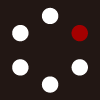
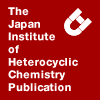
HETEROCYCLES
An International Journal for Reviews and Communications in Heterocyclic ChemistryWeb Edition ISSN: 1881-0942
Published online by The Japan Institute of Heterocyclic Chemistry
e-Journal
Full Text HTML
Received, 4th May, 2014, Accepted, 17th June, 2014, Published online, 19th June, 2014.
DOI: 10.3987/COM-14-S(K)25
■ Group-Assisted Purification (GAP) for Protection of Amino Acids Using N-Phosphonyl Functional Groups
Guanghui An, Cole Seifert, Hao Sun, Yi Pan, and Guigen Li*
Department of Chemistry & Biochemistry, Texas Tech University, Lubbock, TX 79409-1061, U.S.A.
Abstract
Various phosphonyl halides have been synthesized and utilized as protection groups for amino acids. The protection synthesis was performed via GAP (Group-Assisted Purification) procedure under convenient conditions without the use of column chromatography and recrystallization. The synthesis can be carried out on applicational scales with excellent yields (82% - 98%). The phosphonyl protection of amino acids would provide a new greener tool for GAP peptide synthesis.INTRODUCTION
Chemical synthesis of peptides and proteins heavily depends on individual N- or O-protected amino acid building blocks.1 In both solution phase and solid phase peptide synthesis, the most common protection groups have been represented by Cbz, t-Boc and Fmoc functionalities, that can be deprotected under different acidic or basic conditions.1,2 However, the synthesis of the protected amino acids by using these classical functional groups often resulted in glassy oils or poor quality of solids that required purification via either column chromatography or recrystallization. The traditional tedious purifications need large amounts of silica gels, petroleum solvents, much energy and manpower with time-consuming. More seriously, the waste generation of substantial amounts of silica gels, solvents as well as side products derived from low efficient synthesis results in environmental problem for the global environment, particularly, on industrial scale of production of amino acid and peptide drugs as well as numerous chemicals and their precursors.
In the past several years, our group has been attempting to solve this global problem and to improve the contamination/pollution image of chemical and material sciences by establishing new concepts and technologies for organic synthesis and chemical production. The GAP (Group-Assisted Purification) chemistry has thus been developed for this purpose.3-10 GAP chemistry is to introduce well engineered functional groups onto reaction substrates for synthetic reactions to result in solid products that can be purified simply by washing with common solvents or co-solvents without using column chromatography and recrystallization. In addition, the GAP functional groups or auxiliaries can be readily cleaved and recycled for re-use.
The GAP chemistry takes the advantage of solid phase peptide synthesis (for simple purification) and solution phase peptide synthesis (for large scale production). In the meanwhile, GAP chemistry can avoid their disadvantages, such as tedious purification (for the latter) and difficulties of large scale synthesis, the use of large amounts of coupling reagents, expensive resins, etc (for the former). The GAP chemistry has been proven to be efficient for a series of carbonyl addition reactions of N-phosphonyl and N-phosphinyl imines with nucleophiles.3-9 Both chiral auxiliary-controlled diastereroselective synthesis and asymmetric catalytic reactions using achiral N-phosphonyl imines have shown the great success of using GAP chemistry.4
Very recently, the GAP chemistry has been successfully utilized for the asymmetric synthesis of anti-cancer drug, Velcade, by performing asymmetric borylation of chiral N-phosphinyl imine. In this synthesis, the optically pure isomer (dr > 99:1) can be readily obtained by washing the crude mixture of the asymmetric borylation products with hexane; the chiral N-phosphinyl auxiliary has be easily recovered after deprotection was performed.9 Meanwhile, we have utilized the GAP chemistry for the solution phase synthesis of peptides, biphalin derivatives, as well as several N-protected amino acid building blocks.10 Herein, we would like to report our systematic study of N-phosphonyl protection of amino acids (Scheme 1) for complementing the traditional protecting groups of Cbz, Boc and Fmoc counterparts that have been widely employed in academic and industry for several decades.2 Our effort on this topic would result in economic, greener and environmentally friendly synthesis of protected amino acids and peptides in future.
RESULTS AND DISCUSSION
Our protection strategy was started from the synthesis of N-phosphonyl chlorides as shown in Scheme 2. These chlorides were synthesized according to the former published procedure with modifications.3,5d The commercial free diamines were first subjected to condensation reactions with aldehydes or ketones followed by reduction with by sodium borohydride (NaBH4) in ethanol at ambient temperature to give N,N'-protected diamine precusors. The N,N'-protected diamine were then reacted with POCl3 in presence of triethyl amine at rt to afford N-phosphonyl chlorides. The synthesis of 1-naphthyl branched-phosphonyl chlorides has to be conducted by refluxing the reaction mixture in toluene to get completion conversion. The N-phosphonyl chloride products were simply purified by filtering through silica gel packed short column.3,5d For the two N-phosphonyl chlorides, 2a and 2b (in Scheme 2), the purification can also be performed by washing the crude solid products with CH2Cl2/hexanes (1/1, v/v) cosolvent. In fact, the entire process for the synthesis of the above chlorides was conducted without the use of column or recrystallization, which meets the criteria of the GAP chemistry.
