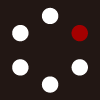
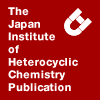
HETEROCYCLES
An International Journal for Reviews and Communications in Heterocyclic ChemistryWeb Edition ISSN: 1881-0942
Published online by The Japan Institute of Heterocyclic Chemistry
e-Journal
Full Text HTML
Received, 27th June, 2014, Accepted, 13th August, 2014, Published online, 20th August, 2014.
DOI: 10.3987/COM-14-S(K)47
■ Synthesis and Biological Evaluation of C-Aromataxane Derivatives as P-Glycoprotein-Mediated Multi Drug Resistance Reversal Agents
Takayuki Doi,* Naoko Yamaguchi, Kosuke Ohsawa, Kazuoki Nakai, Masahito Yoshida, Kazuhiro Satake, Yuji Mitani, Hiroshi Nakagawa, Takashi Takahashi, and Toshihisa Ishikawa
Graduate School of Pharmaceutical Science, Tohoku University, Aramaki, Aoba-ku, Sendai 980-8578, Japan
Abstract
Synthesis and evaluation of C-aromataxane derivatives as P-glycoprotein-mediated MDR reversal agents have been demonstrated. Several derivatives possessing N-benzoylphenylisoserine at the C2 or C14 position of the template 2a were readily synthesized and were evaluated their affinity for P-glycoprotein. Most of the synthesized derivatives exhibited much lower cytotoxicity in both KB-3-1 cells and MDR KB-G2-cells than paclitaxel (1), and it should be noted that the compound (14R)-5a exhibited high Km and Vmax/Km values, and cytotoxicity of paclitaxel (1) in MDR KB-G2 cells was significantly recovered (98% reduction, IC50 30 nM) in the presence of 5a (5.0 μM). The structural features such as endo-cage conformation and the stereochemistry at the C14 position is crucial to exhibit an excellent affinity for P-glycoprotein.INTRODUCTION
Multidrug resistance (MDR) in human cancer is one of the serious problems in the long-term chemotherapy, which significantly reduces the pharmacological effect of anticancer drugs, such as paclitaxel (1) and vincristine.1 Cancer cells often acquire resistance for antitumor drugs by accretion of target enzymes, decrement of affinity or enhancement of inactive enzyme for drugs and overexpression of P-glycoprotein. Among them, P-glycoprotein-associated MDR is a serious problem because P-glycoprotein extrudes various foreign substrates to outside of cells.2 In addition, the fact that P-glycoprotein exists not only cancer cells but also normal one makes it difficult to develop novel drugs, selectively targeting P-glycoprotein of cancer cells. Thus, development of MDR reversal agents has been vigorously investigated to solve this critical issue in recent decades. For instance, isolated taxane compounds are usually utilized as a template of MDR reversal agent due to the similar structure for paclitaxel (1), and structure activity relationships (SAR) provide the derivatives with lower cytotoxic and higher MDR reversal activity.3 On the other hand, unnatural synthetic taxane derivatives would also be interested in use of MDR reversal agents.4 We have previously reported that unnatural nine-membered ether 2a exhibits a good affinity for P-glycoprotein (Figure 1), because 2a forms 3D endo-cage structure similarly to paclitaxel (1), determined by conformational analysis based on MM2 calculation utilizing a Monte Carlo method.5 The compound 2a would be a good template to develop novel MDR reversal agents, and further modification of 2a such as introduction of the side chain of paclitaxel could be effective to increase the affinity for P-glycoprotein. We herein report the synthesis of C-aromataxane derivatives 3–6 consisting of the side chain of paclitaxel (1) and a new template 2b, and evaluation of their MDR reversal activity.
RESULTS AND DISCUSSION
In order to increase the affinity of 2a for P-glycoprotein, we planned to introduce the side chain moiety of paclitaxel (1) on the template 2a via condensation of N-benzoylphenylisoserine at the C2-hydroxy group or allylic oxidation at the C14 position, followed by the condensation with side chain of paclitaxel (1), leading to 3-6. (Figure 2).
The synthesis of derivatives 3-6 is summarized in Scheme 1. Toward the synthesis of 3, a carbonate group in (±)-2a was initially removed under basic condition to afford diol 7 in 96% yield. Selective acylation of the secondary alcohol with β-lactam (+)-8 using NaHMDS at –78 °C,6 followed by removal of the silyl group provided two diastereomers, which were readily separated by silica gel column chromatography to afford the less polar 3a (two steps 38%) and the polar 3b (two steps 31%), respectively.
The synthesis of 4 and 5 was performed as follows: face-selective allylic oxidation of 2a at the C14 position with TBHP,7 followed by reduction of the resulting peroxide furnished the alcohol 9 in 24% overall yield. Acylation of the resulting alcohol at the C14 position was performed according to the synthesis of 3, and removal of the silyl group with TBAF afforded 4ab as a 1:1 mixture of diastereomers. To prepare the benzoyl group at the C2 position, regioselective cleavage of the cyclic carbonate 9 with PhLi was performed to afford benzoate 10 in 96% yield. According to the synthesis of 4, coupling of 10 with β-lactam (+)-8 was performed to provide an inseparable 2.5:1 mixture of 5ab in 52% combined yield. In addition, the compound 6a and 6b were also prepared as follows: oxidation of the alcohol 10 at the C14 position, followed by stereoselective reduction of the resulting ketone from the convex face8 afforded the diastereomeric alcohol 11, whose stereochemistry was confirmed by nOe observation between H14 and C16 methyl group (Figure 3). Condensation of 11 with (+)-8 provided separable diastereomers 6a (27%) and 6b (36%), respectively.
