3 mL) to give 21 (PTLC; EtOAc/hexane = 2/1, 81.7 mg, 68% yield, α/β ratio = 53/47) as white crystals. α And β27 from mixture; Rf 0.72 (EtOAc/hexane = 2/1); 1H NMR (600 MHz, CDCl3): δ 1.83 (3H, s, α-CH3), 1.84 (3H, s, β-CH3), 1.17–1.93 (20H, m, cyclohexyl), 3.21 (1H, dt, J = 7.9 Hz, J = 9.8 Hz, β-H-2), 3.55–3.57 (3H, m, α-CH, β-H-5, β-H-4), 3.59–3.63 (2H, m, β-CH, α-H-3), 3.66–3.78 (5H, m, α-H-6, β-H-6, α-H-4), 3.89 (1H, ddd, J = 1.7 Hz, J = 4.0 Hz, J = 9.6 Hz, α-H-5), 4.23–4.27 (2H, m, α-H-2, β-H-3), 4.50–4.86 (12H, m, CH2Ph), 4.92 (1H, d, J = 3.8 Hz, α-H-1), 5.00 (1H, d, J = 8.1 Hz, β-H-1), 5.26 (1H, d, J = 9.3 Hz, α-NH), 5.69 (1H, d, J = 7.6 Hz, β-NH), 7.17–7.35 (30H, m, Ph); 13C NMR (150 MHz, CDCl3): δ 23.4 (α-CH3), 23.6 (β-CH3), 23.4-25.6 (cyclohexyl), 52.6 (α-C-2), 58.2 (β-C-2), 68.7 (α-C-6), 69.2 (β-C-6), 71.0 (α-C-5), 73.4-74.8 (CH2Ph), 74.7 (β-C-5), 75.1 (α-CH), 77.3 (β-CH), 78.5 (α-C-4), 79.0 (β-C-4), 80.4 (β-C-3), 80.5 (α-C-3), 95.9 (α-C-1), 98.0 (β-C-1), 127.5-128.5 (Ph), 138.1-138.6 (Ph), 169.6 (α-C=O), 170.3 (β-C=O); HRMS (ESI): m/z calcd for C35H43NO6Na+: 596.2983; found: 596.2981.
2-Adamantyl 2-Acetamido-3,4,6-tri-O-benzyl-2-deoxy-D-glucopyranoside (23)
Using the same procedure as described above for 3, Yb(OTf)3 (144.2 mg, 0.2 mmol) was added to a solution of 1 (148.9 mg, 0.3 mmol), 10 (35.4 mg, 0.2 mmol), and BF3·OEt2 (70 μL, 0.007 mmol) in CH2Cl2 (3 mL) to give 23 (PTLC; EtOAc/hexane = 2/1, 71% yield, α form; 31.5 mg, β form; 71.4 mg, α/β ratio = 31/69) as white crystals. α Form; Rf 0.63 (EtOAc/hexane = 2/1); mp 121.0–122.8 °C; [α]26D +82.6 (c 0.55, CHCl3); 1H NMR (600 MHz, CDCl3): δ 1.79 (3H, s, CH3), 1.50–2.17 (14H, m, 2-adamantyl), 3.67 (1H, dd, J = 1.6 Hz, J = 10.7 Hz, Ha-6), 3.72-3.80 (4H, m, H-3, H-4, Hb-6, CH), 3.88 (1H, bd, J = 9.8 Hz, H-5), 4.20 (1H, dt, J = 3.5 Hz, J = 9.7 Hz, H-2), 4.50–4.87 (6H, m, CH2Ph), 4.94 (1H, d, J = 3.6 Hz, H-1), 5.12 (1H, d, J = 9.1 Hz, NH), 7.20–7.36 (15H, m, Ph); 13C NMR (150 MHz, CDCl3): δ 23.4 (CH3), 27.1–37.4 (2-adamantyl), 52.7 (C-2), 68.7 (C-6), 71.3 (C-5), 73.4–75.2 (CH2Ph), 78.5 (C-4), 79.5 (CH), 79.8 (C-3), 95.8 (C-1), 127.6-128.5 (Ph), 138.0-138.7 (Ph), 169.5 (C=O); HRMS (ESI): m/z calcd for C39H47NO6Na+: 648.3296; found: 648.3252. β Form; Rf 0.78 (EtOAc/hexane = 2/1); mp 119.8– 121.8 °C; [α]26D +19.4 (c 1.1, CHCl3); 1H NMR (600 MHz, CDCl3): δ 1.83 (3H, s, CH3), 1.44–2.17 (14H, m, 2-adamantyl), 3.36 (1H, dt, J = 8.4 Hz, J= 9.7 Hz, H-2), 3.60 (1H, t, J = 8.8 Hz, H-4), 3.68 (1H, dd, J = 4.6 Hz, J = 10.7 Hz, Ha-6), 3.76 (1H, dd, J = 1.9 Hz, J = 10.7 Hz, Hb-6), 3.87 (1H, s, CH), 3.88 (1H, m, H-5), 4.19 (1H, t, J = 9.0 Hz, H-3), 4.55–4.84 (6H, m, CH2Ph), 4.92 (1H, d, J = 7.9 Hz, H-1), 5.56 (1H, d, J = 7.6 Hz, NH), 7.21–7.35 (15H, m, Ph); 13C NMR (150 MHz, CDCl3): δ 23.6 (CH3), 27.1–37.6 (2-adamantyl), 57.8 (C-2), 69.1 (C-6), 73.4–74.6 (CH2Ph), 74.8 (C-5), 79.0 (C-4), 80.4 (C-3), 80.7 (CH), 97.7 (C-1), 127.5-128.5 (Ph), 138.2-138.7 (Ph), 170.2 (C=O); HRMS (ESI): m/z calcd for C39H47NO6Na+: 648.3296; found: 648.3255.