With these chlorides in hand, we systematically investigated the protection of various amino acids. N,N’-CH2-dinaphthylcyclohexyldiaminophosphonyl chloride (2a) was first employed for the protection of glycine methyl ester hydrochloride. The HPLC grade of commercial solvents, such as THF, dichloromethane, toluene, DMSO and DMF, were directly utilized for this reaction. Among these solvents, dichloromethane was found to perform most efficiently while others resulted in lower chemical yields (due to solubility or partial decomposing problems). We found the reaction proceeded efficiently at 90 oC to afford 92% yield (Scheme 3). The 4 Ǻ MS was found to be important for the high yield. The entire purification process can be achieved through GAP chemistry. Deprotection of the protected esters can be readily achieved under known systems, which is GAP process as well.10
Different amino acids were sujected to the protection reaction under the standard condition.10 D-Ala-OMe·HCl, L-Pro-OBn·HCl and L-Phe-OMe·HCl were protected smoothly, but the protection turned out ineffective for L-Val-OBn·HCl. Different chlorides as shown in Scheme 2 were thus employed for the protection of L-Val-OBn·HCl. We found achiral N,N’-dinaphthyl-diamino phosphonyl chloride (2b) underwent the protection smoothly to give 90% yield after the reaction was conducted 90 oC for 12 hours (Scheme 4). Other counterparts, N,N’-diisopropylphosphonyl chloride (2c) and achiral chloride (2d) resulted in incomplete conversion after their protections were performed for 12 h.
Since achiral N,N’-dinaphthyl-diaminophosphonyl chloride (2b) showed a greater scope than (2a), it was used for the protection of other amino acid esters (Table 1). For the 2b-based reaction, 2o amino acid proline also underwent protection as smoothly as 1o amino acid esters (Table 1, entry 5). Both Val and D-Ala substrates gave excellent yield, 90% and 96%, respectively (entries 1 & 6, Table 1). It should be noted that there is no need to pre-protect hydroxyl group of tyrosine benzyl ester hydrochloride prior to the protection of its amino group to achieve 90% yield (entry 7, Table 1), which is different from the previous protection by using N-diphenylphosphinyl group and N,N'-di-phenyl-N-phosphonyl group.10-11 For the use of achiral chlorides, N,N’-diisopropylphosphonyl chloride (2d) also showed similar reactivity and efficiency to give yields of 86% and 82%, respectively (entries 9 & 10, Table 1). We also employed N-diphenylphosphinyl group (Dpp)11 for the protection of L-Phe-OMe·HCl by addition of diphenyl- phosphinyl chloride into the CH2Cl2 solution consisting of N-methylmorpholine and L-Phe-OMe·HCl at 0 oC followed by stirring at rt overnight to give 4a in 90% yield. All of above protection processes met the GAP chemistry criteria without requiring recrystallization and column chromatography. However, when the hydrolyzed product 4b was utilized for peptide synthesis, racemization was observed.
After we took the advantage of N-phosphonyl chlorides, we also synthesized N-phosphonyl bromides attempting to further improve the above protection, and more importantly, to synthesize N-phosphonyl amides directly by treating N-phosphonyl bromides with amonia, which failed for this synthesis using N-phosphonyl chlorides.3 During this synthesis, N,N’-dinaphthylcyclohexyldiamine was first treated with POBr3 and dried triethyl amine in dichloromethane at rt to give the bromide 2e in 80% yield (Scheme 6). Since N,N'-diisopropyl-N-phosphonyl bromide is not very stable under air and moisture, we conducted the in situ generation of N-phosphonyl bromide followed by the direct reaction with ammonia. The reaction was performed by stirring pre-formed N-phosphonyl bromide at – 40 oC for 2 h and then at rt overnight to afford amide 2g in an overall yield of 90% via GAP work-up (Scheme 6). Further investigation of N-phosphonyl bromide for large-scale production of N-phosphonyl amides will be conducted in due course.
In conclusion, the synthesis of various N-phosphonyl chlorides and bromides and the protection of amino acid esters has been developed. The in situ generation of of N-phosphonyl amides can avoid the use of sodium azide making their large-scale production of more convenient. The above synthesis can be conducted through GAP work-up without the use of chromatography and recrystallization. The new protected amino acids will be applied on peptide and peptidomimetic research in future.
EXPERIMENTAL
All commercially available solvents, unless otherwise mentioned, were used without purification. 4 Ǻ MS was heated for over 72 h before utility. All melting points are uncorrected. The NMR spectra were recorded at 400, 125, 202 MHz for 1H, 13C and 31P respectively. Shifts are reported in ppm based on an internal TMS standard (for 1H/CDCl3) or on residual solvent peaks (for 13C/CDCl3). 31P NMR spectra were referenced to external H3PO4 (0.00 ppm). Flash chromatographic columns were carried out on silica gel 60 (230-400 mesh). Spectra of compound 2a, 2c were compared to the literature data. Synthesis of compound 2d are similar to the compound 2c.3-5
General procedure for synthesis of N, N’-dinaphthyldiaminophosphonyl chloride (2a and 2b):
To diamine’s toluene solution (12 g diamine in 120 mL toluene) in 500 mL single-necked flask at 0 oC, 4 mL POCl3 followed by 10.1 mL Et3N were slowly added with stirring vigorously. The reaction solution is stirring at refluxing until the reaction finished. The reaction mixture was filter through the Celite in Büchner’s funnel and the filtrate cake was washed by toluene for 3 times. Collect all filtrates and evaporated on rotavapor. Add CH2Cl2 to dissolve the residue and wash it with 1 M HCl solution and brine. Dry over Na2SO4 and evaporate it. The solid obtained can be purified by simple washing with hexane/CH2Cl2 solution to give the chlorides as white solid.