It is known that P-glycoprotein consumes ATP to extrude the substrates to the outside of cells, thus we measured the compound-stimulated ATPase activity of P-glycoprotein by using plasma membranes from P-glycoprotein-overexpressing Sf9 cells to evaluate the affinity of the synthesized compounds 3-6 for P-glycoprotein.9 All derivatives showed saturation kinetics in the relationship between the compound-stimulated ATPase activity and the compound concentration, suggesting Michaelis-Menten kinetics. Thus, Km and Vmax values were calculated from Lineweaver-Burk plots of (compound concentration) x (compound-stimulated ATPase activity)–1 vs (compound concentration); these are summarized in Table 1. The values of Km and Vmax for each derivative are lower than those of the parent 2a, and the value of Vmax/Km for each derivative is higher than that of the parent 2a. In particular, 5ab showed the highest Vmax/Km value, suggesting that 5ab is the best substrate for P-glycoprotein among the synthetic derivatives shown in Table 1. Most of the derivatives exhibited more than one-twentieth weaker cytotoxicity against both KB-3-1 cells and MDR KB-G2 cells than paclitaxel did, except for 6a and 6b (IC50 8.0 µM and 8.5 µM against MDR KB-G2 cells, respectively).
Based on the above results, we chose four derivatives 3a, 3b, 4ab and 5ab as a candidate for P-glycoprotein-mediated MDR reversal agent, and evaluated their activities to reverse P-glycoprotein-mediated MDR by using MDR KB-G2 cells (Figure 4). It was found that cytotoxicity of paclitaxel (1) against MDR KB-G2 cells was enhanced by addition of the derivatives in a dose-dependant manner, therefore the derivatives could be a substrate for P-glycoprotein.
In particular, the sensitivity of MDR KB-G2 cells against paclitaxel (1) was significantly recovered in the presence of 5.0 µM 5ab, where IC50 value was diminished from 0.93 µM to 0.041 µM. In addition, it was found that the other derivatives 3a, 3b and 4ab also reversed the cytotoxicity of paclitaxel (1) against MDR KB-G2 cells. According to the above results, it should be noted that the derivative that possesses a similar structure to paclitaxel (1) would be suitable for the substrate for P-glycoprotein and be dominantly extruded to the outside of cells instead of paclitaxel (1).
With a good candidate 5ab for P-glycoprotein-mediated MDR reversal agent in hand, we next evaluated MDR reversal activity of the diastereomers 5a and 5b. The mixture of 5a and 5b was separated by preparative thin-layer chromatography, and the stereochemistry at the C14 position was determined by circular dichroism spectroscopy.10,11 As a result of ATPase assay, (14R)-5a showed greater Km and Vmax/Km values than (14S)-5b without showing cytotoxicity to KB-G2 cells (IC50 >100 µM) although (14S)-5b exhibited against KB-G2 cells (IC50 23 µM) (Table 2). In particular, cytotoxicity of paclitaxel (1) against MDR KB-G2 cells was considerably enhanced (IC50 30 nM) in the presence of 5.0 µM 5a, thereby (14R)-5a exhibited significant MDR reversal activity (Figure 5). The conformation of 5a would be important to be a substrate for a P-glycoprotein; stereochemistry of the C1 and C2 positions in 5a are identical to that in paclitaxel, thus stereochemistry at the C14 position would be crucial to be dominantly extruded to the outside of cells by P-glycoprotein.
In order to investigate the effect of the substituent on the C-ring, we next synthesized 2b which possesses a methoxymethyloxy (OMOM) group at the C7 position12 and evaluated the affinity for P-glycoprotein (Scheme 2). Coupling of aldehyde 12 with a vinyl anion 1313 proceeded at –78 °C to afford alcohol 14. Stereoselective epoxidation of 14, followed by reductive opening of the resulting epoxide 15 and selective protection of the primary alcohol with a TBS group provided diol 16 in moderate yield. After carbonate formation of 16 with 1,1-carbonyldiimidazole and removal of the TBS group, chlorination of the resulting alcohol 17 and subsequent removal of the PMB group with DDQ14 provided cyclization precursor 18. Intramolecular O-alkylation of 18 with NaH in the presence of 18-crown-6 proceeded at room temperature to afford the desired nine-membered ether 2b in 49% yield. The structure of 2b was determined by 1H and 13C NMR spectra. The affinity of 2b for P-glycoprotein was evaluated. Although it was found that the compound 2b exhibited similarly low cytotoxicity as 2a (data shown in supporting information), ATPase activity of P-glycoprotein in the presence of 100 µM 2b (11.8 nmol min–1 mg protein–1) was lower than that in the presence of 2a (41 nmol min–1 mg protein–1). Thus, the C5 position on the C-ring should be better than the C7 position for introduction of the substituents toward higher affinity for P-glycoprotein.
In summary, we have demonstrated the synthesis of C-aromataxane derivatives based on the parent 2a and evaluated their affinity for P-glycoprotein. The synthesis of derivatives 3–6 was readily performed by introduction of N-benzoylphenylisoserine to the C2 or C14 position by way of the modified Ojima method. According to P-glycoprotein assay, it was found that introduction of the N-benzoylphenylisoserine group to the parent 2a was effective to improve the affinity for P-glycoprotein. In particular, excellent Km and Vmax/Km of (14R)-5a values were observed without cytotoxicity to KB-G2 cells (IC50 >100 µM). It should be noted that (14R)-5a exhibited significant MDR reversal activity as cytotoxicity of paclitaxel (1) was considerably recovered (IC50 30 nM) in the presence of (14R)-5a at 5.0 µM. This observation suggested that both similar conformation to paclitaxel and stereochemistry at the C14 position are crucial to exhibit a good MDR reversal activity and to be extruded to the outside of cells instead of paclitaxel. Substituent effect in the affinity for P-glycoprotein was observed, and ATPase activity in the presence of 2a was greater than that of the new template 2b which possesses a OMOM group at the C7 position on the C-ring. The above observations would be useful to develop MDR reversal agents for the cancer therapy and further investigation for the synthesis and the evaluation of C-aromataxane derivatives is underway in our laboratory.