Methyl 6-O-(2’-Acetamido-3’,4’,6’-tri-O-benzyl-2’-deoxy-D-glucopyranosyl)-2,3,4-tri-O-benzyl-α- D-glucopyranoside (24)24
Using the same procedure as described above for 3, Yb(OTf)3 (128.2 mg, 0.2 mmol) was added to a solution of 1 (132.4 mg, 0.2 mmol), 11 (96.0 mg, 0.2 mmol), and BF3·OEt2 (62 μL, 0.006 mmol) in CH2Cl2 (3 mL) to give 24 (PTLC; EtOAc/hexane = 2/1, 37% yield, α form; 24.9 mg, β form24; 45.7 mg, α/β ratio = 35/65) as white crystals. α Form; Rf 0.23 (EtOAc/hexane = 2/1); mp 164.0–165.6 °C; [α]26D +86.6 (c 1.1, CHCl3); 1H NMR (600 MHz, CDCl3): δ 1.70 (3H, s, CH3), 3.33 (3H, s, OCH3), 3.37 (1H, t, J = 9.5 Hz, H-4’), 3.42 (1H, dd, J = 3.6 Hz, J = 9.6 Hz, H-3), 3.56-3.59 (2H, m, Ha-6’ Ha-6), 3.61 (1H, dd, J = 8.3 Hz, J = 10.4 Hz, H-3’), 3.67 (1H, dd, J = 3.4 Hz, J = 10.7 Hz, Hb-6’), 3.69–3.74 (3H, m, H-5, H-5’, H-4’), 3.85 (1H, dd, J = 4.6 Hz, J = 11.3 Hz, Hb-6), 3.98 (1H, t, J = 9.2 Hz, H-2), 4.19 (1H, dt, J = 3.6 Hz, J = 9.9 Hz, H-2’), 4.45–5.02 (12H, m, CH2Ph), 4.52 (1H, d, J = 2.9 Hz, H-1), 4.80 (1H, d, J = 4.1 Hz, H-1’), 5.20 (1H, d, J = 9.3 Hz, NH), 7.17–7.37 (30H, m, Ph); 13C NMR (150 MHz, CDCl3): δ 23.4 (CH3), 52.6 (C-2’), 55.2 (OCH3), 66.7 (C-6), 68.4 (C-6’), 69.7 (C-5’), 71.3 (C-5), 72.9–74.6 (CH2Ph), 77.7 (C-4), 78.3 (C-4’), 79.7 (C-3’), 80.0 (C-3), 82.0 (C-2), 97.9 (C-1), 98.5 (C-1’), 127.5–128.5 (Ph), 138.1–138.6 (Ph), 169.5 (C=O); HRMS (ESI): m/z calcd for C57H63NO11Na+: 960.4293; found: 960.4283. β Form; Rf 0.65 (EtOAc/hexane = 2/1); mp 194.5–195.8 °C; [α]26D +25.9 (c 2.1, CHCl3); 1H NMR (600 MHz, CDCl3): δ 1.70 (3H, s, CH3), 3.34 (3H, s, OCH3), 3.49–3.51 (1H, m, H-3’), 3.52 (1H, t, J = 8.9 Hz, H-4), 3.59 (2H, m, H-4’, H-5’), 3.68 (1H, dd, J = 3.6 Hz, J = 11.2 Hz, Ha-6), 3.70-3.74 (3H, m, H-5’, H-6’), 3.97 (1H, t, J = 9.3 Hz, H-2), 4.09 (1H, t, J = 9.2 Hz, H-3), 4.09–4.11 (1H, m, Hb-6), 4.49–4.98 (12H, m, CH2Ph), 4.58 (1H, d, J = 3.3 Hz, H-1), 4.83 (1H, d, J = 7.2 Hz, H-1’), 5.44 (1H, d, J = 7.7 Hz, NH), 7.19–7.34 (30H, m, Ph); 13C NMR (150 MHz, CDCl3): δ 23.5 (CH3), 52.1 (OCH3), 56.7 (C-2’), 67.4 (C-6), 69.1 (C-6’), 69.6 (C-5’), 73.3-75.7 (CH2Ph), 74.9 (C-5), 77.6 (C-4), 78.6 (C-4’), 79.7 (C-3’), 80.1 (C-3), 82.0 (C-2), 98.0 (C-1), 99.8 (C-1’), 127.5–128.4 (Ph), 138.0–138.8 (Ph), 170.1 (C=O); HRMS (ESI): m/z calcd for C57H63NO11Na+: 960.4293; found: 961.4316.