Compound 2b: white solid; mp 114-116 oC; 1H NMR (CDCl3, 400 MHz): δ = 8.30 (d, J = 8.5 Hz, 2H), 7.84 (dd, J = 18.3, 8.0 Hz, 4H), 7.65 – 7.35 (m, 8H), 4.91 (dd, J = 14.4, 7.8 Hz, 2H), 4.36 (dd, J = 14.0, 4.6 Hz, 2H), 2.90 (d, J = 11.3 Hz, 4H).13C NMR (CDCl3, 101 MHz): δ = 133.95, 131.75, 131.11 (d, J = 9.6 Hz), 129.12, 128.74, 127.22, 126.81, 126.21, 125.22, 123.82, 47.22 (d, J = 4.9 Hz), 43.02 (d, J = 13.4 Hz). 31P NMR (CDCl3, 162 MHz) δ = 27.83. HRMS (ESI) m/z calcd for C24H22ClN2OPNa 443.1056, found 443.1051.
Compound 2d: colorless liquid; 1H NMR (CDCl3, 400 MHz): δ = 3.63 – 3.49 (m, 2H), 3.30 – 3.15 (m, 2H), 3.09 – 2.97 (m, 2H), 1.18 (ddd, J = 12.6, 6.6, 2.0 Hz, 12H). 13C NMR (CDCl3, 101 MHz): δ = 45.31 (d, J = 4.8 Hz), 38.25 (d, J = 15.1 Hz), 20.97 (d, J = 4.7 Hz), 19.84 (d, J = 3.8 Hz). 31P NMR (CDCl3, 162 MHz) δ = 23.68. HRMS (ESI) m/z calcd for C8H18ClN2OPNa 247.0743, found 247.0749.
General procedure for synthesis of phosphonyl bromide:
To diamine’s toluene solution (12 g diamine in 120 mL toluene) in 500 mL single-necked flask at 0 oC, 6 g POBr3 followed by 10.1 mL Et3N were slowly added with stirring vigorously. The reaction solution is stirring at 0 oC until the reaction finished. The reaction mixture was filter through the Celite in Büchner’s funnel and the filtrate cake was washed by toluene for 3 times. Collect all filtrates and evaporated on rotavapor. Add CH2Cl2 to dissolve the residue and wash it with 1 M HCl solution and brine. Dry over Na2SO4 and evaporate it. The solid you obtain can be purified by simple washing with hexane/CH2Cl2 solution. 9 g of bromide 2e was obtained as brownish solid.
Compound 2e: brownish solid; mp 216-218 oC; [α]D25 - 42.6 (c 1.0, CH2Cl2); 1H NMR (CDCl3, 400 MHz): δ = 8.28 (d, J = 8.5 Hz, 1H), 8.07 (d, J = 8.4 Hz, 1H), 7.88 (dd, J = 8.0, 1.3 Hz, 2H), 7.76 (ddd, J = 23.1, 11.9, 5.0 Hz, 4H), 7.64 – 7.40 (m, 6H), 5.10 (dd, J = 16.5, 12.1 Hz, 1H), 4.93 (t, J = 15.1 Hz, 1H), 4.63 (dd, J = 16.7, 11.7 Hz, 1H), 4.24 (dd, J = 15.7, 8.8 Hz, 1H), 3.26 (t, J = 10.5 Hz, 1H), 3.04 (t, J = 10.6 Hz, 1H), 1.72 (d, J = 11.8 Hz, 1H), 1.59 (dd, J = 22.6, 12.5 Hz, 3H), 1.32 – 1.12 (m, 2H), 1.11 – 0.93 (m, 2H). 13C NMR (CDCl3, 101 MHz): δ = 133.75 (d, J = 11.8 Hz), 132.73, 132.71, 132.68, 131.38, 130.96, 128.88 (d, J = 10.7 Hz), 128.51, 128.07 , 126.57, 126.45, 126.37, 125.88 (d, J = 8.3 Hz), 125.47, 125.25, 123.57, 122.78, 64.91 (d, J = 9.2 Hz), 63.39 (d, J = 10.1 Hz), 46.65 (d, J = 3.1 Hz), 44.75 (d, J = 5.0 Hz), 29.34 (d, J = 7.1 Hz), 28.85 (d, J = 13.3 Hz), 24.26 , 23.90. 31P NMR (CDCl3, 162 MHz) δ = 25.03. HRMS (ESI) m/z calcd for C28H28BrN2OPNa 541.1020, found 541.1013.
Synthesis of phosphonyl amide from bromide:
To a flame dried flask, bromide 2e (5.19 g, 10.0 mmol), ammonium chloride (20.0 mmol), triethylamine (4.2 mL, 3.0 mmol), 4A MS (5g) and CH2Cl2 (20 mL) was added in sequence. The reaction flask was sealed and stirred at 90 oC until the bromide was consumed up. After it’s cooled to room temperature, the reaction mixture was filter through the Celite and the filtrate cake was washed by CH2Cl2 for 3 times. Collect all filtrates and wash it with 1 M HCl solution and brine. Dry over Na2SO4 and evaporate it. The solid can be purified by simple washing with hexane/CH2Cl2 solution.