EXPERIMENTAL
General Techniques: NMR spectra were recorded on a JEOL Model EX-270 (270 MHz for 1H, 67.8 MHz for 13C) or a JEOL Model ECP-400 (400 MHz for 1H, 100 MHz for 13C) instrument in the indicated solvent. Chemical shifts are reported in units parts per million (ppm) relative to chloroform (7.26 ppm for 1H) or CDCl3 (77.1 ppm for 13C), and methanol (3.30 ppm for 1H) or CD3OD (49.8 ppm for 13C), CD2Cl2 (53.8 ppm for 13C) when internal standard is not indicated. Multiplicities are reported by using the following abbreviations: s; singlet, d; doublet, t; triplet, q; quartet, m; multiplet, br; broad, J; coupling constants in Hertz. IR spectra were recorded on Perkin Elmer Spectrum One FT-IR spectrophotometer. Only the strongest and/or structurally important peaks are reported as the IR data given in cm-1. All reactions were monitored by thin-layer chromatography carried out on 0.2 mm E. Merck silica gel plates (60F-254) with UV light, visualized by p-anisaldehyde solution. Merck silica gel or Chlomatorex NH-silica gel was used for column chromatography. Gel permeation chromatography (GPC) for qualitative and quantitative analysis were performed on a Japan Analytical Industry Model LC 605 (recycling preparative HPLC), on a Japan Analytical Industry Model RI-5 refractive index detector and on a Japan Analytical Industry Model 310 ultra violet detector with polystyrene gel column (JAIGEL-1H, 20 mm x 600 mm), using chloroform as a solvent (3.5 mL/min). High-resolution mass spectra were measured with JEOL MS-AX500 (FAB) or P. E. Biosystems TK-3500 Biospectrometry Workstation (ESI-TOF) instruments.
1,2-Diol 7
To a solution of the 9-membered ether 2a5 (49.7 mg, 0.133 mmol) in THF (0.5 mL) was added KOH (0.800 M in MeOH, 5.0 mL) at 0 ˚C. After being stirred at 40 ˚C for 1 h, the reaction mixture was quenched with 1 M aqueous HCl at 0 ˚C. The organic layer was separated and the aqueous layer was extracted twice with EtOAc. The combined organic layers were washed with saturated aqueous NaHCO3 and brine, dried over Na2SO4 and concentrated in vacuo. The residue was purified by column chromatography on silica gel (35% EtOAc in hexane) to afford the 1,2-diol 7 (44.5 mg, 0.128 mmol, 96%) as a colorless oil.
1H NMR (400 MHz, CDCl3) δ 7.40 (d, J = 2.4 Hz, 1H), 7.23 (d, J = 8.2 Hz, 1H), 6.84 (dd, J = 2.4, 8.2 Hz, 1H), 5.22 (d, J = 6.8 Hz, 1H), 5.22 (s, 1H), 5.14 (d, J = 6.8 Hz, 1H), 4.69 (d, J = 13.0 Hz, 1H) 4.47 (d, J = 13.0 Hz, 1H), 4.31 (s, 2H), 3.47 (s, 3H), 3.13 (s, 1H, OH), 2.64 (s, 1H, OH), 2.30 (m, 1H), 1.97 (m, 1H), 1.67 (m, 1H), 1.49 (s, 3H), 1.10 (m, 1H), 1.01 (s, 3H), 0.96 (s, 3H); 13C NMR (67.8 MHz, CDCl3) δ 155.8, 142.5, 139.7, 132.8, 132.2, 130.7, 114.9, 114.2, 94.4, 78.7, 69.6, 67.6, 64.9, 56.0, 40.8, 29.5, 28.3, 26.2, 21.5, 20.3; FT-IR (Neat) 3402, 2952, 1606, 1493, 1461, 1375, 1234, 1153, 1018, 802 cm–1; HRMS (ESI-TOF) calcd for C20H29O5 [M+H]+ 349.2015, found 349.2015.
(1R*, 2R*, 14S*)-Carbonate 9
To a solution of the 9-membered ether 2a (260 mg, 0.695 mmol) in toluene (7.0 mL) was added t-butyl hydroperoxide (5.0 mL, 70 wt % in water) at 0 ˚C. After being stirred at 60 ˚C for 36 h, the reaction mixture was quenched with brine at 0 ˚C. The organic layer was separated and the aqueous layer was extracted twice with EtOAc. The combined organic layers were dried over MgSO4 and concentrated in vacuo. The residue was purified by short-pass column chromatography on silica gel (35~40% EtOAc in hexane) to afford the hydroperoxide (78.3 mg), and the above product was subsequently used for next reaction.
To a solution of the hydroperoxide (78.3 mg) in dry toluene (4.5 mL) was added trimethylphosphine (0.33 mL, 1.00 M in toluene, 0.33 mmol, 1.7 equiv) at 0 ˚C under argon. After being stirred at 0 ˚C for 15 min, the reaction mixture was concentrated in vacuo. The residue was purified by column chromatography on silica gel (2% MeOH in CHCl3) to afford the carbonate 9 (60.5 mg, 0.155 mmol, 24% in 2 steps) as a colorless oil.