Phenyl 2-Acetamido-3,4,6-tri-O-benzyl-2-deoxy-α-D-glucopyranoside (25)
Using the same procedure as described above for 3, Yb(OTf)3 (124 mg, 0.2 mmol) was added to a solution of 1 (141 mg, 0.3 mmol), 12 (20.6 mg, 0.2 mmol), and BF3·OEt2 (66 μL, 0.007 mmol) in CH2Cl2 (3 mL) to give 25 (PTLC; EtOAc/hexane = 2/1, 83.4 mg, 67% yield) as white crystals. Rf 0.47 (EtOAc/hexane = 2/1); mp 161.1–161.8 °C; [α]28D +148.8 (c 1.0, CHCl3); 1H NMR (600 MHz, CDCl3): δ 1.75 (3H, s, CH3), 3.44 (1H, dt, J = 3.4 Hz, J = 9.6 Hz, H-2), 3.54 (1H, d, J = 11.0 Hz, H a-6), 3.68 (1H, dd, J = 2.1 Hz, J = 11.0 Hz, H b-6), 3.78-3.90 (3H, m, H-3, H-4, H-5), 4.37-4.85 (6H, m, CH2Ph), 5.28 (1H, d, J = 8.9 Hz, NH), 5.47 (1H, d, J = 4.1 Hz, H-1), 6.80–7.40 (20H, m, Ph); 13C NMR (150 MHz, CDCl3): δ 23.3 (CH3), 52.5 (C-2), 68.3 (C-6), 71.6 (C-5), 73.4–75.1 (CH2Ph), 78.2 (C-4), 79.8 (C-3), 96.3 (C-1), 116.6 (Ph), 122.6 (Ph), 129.6 (Ph), 156.1 (Ph), 169.9 (C=O), 127.6–128.5 (Ph), 137.9–138.4 (Ph); HRMS (ESI): m/z calcd for C35H37NO6Na+: 590.2513; found: 590.2514.
4-O-Methylphenyl 2-Acetamido-3,4,6-tri-O-benzyl-2-deoxy-α-D-glucopyranoside (26)
Using the same procedure as described above for 3, Yb(OTf)3 (124.2 mg, 0.2 mmol) was added to a solution of 1 (128.0 mg, 0.2 mmol), 13 (25 mg, 0.2 mmol), and BF3·OEt2 (60 μL, 0.006 mmol) in CH2Cl2 (3 mL) to give 26 (PTLC; EtOAc/hexane = 2/1, 100.6 mg, 84% yield) as white crystals. Rf 0.58 (EtOAc/hexane = 2/1); mp 189.2–189.8 °C; [α]25D +172.1 (c 1.0, CHCl3); 1H NMR (600 MHz, CDCl3): δ 1.77 (3H, s, CH3), 3.56 (1H, dd, J = 2.1 Hz, J = 11.0 Hz, Ha-6), 3.68 (1H, s, PhCH3), 3.69 (1H, bd, J = 11.0 Hz, Hb-6), 3.78 (1H, t, J = 8.9 Hz, H-4), 3.82 (1H, t, J = 9.3 Hz, H-3), 3.87 (1H, ddd, J = 9.6 Hz, J = 3.4 Hz, J = 1.4 Hz, H-5), 4.32 (1H, dt, J = 3.4 Hz, J = 9.6 Hz, H-2), 4.38–4.84 (6H, m, CH2Ph), 5.28 (1H, d, J = 8.9 Hz, NH), 5.35 (1H, d, J = 3.4 Hz, H-1), 6.72 (2H, d, J = 8.9 Hz, Ph), 6.87 (2H, d, J = 8.9 Hz, Ph), 7.17–7.31 (15H, m, Ph); 13C NMR (150 MHz, CDCl3): δ 23.4 (CH3), 52.6 (C-2), 55.6 (PhCH3), 68.4 (C-6), 71.5 (C-5), 73.4–75.1 (CH2Ph), 78.3 (C-4), 79.9 (C-3), 97.2 (C-1), 114.6 (Ph), 118.0 (Ph), 128.5–127.6 (Ph), 150.1 (Ph), 155.2 (Ph), 169.8 (C=O), 138.0-138.4 (Ph); HRMS (ESI): m/z calcd for C36H39NO7Na+: 620.2619; found: 620.2616.