Amide 2f: white solid; 1H NMR (CDCl3, 400 MHz): δ = 8.16 (dd, J = 15.1, 8.2 Hz, 2H), 7.85 (t, J = 7.8 Hz, 2H), 7.75 (dd, J = 12.6, 6.4 Hz, 4H), 7.60 – 7.36 (m, 6H), 4.86 (ddd, J = 17.2, 11.1, 6.1 Hz, 2H), 4.55 – 4.41 (m, 1H), 4.35 (dd, J = 16.5, 8.0 Hz, 1H), 3.08 (dd, J = 12.9, 6.4 Hz, 1H), 2.95 (t, J = 9.9 Hz, 1H), 2.31 (d, J = 3.9 Hz, 2H), 1.68 – 1.50 (m, 3H), 1.32 – 0.85 (m, 5H). 31P NMR (CDCl3, 162 MHz) δ = 28.33.
One-pot procedure for synthesis of phosphonyl amide:
To the diamine (7.5 mmol) solution of CH2Cl2 (15 mL) in the three-necked flask, POBr3 (1.2 equiv.) was added under N2. Triethylamine (3 equiv.) was added at 0 oC. The resulting mixture was warmed to room temperature slowly and stirred overnight. The reaction system was cooled to -40 oC and plugged with a balloon. The ammonia gas was injected into the solution via pipe carefully till the balloon grew up. The reaction was stirred for 2 h and warmed to room temperature slowly. After stirring overnight at room temperature, the mixture was filtered and washed with CH2Cl2. The filtrate was extracted with water. The organic layer was dried and evaporated to give the amide product as a white solid in 90% yield.
Amide 2g: white solid; 1H NMR (CDCl3, 400 MHz): δ = 3.57 – 3.37 (m, 2H), 2.94 – 2.85 (m, 1H), 2.76 – 2.62 (m, 1H), 2.43 (s, 2H), 2.02 (d, J = 9.6 Hz, 2H), 1.80 – 1.61 (m, 3H), 1.38 – 1.12 (m, 15H). 31P NMR (CDCl3, 162 MHz) δ = 24.22.
General procedure for the protection of amino acid esters:
To a flame dried flask, chloride (10.0 mmol), amino acid ester (12.0 -15.0 mmol), triethylamine (4.2 mL, 30.0 mmol), 4A MS (5 g) and CH2Cl2 (20 mL) was added in sequence. The reaction flask was sealed and stirred at 90 oC until the chloride was consumed up. After it’s cooled to room temperature, the reaction mixture was filter through the Celite and the filtrate cake was washed by CH2Cl2 for 3 times. Collect all filtrates and wash it with water, 1 M HCl solution and brine. Dry over Na2SO4 and evaporate it. The solid can be purified by simple washing with hexane/CH2Cl2 solution.
Compound 3a: white solid; mp 98-99 oC; [α]D25 - 82.8 (c 1.0, CH2Cl2); 1H NMR (CDCl3, 400 MHz): δ = 8.14 (dd, J = 8.3, 2.3 Hz, 2H), 7.86 – 7.70 (m, 6H), 7.55 – 7.41 (m, 6H), 4.80 (dd, J = 16.6, 11.2 Hz, 1H), 4.68 (dd, J = 14.5, 9.4 Hz, 1H), 4.59 – 4.47 (m, 1H), 4.33 (dd, J = 16.6, 8.6 Hz, 1H), 3.45 (s, 3H), 3.24 (ddd, J = 17.8, 9.3, 6.3 Hz, 1H), 3.16 – 3.04 (m, 2H), 3.03 – 2.93 (m, 1H), 2.62 (dt, J = 9.9, 5.9 Hz, 1H), 2.02 (d, J = 11.7 Hz, 1H), 1.71 (d, J = 11.4 Hz, 1H), 1.59 (t, J = 12.5 Hz, 2H), 1.39 – 1.04 (m, 4H). 13C NMR (CDCl3, 101 MHz): δ = 171.20 (d, J = 8.7 Hz), 135.22 (d, J = 4.7 Hz), 133.76, 133.67, 133.63, 131.62, 131.05, 128.78, 128.07, 127.56, 127.26, 126.08 (d, J = 1.8 Hz), 125.64, 125.59, 125.56, 125.53, 125.42, 123.48, 123.05, 65.26 (d, J = 10.1 Hz), 63.71 (d, J = 8.8 Hz), 53.61, 52.05, 45.39 (d, J = 2.0 Hz), 44.51 (d, J = 4.5 Hz), 42.61 (d, J = 1.7 Hz), 29.93 (d, J = 8.3 Hz), 29.37 (d, J = 10.6 Hz), 24.36 (d, J = 9.9 Hz). 31P NMR (CDCl3, 162 MHz) δ = 27.59. HRMS (ESI) m/z calcd for C31H34N3O3PNa 550.2235, found 550.2230.
Compound 3b: white solid; mp 144-146 oC; [α]D25 - 11.9 (c 1.0, CH2Cl2); 1H NMR (CDCl3, 400 MHz): δ = 8.28 (d, J = 8.4 Hz, 1H), 8.21 (d, J = 8.3 Hz, 1H), 7.86 (d, J = 7.8 Hz, 2H), 7.78 (dd, J = 8.0, 3.0 Hz, 2H), 7.59 – 7.40 (m, 8H), 7.25 – 7.19 (m, 5H), 4.99 (q, J = 12.2 Hz, 2H), 4.73 – 4.39 (m, 4H), 4.00 – 3.90 (m, 1H), 3.24 – 3.13 (m, 1H), 3.10 – 2.84 (m, 4H), 2.06 (dd, J = 12.7, 6.3 Hz, 1H), 0.93 (dd, J = 26.8, 6.8 Hz, 6H). 13C NMR (CDCl3, 101 MHz): δ = 173.89 (d, J = 2.2 Hz), 135.38, 133.84 (d, J = 2.8 Hz), 133.00 (dd, J = 7.6, 6.3 Hz), 131.79 (d, J = 10.2 Hz), 128.70 (d, J = 4.9 Hz), 128.63, 128.51, 128.48, 128.27, 128.14, 126.41 (d, J = 5.8 Hz), 126.13, 125.91 (d, J = 2.2 Hz), 125.58, 125.36 (d, J = 6.6 Hz), 123.71, 123.50, 67.00, 60.17, 46.72 (d, J = 5.1 Hz), 46.52 (d, J = 5.1 Hz), 44.55 (d, J = 8.5 Hz), 44.43 (d, J = 8.1 Hz), 32.69 (d, J = 6.5 Hz), 19.02, 17.97. 31P NMR (CDCl3, 162 MHz) δ = 25.02. HRMS (ESI) m/z calcd for C36H38N3O3PNa 614.2548, found 614.2552.