1H NMR (400 MHz, CDCl3) δ 7.21 (d, J = 2.9 Hz, 1H), 7.16 (d, J = 8.2 Hz, 1H), 6.95 (dd, J = 2.9, 8.2 Hz, 1H), 6.23 (s, 1H), 5.24 (d, J = 6.8 Hz, 1H), 5.11 (d, J = 6.8 Hz, 1H), 4.78 (d, J = 15.0 Hz, 1H), 4.50 (d, J = 15.0 Hz, 1H), 4.36 (d, J = 13.0 Hz, 1H), 4.27 (d, J = 13.0 Hz, 1H), 3.47 (s, 3H), 2.65-2.75 (m, 2H), 2.22 (dd, J = 3.9, 13.5 Hz, 1H), 1.78 (d, J = 4.4 Hz, 1H, OH), 1.55 (s, 3H), 1.32 (s, 3H), 1.29 (s, 3H); 13C NMR (67.8 MHz, CDCl3) δ 156.1, 153.6, 139.6, 135.4, 133.5, 131.8, 130.7, 116.5, 114.3, 94.5, 93.0, 77.6, 71.2, 68.3, 66.5, 56.2, 40.0, 34.7, 30.6, 20.7, 18.3; FT-IR (Neat) 3399, 2927, 1801, 1609, 1502, 1436, 1262, 1208, 1153, 1039, 804, 755 cm–1; HRMS (ESI-TOF) calcd for C21H27O7 [M+H]+ 391.1757, found 391.1755.
Benzoate 10
To a solution of the carbonate 9 (9.6 mg, 25 µmol) in dry THF (1.0 mL) was added PhLi (0.98 M in ether, 0.13 mL, 0.12 mmol, 5.0 equiv) at –78 ˚C under argon. After being stirred at –78 ˚C for 35 min, the reaction mixture was quenched with saturated aqueous NH4Cl at 0 ˚C. The organic layer was separated and the aqueous layer was extracted twice with EtOAc. The combined organic layers were washed with saturated aqueous NaHCO3 and brine, dried over Na2SO4 and concentrated in vacuo. The residue was purified by column chromatography on silica gel (5% MeOH in CHCl3) to afford benzoate 10 (11.1 mg, 23.7 µmol, 96%).
1H NMR (400 MHz, CDCl3) δ 8.08 (d, J = 7.3 Hz, 2H), 7.59 (t, J = 7.3 Hz, 1H), 7.47 (t, J = 7.3 Hz, 2H) , 7.29 (d, J = 8.7 Hz, 1H), 7.27 (d, J = 2.4 Hz, 1H), 6.87 (dd, J = 2.4, 8.7 Hz, 1H), 6.64 (s, 1H), 5.12 (s, 2H), 4.85 (d, J = 13.5 Hz, 1H), 4.57 (d, J = 13.5 Hz, 1H), 4.46 (d, J = 12.6 Hz, 1H), 4.35 (d, J = 12.6 Hz, 1H), 3.40 (s, 3H), 3.15 (dd, J = 4.8, 9.7 Hz 1H), 2.86 (dd, J = 9.7, 14.5 Hz 1H), 2.66 (brs, 1H, OH), 2.12 (dd, J = 4.8, 14.5 Hz 1H), 1.62 (s, 3H), 1.23 (s, 3H), 1.17 (s, 3H, e); 13C NMR (67.8 MHz, CDCl3) δ 165.7, 156.2, 139.8, 139.5, 135.9, 133.5, 132.8, 132.6, 129.9, 129.7, 128.7, 115.4, 114.1, 94.6, 80.9, 73.1, 69.3, 68.0, 65.1, 55.9, 41.6, 38.3, 31.2, 21.6, 18.1; FT-IR (Neat) 3471, 2947, 1716, 1607, 1499, 1452, 1275, 1153, 1071, 1026, 713 cm–1; HRMS (ESI-TOF) calcd for C27H33O7 [M+H]+ 469.2226, found 469.2226.
(1R*, 2R*, 14R*)-Diol 11
To a solution of the allylic alcohol 10 (7.2 mg, 15.4 µmol) and NMO (9.0 mg, 77 µmol, 5.0 equiv.) in CH2Cl2 (2.0 mL) was added a catalytic amount of TPAP at 0 ˚C under argon. After being stirred for 36 h, the reaction mixture was diluted with hexane (15 mL) and EtOAc (15 mL) and stirred with Florisil® at room temperature for 4 h. The suspension was filtered through a pad of Celite® and the filtrate was concentrated in vacuo. The residue was purified by column chromatography on silica gel (40-50% EtOAc in hexane) to afford the ketone (7.1 mg, 15 µmol, 99%) as a colorless oil.
1H NMR (400 MHz, CDCl3) δ 8.08 (d, J = 7.3 Hz, 2H), 7.61 (t, J = 7.3 Hz, 1H), 7.48 (t, J = 7.3 Hz, 2H), 7.24 (d, J = 8.2 Hz, 1H), 7.19 (d, J = 2.4 Hz, 1H), 6.80 (dd, J = 2.4, 8.2 Hz, 1H), 6.73 (s, 1H), 5.15 (d, J = 7.3 Hz, 1H), 5.04 (d, J = 7.3 Hz, 1H), 5.01 (d, J = 13.5 Hz, 1H), 4.64 (d, J = 13.5 Hz, 1H), 4.62 (d, J = 13.1 Hz, 1H), 4.44 (d, J = 13.1 Hz, 1H), 3.38 (s, 3H), 3.27 (d, J = 18.9 Hz, 1H), 2.87 (d, J = 18.9 Hz, 1H), 2.80 (brs, 1H, OH), 1.62 (s, 3H), 1.23 (s, 3H), 1.17 (s, 3H, d); 13C NMR (67.8 MHz, CDCl3) δ 197.7, 165.9, 156.9, 153.0, 140.6, 138.8, 133.7, 132.9, 130.7, 129.8, 129.6, 128.7, 115.8, 114.2, 94.4, 78.2, 73.0, 69.4, 65.5, 55.8, 43.9, 43.1, 31.3, 21.2, 12.1; FT-IR (Neat) 3504, 2989, 1718, 1671, 1607, 1498, 1451, 1359, 1269, 1215, 1151, 757 cm–1; HRMS (ESI-TOF) calcd for C27H31O7 [M+H]+ 467.2070, found 467.2078.