4-Benzoyloxyphenyl 2-Acetamido-3,4,6-tri-O-benzyl-2-deoxy-α-D-glucopyranoside (27)
Using the same procedure as described above for 3, Yb(OTf)3 (113.8 mg, 0.2 mmol) was added to a solution of 1 (117.5 mg, 0.2 mmol), 14 (39.3 mg, 0.2 mmol), and BF3·OEt2 (55 μL, 0.006 mmol) in CH2Cl2 (3 mL) to give 27 (PTLC; EtOAc/hexane = 2/1, 70.2 mg, 57% yield) as white crystals. Rf 0.81 (EtOAc/hexane = 2/1); mp 190.5–191.7 °C; [α]27D +160.8 (c 1.0, CHCl3); 1H NMR (600 MHz, CDCl3): δ 1.84 (3H, s, CH3), 3.64 (1H, d, J = 10.8 Hz, Ha-6), 3.78 (1H, dd, J = 1.5 Hz, J = 11.0 Hz, Hb-6), 3.90–3.92 (3H, m, H-3, H-4, H-5), 4.42 (1H, ddd, J = 3.3 Hz, J = 6.7 Hz, J = 12.5 Hz, H-2), 4.46–4.93 (6H, m, CH2Ph), 5.30 (1H, d, J = 9.1 Hz, NH), 5.54 (1H, d, J = 3.4 Hz, H-1), 7.07 (2H, d, J = 9.1 Hz, Ph), 7.12 (2H, d, J = 9.1 Hz, Ph), 7.20–7.40 (15H, m, Ph), 7.51 (2H, t, J = 7.8 Hz, Ph), 7.63 (1H, dt, J = 1.3 Hz, J = 7.5 Hz, Ph), 8.19 (2H, dd, J = 1.3 Hz, J = 8.3 Hz, Ph); 13C NMR (150 MHz, CDCl3): δ 23.4 (CH3), 52.5 (C-2), 68.2 (C-6), 71.8 (C-5), 73.5-75.1 (CH2Ph), 78.2 (C-4), 79.8 (C-3), 96.8 (C-1), 117.4 (Ph), 122.7 (Ph), 127.7-128.6 (Ph), 129.5 (Ph), 130.1-130.2 (Ph), 133.6 (Ph), 137.9–138.4 (Ph), 145.9 (Ph), 153.9 (Ph), 165.4 (C=O), 169.9 (C=O(CH3)); HRMS (ESI): m/z calcd for C42H39NO8Na+: 710.2724; found: 710.2734.
4-O-(2-Acetamido-3,4,6-tri-O-benzyl-2-deoxy-α-D-glucopyranosyl)-benzoic acid benzyl ester (28)
Using the same procedure as described above for 3, Yb(OTf)3 (80.0 mg, 0.13 mmol) was added to a solution of 1 (82.6 mg, 0.15 mmol), 15 (29.4 mg, 0.13 mmol), and BF3·OEt2 (39 μL, 0.004 mmol) in CH2Cl2 (3 mL) to give 28 (PTLC; EtOAc/hexane = 2/1, 19.2 mg, 46% yield) as white crystals. Rf 0.57 (EtOAc/hexane = 2/1); mp 147.2–148.1 °C; [α]25D +163.7 (c 1.0, CHCl3); 1H NMR (600 MHz, CDCl3): δ 1.74 (3H, s, CH3), 3.49 (1H, d, J = 9.8 Hz, H a-6), 3.66 (1H, dd, J = 3.5 Hz, J = 10.9 Hz, H b-6 ), 3.71 (1H, d, J = 8.1 Hz, H-5), 3.82 (2H, m, H-3, H-4), 4.33 (1H, dd, J = 6.7 Hz, J = 8.9 Hz, H-2), 4.35–4.85 (6H, m, CH2Ph), 5.15 (1H, d, J = 8.8 Hz, NH), 5.25 (2H, d, J = 2.6 Hz, CH2Ph), 5.56 (1H, d, J = 3.3 Hz, H-1), 6.97 (2H, d, J = 8.8 Hz, Ph), 7.10–7.35 (20H, m, Ph), 7.93 (2H, d, J = 8.6 Hz, Ph); 13C NMR (150 MHz, CDCl3): δ 23.2 (CH3), 52.4 (C-2), 66.5 (CH2Ph), 68.1 (C-6), 71.8 (C-5), 73.4–75.1 (CH2Ph ), 78.0 (C-4), 79.4 (C-3), 95.9 (C-1), 116.0 (Ph), 124.3 (Ph), 131.7 (Ph), 136.1 (Ph), 159.7 (Ph), 165.8 (C=O), 170.0 (C=O(CH3)), 127.7-128.6 (Ph), 137.7-138.2 (Ph); HRMS (ESI): m/z calcd for C43H43NO8Na+: 724.2881; found: 724.2883.