Compound 3c: white solid; mp 120-121 oC; 1H NMR (CDCl3, 400 MHz): δ = 8.23 (d, J = 8.3 Hz, 2H), 7.88 – 7.81 (m, 2H), 7.77 (d, J = 8.1 Hz, 2H), 7.51 (ddd, J = 17.7, 10.4, 3.7 Hz, 6H), 7.45 – 7.37 (m, 2H), 4.67 – 4.47 (m, 4H), 3.62 (s, 3H), 3.57 (dd, J = 10.0, 6.2 Hz, 2H), 3.39 – 3.29 (m, 1H), 3.09 – 2.88 (m, 4H). 13C NMR (CDCl3, 101 MHz): δ = 171.79 (d, J = 7.6 Hz), 133.91, 133.02 (d, J = 7.1 Hz), 131.79, 128.73, 128.41, 126.51, 126.40, 125.92, 125.36, 123.62, 52.27, 46.82 (d, J = 5.1 Hz), 44.77 (d, J = 12.3 Hz), 43.17 (d, J = 1.3 Hz). 31P NMR (CDCl3, 162 MHz) δ = 24.90. HRMS (ESI) m/z calcd for C27H28N3O3PNa 496.1766, found 496.1762.
Compound 3d: white solid; mp 155-157 oC; 1H NMR (CDCl3, 400 MHz): δ = 8.25 (d, J = 8.2 Hz, 2H), 7.81 (dd, J = 26.7, 7.9 Hz, 4H), 7.56 – 7.37 (m, 8H), 7.31 (d, J = 3.9 Hz, 5H), 5.08 (s, 2H), 4.58 (d, J = 6.1 Hz, 4H), 3.63 (dd, J = 9.8, 6.2 Hz, 2H), 3.51 (dd, J = 10.3, 5.9 Hz, 1H), 2.95 (dd, J = 9.2, 4.8 Hz, 4H). 13C NMR (CDCl3, 101 MHz): δ = 171.19 (d, J = 8.0 Hz), 135.32, 133.94, 133.09 (d, J = 7.2 Hz), 131.83, 128.76, 128.74, 128.65, 128.59, 128.43, 126.58, 126.43, 125.95, 125.39, 123.68, 67.16, 46.85 (d, J = 5.1 Hz), 44.77 (d, J = 12.3 Hz), 43.38. 31P NMR (CDCl3, 162 MHz) δ = 24.83. HRMS (ESI) m/z calcd for C33H32N3O3PNa 572.2079, found 572.2082.
Compound 3e: white solid; mp 115-117 oC; [α]D25 5.1 (c 1.0, CH2Cl2); 1H NMR (CDCl3, 400 MHz): δ = 8.26 (d, J = 8.4 Hz, 1H), 8.13 (d, J = 8.3 Hz, 1H), 7.85 (d, J = 8.0 Hz, 2H), 7.78 (d, J = 8.4 Hz, 2H), 7.59 – 7.41 (m, 8H), 7.20 – 7.08 (m, 5H), 4.57 – 4.43 (m, 2H), 4.37 – 4.19 (m, 3H), 3.52 (d, J = 1.0 Hz, 3H), 3.21 – 3.09 (m, 1H), 3.08 – 2.84 (m, 6H). 13C NMR (CDCl3, 101 MHz): δ = 174.26 (d, J = 2.4 Hz), 136.49 (d, J = 2.4 Hz), 133.87, 133.82, 133.18, 133.11, 133.09 (d, J = 1.0 Hz), 133.01, 131.85, 131.68, 129.57, 128.74, 128.70, 128.66, 128.30, 128.04, 127.14, 126.40, 126.37, 126.24, 125.92 (d, J = 2.0 Hz), 125.41 (d, J = 1.8 Hz), 125.16, 123.82, 123.45, 56.25, 52.21, 46.36 (d, J = 5.0 Hz), 46.19 (d, J = 5.1 Hz), 44.60 (d, J = 3.6 Hz), 44.48 (d, J = 3.2 Hz), 41.02 (d, J = 6.7 Hz). 31P NMR (CDCl3, 162 MHz) δ = 24.21. HRMS (ESI) m/z calcd for C34H34N3O3PNa 586.2235, found 586.2239.