To a solution of the above ketone (7.30 mg, 15.6 µmol) in MeOH (3.0 mL) and THF (0.50 mL) was added CeCl3 (400 mg, large excess) at 0 ˚C under argon. After being stirred at 0 ˚C for 15 min, the mixture was treated with NaBH4 (7.49 mg, 0.198 mmol, 10 equiv) in eight portions for 30 min at room temperature. The reaction mixture was quenched with saturated aqueous NH4Cl at 0 ˚C, and the aqueous layer was extracted twice with EtOAc. The combined organic layers were washed with saturated aqueous NaHCO3 and brine, dried over MgSO4 and concentrated in vacuo. The residue was purified by column chromatography on silica gel (35% EtOAc in hexane) to afford the diol 11 (4.00 mg, 8.54 µmol, 55 %).
1H NMR (400 MHz, CDCl3) δ 8.01 (d, J = 7.3 Hz, 2H), 7.53 (t, J = 7.3 Hz, 1H), 7.41 (t, J = 7.3 Hz, 2H), 7.29 (d, J = 8.2 Hz, 1H), 7.19 (d, J = 2.4 Hz, 1H), 6.85 (dd, J = 2.4, 8.2 Hz, 1H), 6.58 (s, 1H), 5.09 (d, J = 7.3 Hz, 1H), 5.06 (d, J = 7.3 Hz, 1H), 4.78 (d, J = 13.5 Hz, 1H), 4.51 (d, J = 13.5 Hz, 1H), 4.36 (d, J = 12.6 Hz, 1H), 4.27 (d, J = 12.6 Hz, 1H), 4.08 (m, 1H), 3.36 (s, 3H), 2.56 (dd, J = 10.6, 15.5 Hz, 1H), 2.56 (brs, 1H, OH), 2.39 (dd, J = 2.9, 15.5 Hz, 1H), 1.55 (s, 3H), 1.11 (s, 3H), 0.94 (s, 3H); 13C NMR (67.8 MHz, CDCl3) δ 165.7, 155.8, 142.9, 141.4, 133.6, 133.3, 131.6, 129.9, 129.7, 128.7, 116.1, 113.3, 94.3, 76.9, 73.5, 67.9, 67.1, 65.4, 56.1, 42.2, 40.1, 29.8, 26.8, 21.8, 15.2; FT-IR (Neat) 3546, 2928, 1719, 1605, 1498, 1451, 1272, 1153, 1069, 1025, 753, 711 cm–1; HRMS (ESI-TOF) calcd for C27H33O7 [M+H]+ 469.2226, found 469.2227.
General Procedure for acylation with N-benzoyl-β-phenylisoserine side chain.
To a mixture of the alcohol and β-lactam (+)-8 in THF was added sodium hexamethyldisilazide (1.0 M in THF) at –78 ˚C under argon. After being stirred at –78 ˚C, the reaction mixture was quenched with saturated aqueous NH4Cl at 0 ˚C. The organic layer was separated and the aqueous layer was extracted twice with EtOAc. The combined organic layers were washed with saturated aqueous NaHCO3 and brine, dried over MgSO4. After removal of the solvent in vacuo, the crude mixture of diastereomers was used for the next reaction without further purification.
To a solution of the mixture in THF was added TBAF (1.0 M in THF) at 0 ˚C under argon. After being stirred at 0 ˚C, the reaction mixture was quenched with saturated aqueous NaHCO3 at 0 ˚C. The organic layer was separated and the aqueous layer was extracted twice with EtOAc. The combined organic layers were washed with brine, dried over MgSO4 and concentrated in vacuo. The resulting residue was purified by preparative TLC or column chromatography on silica gel (eluted with EtOAc in hexane) to afford the coupling product.
3a and 3b
Condition: 1) substrate 7 (25.0 mg, 71.8 µmol), sodium hexamethyldisilazide (180 µL, 0.178 mmol, 2.50 equiv), β-lactam (+)-8 (54.1 mg, 0.128 mmol, 1.78 equiv), THF (1.0 mL), –78 °C, 30 min; 2) TBAF (0.72 mL, 0.72 mmol, 10.0 equiv), THF (2.0 mL), 0 °C, 30 min; Purification: preparative TLC (50% EtOAc in hexane). The absolute configuration of two diastereomers was not determined.
3a (less polar diastereomer) 38 % (8.5 mg, 14 µmol)
1H NMR (400 MHz, CDCl3) δ 7.78 (d, J = 7.3 Hz, 2H), 7.56 (d, J = 7.3 Hz, 2H), 7.53 (t, J = 7.3 Hz, 1H), 7.45 (t, J = 7.3 Hz, 2H), 7.41 (t, J = 7.3 Hz, 2H), 7.34 (t, J = 7.3 Hz, 1H), 7.23 (d, J = 8.2 Hz, 1H), 7.10 (d, J = 2.4 Hz, 1H), 6.96 (d, J = 9.7 Hz, 1H), 6.85 (dd, J = 2.4, 8.2 Hz, 1H), 6.42 (s, 1H), 6.05 (dd, J = 1.5, 9.7 Hz, 1H), 5.18 (d, J = 6.8 Hz, 1H), 5.14 (d, J = 6.8 Hz, 1H), 4.83 (d, J = 13.0 Hz, 1H), 4.66 (d, J = 1.5 Hz, 1H, NH), 4.51 (d, J = 13.0 Hz, 1H), 4.33 (s, 2H), 3.45 (s, 3H), 3.26 (brs, 1H, OH), 3.05 (brs, 1H, OH), 2.39 (m, 1H), 1.93-2.09 (m, 2H), 1.55 (s, 3H), 1.19 (m, 1H), 1.05 (s, 3H), 0.96 (s, 3H, e); 13C NMR (67.8 MHz, CDCl3) δ 172.3, 167.8, 155.8, 140.2, 139.5, 138.7, 133.8, 132.6, 132.2, 132.1, 131.0, 128.9, 128.7, 128.2, 127.3, 15.0, 113.9, 94.5, 75.2, 72.9, 68.1, 65.2, 56.0, 54.5, 41.8, 29.8, 29.4, 28.1, 26.6, 21.3, 20.5; FT-IR (Neat) 3420, 2955, 1743, 1652, 1521, 1488, 1237, 1152, 1077, 1020, 700 cm–1; HRMS (ESI-TOF) calcd for C36H42NO8 [M+H]+ 616.2910, found 616.2908.