4-O-(2-Acetamido-3,4,6-tri-O-benzyl-2-deoxy-α-D-glucopyranosyl)-N-benzyloxycarbonyl-L-tyrosine benzyl ester (29)
Using the same procedure as described above for 3, Yb(OTf)3 (76.0 mg, 0.1 mmol) was added to a solution of 1 (78.4 mg, 0.1 mmol), 16 (49.7 mg, 0.1 mmol), and BF3·OEt2 (37 μL, 0.004 mmol) in CH2Cl2 (3 mL) to give 29 (PTLC; EtOAc/hexane = 2/1, 64.8 mg, 60% yield) as white crystals. Rf 0.70 (EtOAc/hexane = 2/1); mp 169.9–170.1 °C; [α]25D +105.5 (c 1.1, CHCl3); 1H NMR (600 MHz, CDCl3): δ 1.74 (3H, s, CH3), 2.96 (2H, m, PhCH2CH), 3.50 (1H, d, J = 9.8 Hz, Ha-6), 3.67 (1H, dd, J = 3.2 Hz, J = 10.9 Hz, Hb-6), 3.76 (1H, bd, J = 6.9 Hz, H-5), 3.81–3.82 (2H, m, H-3, H-4), 4.33 (1H, td, J = 4.0 Hz, J = 9.3 Hz, H-2), 4.35–4.84 (6H, m, CH2Ph), 4.57 (1H, dd, J = 5.5 Hz, J = 13.2 Hz, CH), 5.00 (2H, s, NHC(O)OCH2Ph), 5.02 (1H, d, J = 12.5 Hz, C(O)OCHaHbPh), 5.07 (1H, d, J = 12.0 Hz, C(O)OCHaHbPh), 5.16 (1H, d, J = 8.1 Hz, NH), 5.22 (1H, d, J = 9.1 Hz, NH), 5.41 (1H, d, J = 3.4 Hz, H-1), 6.76 (1H, d, J = 8.3 Hz, Ph), 6.80 (1H, d, J = 8.4 Hz, Ph), 7.10–7.31 (25H, m, Ph); 13C NMR (150 MHz, CDCl3): δ 23.3 (CH3), 37.2 (PhCH2CH), 52.3 (C-2), 54.8 (CH), 66.9 (NHC(O)OCH2Ph), 67.2 (C(O)OCH2Ph), 68.1 (C-6), 71.6 (C-5), 73.3–75.0 (CH2Ph), 78.0 (C-4), 79.7 (C-3), 96.2 (C-1), 116.5 (Ph), 127.6-128.6 (Ph), 129.6 (Ph), 130.4 (Ph), 135.0-136.1 (Ph), 137.8–138.3 (Ph), 155.1 (Ph), 155.5 (Ph), 169.8 (C=O(CH3)), 172.2 (C=O); HRMS (ESI): m/z calcd for C53H54NO10Na+: 901.3671; found: 901.3675.
4-Hydroxyphenyl 2-acetamido-3,4,6-tri-O-benzyl-2-deoxy-α-D-glucopyranoside (35) and 4-tert-butoxyphenyl 2-acetamido-3,4,6-tri-O-benzyl-2-deoxy-α-D-glucopyranoside (36)
Yb(OTf)3 (126.9 mg, 0.20 mmol) was added to a solution of 1 (133.8 mg, 0.25 mmol), 34 (35.0 mg, 0.21 mmol), and BF3·OEt2 (0.8 μL, 0.006 mmol) in CH2Cl2 (2 mL) at 0 °C. The resulting mixture was stirred for 1 h at room temperature and then quenched by the addition of sat. aq. NaHCO3 solution (5 mL). The reaction mixture was then extracted with CH2Cl2, and the organic layer was rinsed with water and sat. aq. NaCl solution. After the organic layer was dried over Na2SO4, the solvent was evaporated under reduced pressure. The crude products were purified via preparative silica gel TLC (EtOAc/hexane = 1/1) to give 35 (80.3 mg, 66%) and 36 (10.7 mg, 8%) as white crystals. Compound 35; Rf 0.12 (EtOAc/hexane = 1/1); mp 207.2–209.2 °C; [α]23D +38 (c 0.9, CHCl3); 1H NMR (600 MHz, CDCl3): δ: 1.81 (3H, s, CH3), 3.63 (1H, d, J = 10.7 Hz, Ha-6), 3.76 (1H, dd, J = 3.9 Hz, J = 9.5 Hz, Hb-6), 3.84–3.94 (3H, m, H-3, H-4, H-5), 4.33 (1H, dt, J = 3.9 Hz, J = 9.5 Hz, H-2), 4.44 (1H, d, J = 12.2 Hz, CH2Ph), 4.57 (1H, d, J = 10.7 Hz, CH2Ph), 