Compound 3f: white solid; mp 134-136 oC; [α]D25 - 71.9 (c 1.0, CH2Cl2); 1H NMR (CDCl3, 400 MHz): δ = 8.49 (d, J = 8.1 Hz, 1H), 8.21 (d, J = 8.0 Hz, 1H), 7.81 (dd, J = 25.7, 7.5 Hz, 4H), 7.48 (dd, J = 37.9, 21.8 Hz, 8H), 7.25 (dd, J = 27.9, 6.7 Hz, 5H), 5.13 (s, 2H), 4.90 (dd, J = 14.0, 5.4 Hz, 1H), 4.60 – 4.32 (m, 3H), 4.12 (d, J = 6.8 Hz, 1H), 3.17 (s, 1H), 3.08 – 2.78 (m, 5H), 1.78 (s, 1H), 1.56 (s, 1H), 1.32 (s, 1H), 0.99 – 0.82 (m, 1H). 13C NMR (CDCl3, 101 MHz): δ = 175.02, 136.05, 134.03, 133.98, 133.87, 133.41, 132.12 (d, J = 12.9 Hz), 128.78, 128.58, 128.50, 128.26, 128.18, 127.34, 126.98, 126.28, 125.98, 125.92, 125.57, 125.36, 124.60, 124.00, 66.67, 64.57, 60.71 (d, J = 6.7 Hz), 47.77, 46.93, 45.81 (d, J = 12.7 Hz), 44.73 (d, J = 12.3 Hz), 31.04 (d, J = 8.4 Hz), 25.10 (d, J = 7.8 Hz). 31P NMR (CDCl3, 162 MHz) δ = 22.96. HRMS (ESI) m/z calcd for C36H36N3O3PNa 612.2392, found 612.2397.
Compound 3g: white solid; mp 155-156 oC; [α]D25 - 30.1 (c 1.0, CH2Cl2); 1H NMR (CDCl3, 400 MHz): δ = 8.26 (d, J = 8.4 Hz, 1H), 8.21 (d, J = 8.4 Hz, 1H), 7.85 (d, J = 8.1 Hz, 2H), 7.78 (dd, J = 8.0, 3.5 Hz, 2H), 7.61 – 7.26 (m, 13H), 5.05 (s, 2H), 4.65 – 4.52 (m, 3H), 4.41 (dd, J = 15.1, 6.3 Hz, 1H), 4.11 – 3.98 (m, 1H), 3.32 (t, J = 10.1 Hz, 1H), 3.03 – 2.88 (m, 4H), 1.36 (d, J = 7.1 Hz, 3H). 13C NMR (CDCl3, 101 MHz): δ = 174.60 (d, J = 4.3 Hz), 135.45, 133.87 (d, J = 2.7 Hz), 133.05 (t, J = 7.2 Hz), 131.82 (d, J = 4.6 Hz), 128.70 (d, J = 2.6 Hz), 128.50, 128.30 (d, J = 5.6 Hz), 128.29 (d, J = 0.8 Hz), 126.42 (d, J = 2.3 Hz), 126.31, 126.00, 125.93 (d, J = 3.9 Hz), 125.39, 123.66 (d, J = 12.8 Hz), 67.11, 50.45, 46.88 (d, J = 4.8 Hz), 46.51 (d, J = 5.1 Hz), 44.76 (d, J = 12.3 Hz), 44.45 (d, J = 12.3 Hz), 21.69 (d, J = 5.7 Hz). 31P NMR (CDCl3, 162 MHz) δ = 23.99. HRMS (ESI) m/z calcd for C28H30N3O3PNa 510.1922, found 510.1927.
Compound 3h: white solid; mp 110 oC start decompose; [α]D25 - 41.9 (c 1.0, CH2Cl2); 1H NMR (CDCl3, 400 MHz): δ = 8.93 (s, 1H), 8.21 – 8.13 (m, 1H), 8.11 – 8.03 (m, 1H), 7.90 – 7.69 (m, 4H), 7.45 (ddd, J = 11.1, 8.2, 5.2 Hz, 6H), 7.15 (dd, J = 4.8, 1.7 Hz, 2H), 6.75 (s, 4H), 5.02 – 4.78 (m, 2H), 4.55 – 4.07 (m, 5H), 3.19 (t, J = 10.2 Hz, 1H), 3.08 – 2.73 (m, 6H). 13C NMR (CDCl3, 101 MHz): δ = 173.72, 156.75, 135.19, 133.81, 132.78 (d, J = 7.4 Hz), 131.72 (d, J = 7.8 Hz), 130.53, 128.72, 128.66, 128.60, 128.54, 128.33, 128.13, 126.58, 126.45, 126.37, 126.13, 125.96, 125.41, 123.70, 123.48, 115.86, 67.24, 56.27, 46.29 (d, J = 4.2 Hz), 46.10 (d, J = 5.5 Hz), 44.67 (d, J = 13.5 Hz), 44.38 (d, J = 12.1 Hz), 40.06. 31P NMR (CDCl3, 162 MHz) δ = 24.47. HRMS (ESI) m/z calcd for C40H38N3O4PNa 678.2498, found 678.2501.