3b (polar diastereomer) 31% (6.9 mg, 11 µmol)
1H NMR (400 MHz, CDCl3) δ 7.57 (d, J = 7.3 Hz, 2H), 7.46 (t, J = 7.3 Hz, 1H), 7.42 (d, J = 7.3 Hz, 2H), 7.35 (t, J = 7.3 Hz, 4H), 7.31 (t, J = 7.3 Hz, 1H), 7.15 (d, J = 8.2 Hz, 1H), 7.11 (d, J = 2.4 Hz, 1H), 6.96 (d, J = 8.2 Hz, 1H), 6.72 (dd, J = 2.4, 8.2 Hz, 1H), 6.47 (s, 1H, f), 5.62 (dd, J = 2.4, 8.2 Hz, 1H), 5.08 (d, J = 6.8 Hz, 1H), 4.95 (d, J = 6.8 Hz, 1H), 4.81 (d, J = 13.5 Hz, 1H), 4.72 (d, J = 2.4 Hz, 1H, NH), 4.48 (d, J = 13.5 Hz, 1H), 4.31 (d, J = 13.0 Hz, 1H), 4.28 (d, J = 13.0 Hz, 1H), 3.66 (brs, 1H, OH), 3.37 (s, 3H), 2.44 (brs, 1H, OH), 2.26 (m, 1H), 2.00 (m, 1H), 1.83 (m, 1H), 1.49 (s, 3H), 1.15 (m, 1H), 1.03 (s, 3H), 1.00 (s, 3H); 13C NMR (67.8 MHz, CDCl3) δ 172.0, 167.3, 155.8, 139.4, 139.1,138.6, 134.1, 132.1, 132.0, 131.7, 130.7, 128.8, 128.5, 128.1, 127.2, 127.0, 115.3, 113.6, 94.1, 75.0, 73.8, 68.4, 65.0, 55.9, 55.7, 41.3, 29.8, 29.3, 27.9, 27.0, 21.3, 20.1; FT-IR (Neat) 3374, 2962, 1733, 1646, 1521, 1489, 1216, 1152, 1076, 1027, 943, 755 cm–1; HRMS (ESI-TOF) calcd for C36H42NO8 [M+H]+ 616.2910, found 616.2917.
4ab
Conditions: 1) Substrate 9 (7.6 mg, 20 µmol), sodium hexamethyldisilazide (49 µL, 49 µmol, 2.5 equiv), β-lactam (+)-8 (14.8 mg, 35.0 µmol, 1.79 equiv), THF (0.50 mL), –78 °C, 30 min; 2) TBAF (0.11 mL, 0.11 mmol, 10 equiv), THF (1.00 mL), 0 °C, 15 min; Purification: preparative TLC (50% EtOAc in hexane); 4.4 mg, 6.7 µmol, 52% in 2 steps.
1H NMR (400 MHz, CDCl3, a mixture of 1:1 diastereomers)
δ 7.77 (d, J = 7.3 Hz, 2H), 7.68 (d, J = 7.3 Hz, 2H), 7.24-7.67 (m, 19H), 7.17 (t, J = 7.3 Hz, 1H), 7.16 (d, J = 8.2 Hz, 1H), 7.10 (d, J = 8.2 Hz, 1H), 6.96 (dd, J = 2.4, 8.2 Hz, 1H), 6.92 (dd, J = 2.4, 8.2 Hz, 1H), 6.90 (d, J = 9.2 Hz, 1H, m), 6.86 (d, J = 9.2 Hz, 1H, m), 6.24 (s, 1H), 6.19 (s, 1H), 5.69 (dd, J = 2.9, 9.2 Hz, 1H), 5.58 (dd, J = 1.9, 9.2 Hz, 1H), 5.21 (m, 2H), 5.17 (d, J = 7.3 Hz, 1H), 5.13 (d, J = 6.8 Hz, 1H), 4.82 (d, J = 15.0 Hz, 1H), 4.78 (d, J = 15.0 Hz, 1H), 4.56 (brs, 2H, NH), 4.51 (d, J = 15.0 Hz, 1H), 4.48 (d, J = 15.0 Hz, 1H), 4.37 (d, J = 13.0 Hz, 1H), 4.36 (d, J = 13.0 Hz, 1H), 4.28 (d, J = 13.0 Hz, 1H), 4.25 (d, J = 13.0 Hz, 1H), 3.85 (dd, J = 4.83, 9.67 Hz, 1H), 3.80 (dd, J = 4.8, 9.7 Hz, 1H), 3.45 (s, 3H), 3.42 (s, 3H), 3.28 (brs, 1H, OH), 3.21 (brs, 1H, OH), 2.90 (dd, J = 9.7, 15.0 Hz, 1H), 2.74 (dd, J = 9.7, 15.0 Hz, 1H), 2.11 (dd, J = 4.8, 15.0 Hz, 1H), 2.06 (dd, J = 4.8, 15.0 Hz, 1H), 1.56 (s, 3H), 1.56 (s, 3H), 1.33 (s, 3H), 1.28 (s, 3H), 1.16 (s, 3H), 1.14 (s, 3H); HRMS (ESI-TOF) calcd for C37H40NO10 [M+H]+ 658.2652, found 658.2654.