4.60 (1H, d, J = 12.2 Hz, CH2Ph), 4.68 (1H, d, J = 11.7 Hz, CH2Ph), 4.83 (1H, d, J = 10.7 Hz, CH2Ph), 4.90 (1H, d, J = 11.7 Hz, CH2Ph), 5.30 (1H, d, J = 8.5 Hz, NH), 5.40 (1H, d, J = 3.7 Hz, H-1), 6.68 (2H, d, J = 9.1 Hz, Ph), 6.82 (2H, d, J = 8.8 Hz, Ph), 7.20–7.37 (15H, m, Ph); 13C-NMR (150 MHz, CDCl3) δ: 23.4 (CH3), 52.8 (C-2), 68.3 (C-6), 71.5 (C-5), 73.4 (CH2Ph), 74.9 (CH2Ph), 75.1 (CH2Ph), 78.3 (C-4), 79.6 (C-3), 97.1 (C-1), 116.1 (Ph), 118.1 (Ph), 127.6-151.5 (Ph), 170.2 (C=O); Anal. Calcd for C35H37NO7: C, 72.02; H, 6.39; N, 2.40. Found: C, 71.80; H, 6.40; N, 2.36. Compound 36; Rf 0.36 (EtOAc/hexane = 1/1); mp 135.2–137.2 °C; [α]23D +134 (c 0.3, CHCl3); 1H NMR (600 MHz, CDCl3): δ 1.29 (9H, s, CH3), 1.83 (3H, s, CH3), 3.63 (1H, dd, J = 1.7 Hz, J = 11.0 Hz, Ha-6), 3.76 (1H, dd, J = 3.4 Hz, J = 11.0 Hz, Hb-6), 3.86-3–89 (2H, m, H-3, H-4), 3.91-3.93 (1H, m, H-5), 4.38 (1H, dt, J = 3.7 Hz, J = 9.5 Hz, H-2), 4.46 (1H, d, J = 12.2 Hz, CH2Ph), 4.56 (1H, d, J = 10.8 Hz, CH2Ph), 4.62 (1H, d, J = 11.9 Hz, CH2Ph), 4.70 (1H, d, J = 11.7 Hz, CH2Ph), 4.83 (1H, d, J = 10.8 Hz, CH2Ph), 4.90 (1H, d, J = 11.7 Hz, CH2Ph), 5.28 (1H, d, J = 9.0 Hz, NH), 5.44 (1H, d, J = 3.5 Hz, H-1), 6.89 (4H, m, Ph), 7.18–7.39 (15H, m, Ph); 13C NMR (150 MHz, CDCl3): δ 23.4 (Ac), 28.7 (Me x 3), 52.4 (C-2), 68.3 (C-6), 71.6 (C-5), 73.4 (CH2Ph), 74.8 (CH2Ph), 75.1 (CH2Ph), 78.2 (C-4), 78.4 (C), 79.8 (C-3), 97.0 (C-1), 117.1 (Ph), 125.4 (Ph), 127.7–128.6 (Ph), 137.8 (Ph), 137.9 (Ph), 138.3 (Ph), 150.4 (Ph), 152.2 (Ph), 169.8 (C=O); Anal. Calcd for C39H45NO7·2.5H2O: C, 68.40; H, 7.36; N, 2.05. Found: C, 68.69; H, 7.09; N, 1.99.
4-Hydroxyphenyl 2-acetamido-2-deoxy-α-D-glucopyranoside (31)
Palladium hydroxide (26.4 mg, 0.1 mmol) was added to a solution of 35 (54.6 mg, 0.1 mmol) in THF/MeOH (5 mL/5 mL). Hydrogen was then bubbled through the solution for 1 h. After the solvent was filtered and evaporated under reduced pressure, the crude product was purified via flash column chromatography on silica gel (CHCl3/MeOH = 8/1) to afford 31 (27.3 mg, 93%) as white crystals. Rf 0.10 (CHCl3/MeOH = 8/1); mp 78.8–80.8 °C; [α]23D +150 (c 1.1, MeOH); 1H NMR (600 MHz, D2O) δ: 1.91 (3H, s, CH3), 3.44 (1H, t, J = 9.6 Hz, H-4), 3.65–3.67 (2H, m, H-6), 3.69–3.71 (1H, m, H-5), 3.80 (1H, t, J = 10.3 Hz, H-3), 3.91 (1H, dd, J = 3.4 Hz, J = 11.0 Hz, H-2), 5.27 (1H, d, J = 2.7 Hz, H-1), 6.70 (2H, d, J = 9.0 Hz, Ph), 6.87 (2H, d, J = 9.0 Hz, Ph); 13C NMR (150 MHz, D2O) δ: 22.6 (CH3), 54.3 (C-2), 61.0 (C-6), 70.5 (C-4), 71.6 (C-3), 73.2 (C-5), 97.7 (C-1), 116.8 (Ph), 119.5 (Ph), 150.3 (Ph), 151.9 (Ph), 175.0 (C=O); Anal. Calcd for C14H19NO7: C, 53.67; H, 6.11; N, 4.47. Found: C, 53.45; H, 6.44; N, 4.23.