Compound 3i: white solid; mp 130-131 oC; [α]D25 - 32.9 (c 1.0, CH2Cl2); 1H NMR (CDCl3, 400 MHz): δ = 8.28 (dd, J = 19.9, 8.4 Hz, 2H), 7.90 (d, J = 7.8 Hz, 2H), 7.82 (dd, J = 7.7, 3.3 Hz, 2H), 7.65 – 7.40 (m, 9H), 7.28 – 7.24 (m, 4H), 5.12 – 5.00 (m, 2H), 4.72 – 4.42 (m, 4H), 4.17 – 4.00 (m, 1H), 3.15 – 2.91 (m, 5H), 1.75 – 1.57 (m, 2H), 1.46 (dt, J = 13.4, 7.2 Hz, 1H), 0.89 (dd, J = 9.6, 6.4 Hz, 6H). 13C NMR (CDCl3, 101 MHz): δ = 174.78 (d, J = 2.5 Hz), 135.39, 133.85 (d, J = 4.1 Hz), 133.13, 133.05, 132.98, 131.81 (d, J = 10.1 Hz), 128.69, 128.62, 128.44, 128.38 (d, J = 1.3 Hz), 128.29, 128.19, 126.45, 126.37, 126.24, 125.90 (d, J = 2.3 Hz), 125.82, 125.36 (d, J = 1.3 Hz), 123.73, 123.54, 66.99, 53.57, 46.68 (d, J = 5.0 Hz), 46.41 (d, J = 5.2 Hz), 44.67 (d, J = 12.3 Hz), 44.43 (d, J = 9.1 Hz), 44.33 (d, J = 3.3 Hz), 24.82, 22.57, 22.46. 31P NMR (CDCl3, 162 MHz) δ = 24.49. HRMS (ESI) m/z calcd for C37H40N3O3PNa 628.2705, found 628.2709.
Compound 3j: white solid; mp 90-91 oC; 1H NMR (CDCl3, 400 MHz): δ = 3.64 – 3.47 (m, 3H), 3.47 – 3.24 (m, 4H), 3.09 – 2.73 (m, 5H), 1.16 – 0.71 (m, 12H). 13C NMR (CDCl3, 101 MHz): δ = 172.03 (d, J = 5.5 Hz), 51.91, 43.86 (d, J = 5.2 Hz), 43.30, 38.42 (d, J = 13.7 Hz), 20.98. 31P NMR (CDCl3, 162 MHz) δ = 21.55. HRMS (ESI) m/z calcd for C11H24N3O3PNa 300.1453, found 300.1458.
Compound 3k: white solid; mp 99-100 oC; [α]D25 - 4.3 (c 1.0, CH2Cl2); 1H NMR (CDCl3, 400 MHz): δ = 7.45 – 7.02 (m, 5H), 4.04 – 3.85 (m, 1H), 3.60 (s, 3H), 3.35 (ddd, J = 19.5, 13.3, 6.6 Hz, 2H), 3.18 – 2.71 (m, 7H), 1.34 – 0.68 (m, 12H). 13C NMR (CDCl3, 101 MHz): δ = 174.10 (d, J = 2.7 Hz), 136.32, 129.48, 128.56, 127.05, 56.02, 51.86, 43.83 (dd, J = 9.6, 5.3 Hz), 41.37 (d, J = 6.3 Hz), 38.09 (dd, J = 21.7, 13.8 Hz), 21.29 (dd, J = 12.6, 6.1 Hz), 21.01 (d, J = 27.8 Hz). 31P NMR (CDCl3, 162 MHz) δ = 21.30. HRMS (ESI) m/z calcd for C18H30N3O3PNa 390.1922, found 390.1920.
Procedure for the protection of amino acid esters with diphenylphosphinyl chloride:
To a mixture of NMM (3 mmol), amino acid ester (2 mmol) and CH2Cl2 (10 mL) was added Dpp-Cl (1 mmol) in CH2Cl2 (5 mL) solution at 0 oC. The resulting mixture was stirred at room temperature for 24 hours. The mixture was then filtered through Celite, washed with by 5% citric acid aqueous solution, sat. aqueous NaHCO3 solution, H2O and then brine, dried over Na2SO4, filtered, and evaporated to give a white solid.
Compound 4a: white solid; 1H NMR (CDCl3, 400 MHz): δ = 7.85 – 7.76 (m, 2H), 7.70 – 7.62 (m, 2H), 7.52 – 7.38 (m, 4H), 7.37 – 7.31 (m, 2H), 7.30 – 7.20 (m, 3H), 7.16 – 7.10 (m, 2H), 4.10 – 4.00 (m, 1H), 3.64 (s, 3H), 3.53 (dd, J = 10.8, 7.0 Hz, 1H), 3.08 (dd, J = 6.0, 2.2 Hz, 2H). 13C NMR (CDCl3, 101 MHz): δ = 173.35 (d, J = 5.8 Hz), 136.08, 132.70 (d, J = 10.9 Hz), 132.24 (d, J = 9.7 Hz), 132.14 (d, J = 2.8 Hz), 132.03 (d, J = 2.8 Hz), 132.03 (d, J = 9.5 Hz), 131.42 (d, J = 9.5 Hz), 129.79 (d, J = 1.3 Hz), 128.70 (d, J = 10.2 Hz), 128.59, 128.57 (d, J = 10.1 Hz), 127.14, 54.88, 52.33, 41.35 (d, J = 4.9 Hz). 31P NMR (CDCl3, 162 MHz) δ = 23.76.
Procedure for hydrolysis of Dpp-Phe-OMe (4a):
To a flask, compound 4a (1.0 mmol) was suspended in a 10 mL MeOH/H2O (v:v, 9:1) solution. The mixture was cooled to 0 oC followed by addition of LiOH·H2O (5 mmol). The reaction was allowed to warm to room temperature and stirred overnight. HCl (1.0 M aqueous solution) was added to neutralize the reaction. The water phase was extracted by CH2Cl2 for 3 times. Combine all organic phase. Dry over Na2SO4 and evaporate to get the corresponding acid.