5a and 5b
Condition: 1) Substrate 10 (33.0 mg, 70.4 µmol), sodium hexamethyldisilazide (0.18 mL, 0.18 mmol, 2.6 equiv), β-lactam (+)-8 (57.3 mg, 0.135 mmol, 1.92 equiv.), THF (3.00 mL, 42.6 mL/mmol), –78 °C, 30 min; 2) TBAF (46 µL, 46 µmol, 1.1 equiv.), THF (2.0 mL), 0 °C, 10 min; Purification: column chromatography on silica gel (40-50% EtOAc in hexane)
5a (less polar diastereomer): 35% (18.0 mg, 24.5 µmol)
1H NMR (400 MHz, CDCl3) δ 8.04 (d, J = 7.3 Hz, 2H), 7.79 (d, J = 7.3 Hz, 2H), 7.59 (t, J = 7.3 Hz, 1H), 7.53 (t, J = 7.3 Hz, 1H), 7.45 (t, J = 7.3 Hz, 2H), 7.43 (t, J = 7.3 Hz, 2H), 7.42 (d, J = 7.3 Hz, 2H), 7.38 (d, J = 2.9 Hz, 1H), 7.34 (t, J = 7.3 Hz, 2H), 7.29 (t, J = 7.3 Hz, 1H), 7.28 (d, J = 8.7 Hz, 1H), 6.94 (d, J = 9.2 Hz, 1H), 6.86 (dd, J = 2.9, 8.7 Hz, 1H), 6.62 (s, 1H), 5.69 (dd, J = 1.9, 9.2 Hz, 1H), 5.23 (d, J = 6.8 Hz, 1H), 5.09 (d, J = 6.8 Hz, 1H), 4.85 (d, J = 13.5 Hz, 1H), 4.59 (d, J = 13.5 Hz, 1H), 4.58 (d, J = 1.9 Hz, 1H, NH), 4.48 (d, J = 12.6 Hz, 1H), 4.36 (d, J = 12.6 Hz, 1H), 4.33 (dd, J = 4.8, 9.7 Hz, 1H), 3.38 (s, 3H, k), 3.25 (brs, 1H, OH), 2.90 (dd, J = 9.7, 14.5 Hz, 1H), 2.02 (dd, J = 4.8, 14.5 Hz, 1H), 1.63 (s, 3H), 1.26 (s, 3H), 1.03 (s, 3H); 13C NMR (100 MHz, CD2Cl2) δ 172.9, 166.8, 165.3, 156.7, 140.1, 140.0, 138.9, 134.7, 134.3, 133.4, 132.7, 132.5, 131.8, 129.9, 129.6, 128.7, 128.6, 127.9, 127.1, 126.9, 115.8, 113.7, 94.7, 80.2, 74.2, 73.5, 72.7, 68.2, 64.8, 55.7, 54.9, 53.9, 41.5, 35.2, 30.5, 21.3, 17.7; FT-IR (Solid) 3430, 2929, 1732, 1651, 1604, 1487, 1452, 1273, 1152, 1070, 967, 753, 712 cm–1; HRMS (ESI-TOF) calcd for C43H46NO10 [M+H]+ 736.3122, found 736.3121.
5b (polar diastereomer): 36% (14.0 mg, 25.3 µmol)
1H NMR (400 MHz, CDCl3) δ 8.01 (d, J = 7.3 Hz, 2H), 7.63 (d, J = 7.3 Hz, 2H), 7.53 (t, J = 7.3 Hz, 1H), 7.44 (t, J = 7.3 Hz, 1H), 7.40 (t, J = 7.3 Hz, 2H), 7.36 (t, J = 7.3 Hz, 2H), 7.33 (d, J = 6.8 Hz, 2H), 7.33 (d, J = 2.4 Hz, 1H), 7.26 (t, J = 6.8 Hz, 2H), 7.21 (t, J = 6.8 Hz, 1H), 7.17 (d, J = 8.7 Hz, 1H), 6.87 (d, J = 9.2 Hz, 1H), 6.75 (dd, J = 2.4, 8.7 Hz, 1H), 6.55 (s, 1H), 5.54 (dd, J = 1.9, 9.2 Hz, 1H, q), 5.12 (d, J = 7.3 Hz, 1H), 4.99 (d, J = 7.3 Hz, 1H), 4.79 (d, J = 13.5 Hz, 1H), 4.50 (d, J = 13.5 Hz, 1H), 4.49 (d, J = 1.9 Hz, 1H, NH), 4.38 (d, J = 12.6 Hz, 1H), 4.28 (d, J = 12.6 Hz, 1H), 4.26 (dd, J = 4.8, 10.1 Hz, 1H), 3.24 (s, 3H), 3.15 (brs, 1H, OH), 2.99 (dd, J = 10.1, 15.0 Hz, 1H), 2.63 (brs, 1H, OH), 1.91 (dd, J = 4.8, 15.0 Hz, 1H), 1.55 (s, 3H), 1.12 (s, 3H), 0.93 (s, 3H); 13C NMR (100 MHz, CD2Cl2) δ 172.7, 166.5, 165.4,156.7, 139.9, 139.9, 139.0, 134.8, 134.2, 133.5, 132.6,132.3, 131.8, 129.9, 129.7, 128.6, 127.9, 127.0, 126.8, 115.9, 113.5, 94.6, 80.3, 74.3, 73.8, 72.8, 68.3, 64.9, 55.6, 54.9, 41.5, 35.8, 30.2, 21.2, 17.6; FT-IR (Solid) 3430, 2957, 1725, 1655, 1604, 1486, 1451, 1271, 1108, 1071, 1026, 754, 711 cm–1; HRMS (ESI-TOF) calcd for C43H46NO10 [M+H]+ 736.3122, found 736.3120.