References
1. S. Kobayashi, M. Sugiura, H. Kitagawa, and W. W.-L. Lam, Chem. Rev., 2002, 102, 2227. CrossRef
2. (a) T. Yamanoi, Y. Iwai, and T. Inazu, Heterocycles, 2000, 53, 1263; CrossRef (b) T. Yamanoi and I. Yamazaki, Tetrahedron Lett., 2001, 42, 4009. CrossRef
3. K. Ikeda, Y. Torisawa, T. Nishi, J. Minamikawa, K. Tanaka, and M. Sato, Bioorg. Med. Chem., 2003, 11, 3073.
4. T. Mukaiyama and N. Shimomura, Chem. Lett., 1993, 781. CrossRef
5. S. Hosono, W.-S. Kim, H. Sasai, and M. Shibasaki, J. Org. Chem., 1995, 60, 4. Other references cited therein. CrossRef
6. (a) T. Yamanoi, Y. Iwai, and T. Inazu, J. Carbohydr. Chem., 1998, 17, 819; CrossRef (b) It was found that Yb(OTf)3 was basically ineffective for the less reactive glycosyl acetates such as 2,3,4,6-tetra-O- benzyl-D-glucopyranosyl acetate. J. Inanaga, Y. Yokoyama, and T. Hashimoto, Tetrahedron Lett., 1993, 34, 2791. CrossRef
7. R. Harrison and H. G. Fletcher, J. Org. Chem., 1965, 30, 2317. CrossRef
8. T. Yamanoi, M. Midorikawa, and Y. Oda, Heterocycles, 2014, 88, 201. CrossRef
9. For example, M. Kawakubo, Y. Ito, Y. Okimura, M. Kobayashi, K. Sakura, S. Kasama, M. N. Fukuda, M. Fukuda, T. Katsuyama, and J. Nakayama, Science, 2004, 305, 1003. Other references cited therein. CrossRef
10. For example, (a) L. Feng, A. V. Perepelov, G. Zhao, S. D. Shevelev, Q. Wang, S. N. Senchenkova, A. S. Shashkov, Y. Geng, P. R. Reeves, Y. A. Knirel, and L. Wang, Microbiology, 2007, 153, 139; CrossRef (b) A. N. Kondakova, R. Fudala, S. N. Senchenkova, A. S. Shashkov, Y. A. Knirel, and W. Kaca, Carbohydr. Res., 2003, 338, 1191; CrossRef (c) A. D. Cox, J.-R. Brisson, P. Thibault, and M. B. Perry, Carbohydr. Res., 1997, 304, 191; CrossRef (d) H. Parolis, S. M. R. Stanley, A. Dell, and A. J. Reason, Carbohydr. Res., 1995, 266, 95. CrossRef
11. For example, (a) A. Takatsuki, K. Arima, and G. Tamura, J. Antibiot., 1971, 24, 215; CrossRef (b) T. Suami, H. Sasai, K. Matsuno, N. Suzuki, Y. Fukuda, and O. Sakanaka, Tetrahedron Lett., 1984, 25, 4533; CrossRef (c) A. Tordai, L. F. Brass, and E. W. Gelfand, Biochem. Biophys. Res. Commun., 1975, 65, 248. CrossRef
12. K.-F. Mo, X. Li, H. Li, L. Y. Low, C. P. Quinn, and G.-J. Boons, J. Am. Chem. Soc., 2012, 134, 15556. CrossRef
13. Manabe et al. reported the synthetic approach of the 2-acetamido-2-deoxy-α-D-glucopyranosides through the glycosidation using a N-benzyl-2,3-oxazolidinone-protected glucosamine derivative. (a) S. Manabe, K. Ishii, and Y. Ito, J. Am. Chem. Soc., 2006, 128, 10666; CrossRef (b) S. Manabe, K. Ishii, and Y. Ito, J. Org. Chem., 2007, 72, 6107; CrossRef The typical approaches for the preparation of the 2-amido-2- deoxy-α-D-glucopyranosides utilized the glycosyl donors having an azido group or a benzylideneamino group at C-2. For example, (c) J. Park, S. Kawatkar, J.-H. Kim, and G.-J. Boons, Org. Lett., 2007, 9, 1959; CrossRef (d) E. A. Mensah, F. Yu, and H. M. Nguyen, J. Am. Chem. Soc., 2010, 132, 14288. CrossRef