Compound 4b: white solid; 1H NMR (CDCl3, 400 MHz): δ = 13.10 (d, J = 75.9 Hz, 1H), 7.79 (ddd, J = 10.5, 3.9, 2.3 Hz, 2H), 7.50 – 7.13 (m, 13H), 3.94 – 3.76 (m, 1H), 3.69 (dd, J = 8.2, 4.4 Hz, 1H), 3.18 (d, J = 13.4 Hz, 1H), 2.95 – 2.76 (m, 1H). 13C NMR (CDCl3, 101 MHz): δ = 174.33, 137.24, 132.78 (d, J = 10.0 Hz), 132.47, 132.14, 131.88 (d, J = 9.8 Hz), 130.83, 130.11, 129.77, 128.76, 128.61, 128.53, 126.97, 55.83, 40.79 (d, J = 9.9 Hz). 31P NMR (CDCl3, 162 MHz) δ = 26.77.
ACKNOWLEDGEMENTS
We gratefully acknowledge the financial support from the NIH (R33DA031860), the Robert A. Welch Foundation (D-1361), the National Natural Science Foundation of China (21332005) and Jiangsu Educational Innovation Team Program (P.R. China) for their generous support of this research. We also thank NSF Grant CHE-1048553 and the CRIF program for supporting our NMR facility.
References
1. (a) A. B. Hughes, Amino Acids, Peptides and Proteins in Organic Chemistry, Wiley–VCH, Weinheim, Germany, 2011, vol. 3; (b) A. B. Hughes, Amino Acids, Peptides and Proteins in Organic Chemistry, Wiley–VCH, Weinheim, Germany, 2011, vol. 4; (c) N. Sewald and H. Jakubke, Peptides: Chemistry and Biology, Wiley–VCH, Weinheim, Germany, 2009, 2nd Ed.; (d) A. Isidro–Llobet, M. Alvarez, and F. Albericio, Chem. Rev., 2009, 109, 2455. CrossRef
2. (a) P. G. M. Wuts and T. W. Greene, Protective Groups in Organic Synthesis, Wiley, 2006; CrossRef (b) P. J. Kocienski, Protecting Groups, Georg Thieme Verlag, 2005.
3. (a) A. Kattuboina and G. Li, Tetrahedron Lett., 2008, 49, 1573; CrossRef (b) A. Kattuboina, P. Kaur, T. Ai, and G. Li, Chem. Biol. Drug Des., 2008, 71, 216; CrossRef (c) A. Kattuboina, P. Kaur, T. Nguyen, and G. Li, Tetrahedron Lett., 2008, 49, 3722; CrossRef (d) J. Han, T. Ai, T. Nguyen, and G. Li, Chem. Biol. Drug Des., 2008, 72, 120. CrossRef
4. P. Kaur, S. Pindi, W. Wever, T. Rajale, and G. Li, Chem. Commun., 2010, 46, 4330. CrossRef
5. (a) T. Ai, J. Han, Z.–X. Chen, and G. Li, Chem. Biol. Drug Des., 2009, 73, 203; CrossRef (b) T. Ai and G. Li, Bioorg. Med. Chem. Lett., 2009, 19, 3967; CrossRef (c) Z.–X. Chen, T. Ai, P. Kaur, and G. Li, Tetrahedron Lett., 2009, 50, 1079; CrossRef (d) J. Han, T. Ai, and G. Li, Synthesis, 2008, 2519; (e) P. Kaur, T. Nguyen, and G. Li, Eur. J. Org. Chem., 2009, 912.
6. (a) P. Kaur, G. Shakya, H. Sun, Y. Pan, and G. Li, Org. Biomol. Chem., 2010, 8, 1091; CrossRef (b) P. Kaur, W. Wever, S. Pindi, R. Milles, P. Gu, M. Shi, and G. Li, Green Chem., 2011, 13, 1288; CrossRef (c) P. Kaur, W. Wever, T. Rajale, and G. Li, Chem. Biol. Drug Des., 2010, 74, 314; CrossRef (d) H. Sun, H. Zhang, J. Han, Y. Pan, and G. Li, Eur. J. Org. Chem., 2013, 4744. CrossRef
7. (a) For chiral N–phosphinyl imine chemistry, see: S. Pindi, P. Kaur, G. Shakya, and G. Li, Chem. Biol. Drug Des., 2010, 77, 20; CrossRef (b) For chiral N–phosphonylyl imine chemistry, see: H. Sun, T. Rajale, Y. Pan, and G. Li, Tetrahedron Lett., 2010, 51, 4403; CrossRef (c) For chiral sulfinyl imine, see: S. Pindi, J. B. Wu, and G. Li, J. Org. Chem., 2013, 78, 4006. CrossRef
8. For umpolung reaction, see: P. V. Kattamuri, T. Ai, S. Pindi, Y. Sun, P. Gu, M. Shi, and G. Li, J. Org. Chem., 2011, 76, 2792; CrossRef For GAP aminohalogenation, see: H. Sun, J. L. Han, P. V. Kattamuri, Y. Pan, and G. Li, J. Org. Chem., 2013, 78, 1171. CrossRef
9. J. B. Xie, J. Luo, T. R. Winn, D. Cordes, and G. Li, Beilstein J. Org. Chem., 2014, 10, 746. CrossRef
10. J. B. Wu, G. H. An, S. Q. Lin, J. B. Xie, W. Zhou, H. Sun, Y. Pan, and G. Li, Chem. Commun., 2014, 50, 1259. CrossRef
11. G. W. Kenner, G. A. Moore, and R. Ramage, Tetrahedron Lett., 1976, 17, 3623. CrossRef