14. For example, (a) M. Kiso and L. Anderson, Carbohydr. Res., 1985, 136, 309; CrossRef (b) G. Arsequell, L. Krippner, R. A. Dwek, and S. Y. C. Wong, J. Chem. Soc., Chem. Commun., 1994, 2383; CrossRef (c) I. Carvalho, S. L. Scheuerl, K. P. R. Kartha, and R. A. Field, Carbohydr. Res., 2003, 338, 1039; CrossRef (d) A β-glycosidation method has been reported directly from 2-acetamido-3,4,6-tri-O-benzyl-2-deoxy- α-D-glucopyranosyl diethyl phosphate in the presence of bis(trifluoromethanesulfonyl)imide via the α-glycosyl triflate intermediate, not the oxazoline derivative. R. Arihara, S. Nakamura, and S. Hashimoto, Angew. Chem. Int. Ed., 2005, 44, 2245. CrossRef
15. The addition of a Brønsted acid, such as hydrochloric or carboxylic acid, dramatically accelerated the aldol and allylation reactions catalyzed by a Lewis acid in water. K. Manabe, Y. Mori, S. Nagayama, K. Odashima, and S. Kobayashi, Inorg. Chim. Acta, 1999, 296, 158. CrossRef
16. P. Rollin and P. Sinay, J. Chem. Soc., Perkin Trans. 1, 1977, 2513. CrossRef
17. We have also found that the glycosidation of 1 with triallylborate in the presence of Yb(OTf)3 gave allyl 2-acetamido-3,4,6-tri-O-benzyl-2-deoxy-D-glucopyranoside with a considerable amount of α-isomer. See ref. 2a.
18. We also have applied the 1,2-cis-α-aryl-D-glycosidation method to the synthesis of a β-cyclodextrin derivative conjugated with a 2-acetamido-2-deoxy-α-D-glucopyranoside residue. Y. Oda, M. Miura, K. Hattori, and T. Yamanoi, Chem. Pharm. Bull., 2009, 57, 74. CrossRef
19. (a) M. Funayama, H. Arakawa, R. Yamamoto, T. Nishino, T. Shin, and S. Murao, Biosci. Biotech. Biochem., 1995, 59, 143; CrossRef (b) K. Sugimoto, T. Nishimura, K. Nomura, K. Sugimoto, and T. Kuriki, Chem. Pharm. Bull., 2003, 51, 798. CrossRef
20. For example, (a) S. Mathew and P. Adlercreutz, Biochem. Eng. J., 2003, 79, 187; CrossRef (b) R. Prodanović, N. Milosavić, D. Sladić, M. Zlatović, B. Božić, T. C. Veličković, and Z. Vujčić, J. Mol. Catal. B: Enzym., 2005, 35, 142; CrossRef (c) K. Sugimoto, K. Nomura, T. Nishimura, T. Kiso, K. Sugimoto, and T. Kuriki, J. Biosci. Bioeng., 2005, 99, 272; CrossRef (d) J.-P. Praly, L. He, B. B. Qin, M. Tanoh, and G.-R. Chen, Tetrahedron Lett., 2005, 46, 7081; CrossRef (e) P. Wang, B. D. Martin, S. Parida, D. G. Rethwisch, and J. S. Dordick, J. Am. Chem. Soc., 1995, 117, 12885; CrossRef (f) H. Nakano, M. Shizuma, H. Murakami, T. Kiryu, and T. Kiso, J. Mol. Catal. B: Enzym., 2005, 33, 1. CrossRef
21. (a) Z.-X. Wang, X.-X. Shi, G.-R. Chen, Z.-H. Ren, L. Luo, and J. Yan, Carbohydr. Res., 2006, 341, 1945; CrossRef (b) H. G. Thomas and J.-L. Mieusset, Tetrahedron, 2008, 64, 5124. CrossRef
22. J.-C. Jacquinet and P. Sinay, Carbohydr. Res., 1976, 46, 138. CrossRef
23. A. Mbongo, C. Fréchou, D. Beaupère, R. Uzan, and G. Demailly, Carbohydr. Res., 1993, 246, 361. CrossRef
24. J. Liu and D. Y. Gin, J. Am. Chem. Soc., 2002, 124, 9789. CrossRef
25. C. D. Warren, M. A. E. Shaban, and R. W. Jeanloz, Carbohydr. Res., 1977, 59, 427. CrossRef
26. M. A. Nashed, C. W. Slife, M. Kiso, and L. Anderson, Carbohydr. Res., 1980, 82, 237. CrossRef
27. W.-S. Kim, S. Hosono, H. Sasai, and M. Shibasaki, Heterocycles, 1996, 42, 795. CrossRef