6a and 6b
Condition : 1) substrate 11 (6.6 mg, 14 µmol), sodium hexamethyldisilazide (0.15 mL, 0.15 mmol, 10 equiv), β-lactam (+)-8 (23 mg, 55 µmol, 3.8 equiv), THF (1.5 mL), –78 °C, 30 min; 2) TBAF (43 µL, 43 µmol, 5.0 equiv), THF (2.0 mL), 0 °C, 15 min; Purification : preparative TLC (50% EtOAc in hexane). The absolute configuration of two diastereomers was not determined.
6a (less polar diastereomer): 27% (2.8 mg, 3.8 µmol)
1H NMR (400 MHz, CDCl3) δ 8.28 (d, J = 7.3 Hz, 2H), 7.85 (d, J = 7.3 Hz, 2H), 7.52-7.61 (m, 5H), 7.49 (t, J = 7.3 Hz, 2H), 7.47 (t, J = 7.3 Hz, 2H), 7.39 (t, J = 7.3 Hz, 2H), 7.31 (t, J = 2.9 Hz, 1H), 7.28 (d, J = 8.2 Hz, 1H), 6.99 (d, J = 9.7 Hz, 1H), 6.90 (dd, J = 2.4, 8.2 Hz, 1H), 6.59 (s, 1H), 6.05 (dd, J = 1.45, 9.7 Hz, 1H), 5.64 (dd, J = 3.4, 11.1 Hz, 1H), 4.89 (d, J = 13.0 Hz, 1H), 4.84 (d, J = 6.8 Hz, 1H), 4.68 (d, J = 6.8 Hz, 1H), 4.68 (d, J = 1.5 Hz, 1H, NH), 4.59 (d, J = 13.0 Hz, 1H), 4.41 (d, J = 12.6 Hz, 1H), 4.29 (d, J = 12.6 Hz, 1H), 3.31 (s, 1H, OH), 3.11 (s, 3H), 2.73 (dd, J = 3.4, 15.5 Hz, 1H), 2.67 (s, 1H, OH), 2.41 (dd, J = 11.1, 15.5 Hz, 1H), 1.63 (s, 3H), 1.03 (s, 3H), 0.91 (s, 3H); 13C NMR (67.8 MHz, CDCl3) δ 172.8, 166.8, 165.7, 156.4, 140.7, 139.0, 137.6, 134.4, 133.4, 132.9, 132.8, 132.7, 131.9, 130.1, 129.9, 128.8, 128.7, 127.8, 127.3, 127.1, 117.7, 115.7, 95.3, 76.3, 73.7, 72.9, 71.1, 68.0, 64.8, 55.9, 54.3, 42.2, 35.5, 29.8, 26.1, 22.0, 14.6; FT-IR (Solid) 3429, 2927, 1726, 1661, 1520, 1488, 1452, 1271, 1101, 1073, 1026, 758, 713 cm–1; HRMS (ESI-TOF) calcd for C43H46NO10 [M+H]+ 736.3122, found 736.3123.
6b (polar diastereomer): 15% (1.2 mg, 2.1 µmol)
1H NMR (400 MHz, CDCl3) δ 8.06 (d, J = 7.3 Hz, 2H), 7.74 (d, J = 7.3 Hz, 2H), 7.59 (t, J = 7.3 Hz, 1H), 7.38-7.50 (m, 9H), 7.34 (t, J = 7.3 Hz, 1H), 7.33 (t, J = 8.2 Hz, 1H), 7.07 (d, J = 2.4 Hz, 1H), 6.94 (d, J = 9.2 Hz, 1H), 6.89 (dd, J = 2.4, 8.2 Hz, 1H), 6.64 (s, 1H), 5.44 (dd, J = 1.9, 9.2 Hz, 1H), 5.37 (dd, J = 2.9, 10.6 Hz, 1H), 4.93 (d, J = 6.8 Hz, 1H), 4.85 (d, J = 14.0 Hz, 1H), 4.75 (d, J = 6.77 Hz, 1H), 4.66 (d, J = 1.93 Hz, 1H, NH), 4.59 (d, J = 14.0 Hz, 1H), 4.42 (d, J = 13.0 Hz, 1H), 4.33 (d, J = 13.0 Hz, 1H), 3.35 (brs, 1H, OH), 3.19 (s, 3H), 2.69 (brs, 1H, OH), 2.66 (dd, J = 10.6, 15.5 Hz, 1H), 2.40 (dd, J = 2.9, 15.5 Hz, 1H), 1.63 (s, 3H), 1.18 (s, 3H), 1.03 (s, 3H); 13C NMR (67.8 MHz, CDCl3) δ 172.6, 166.7, 165.7, 155.8, 140.4, 139.1, 137.6, 134.2, 133.7, 133.6, 132.8, 132.7, 131.8, 129.8, 129.7, 128.9, 128.7, 128.1, 127.2, 127.1, 116.7, 115.1, 94.9, 76.5, 73.3, 73.0, 71.9, 68.4, 65.2, 56.1, 55.1, 42.2, 37.0, 29.8, 26.7, 21.7, 15.5; FT-IR (Solid) 3436, 2926, 1726, 1661, 1515, 1486, 1273, 1111, 1071, 1026, 755, 712 cm–1; HRMS (ESI-TOF) calcd for C43H46NO10 [M+H]+ 736.3122, found 736.3118.
Alcohol 14
To a solution of vinyllithium 13 in dry THF (11 mL) was added a solution of benzaldehyde 12 (300 mg, 0.948 mmol) in dry THF (6.6 mL) via cannula at −78 °C under argon. After being stirred at −78 °C for 4.5 h, the reaction mixture was quenched with saturated aqueous NH4Cl at −78 °C under argon atmosphere. The organic layer was separated and the aqueous layer was extracted twice with Et2O. The combined organic layers were washed with saturated aqueous NaHCO3 and brine, and dried over MgSO4. The filtrate was concentrated in vacuo and the resulting residue was purified by flash column chromatography on silica gel (10% EtOAc in hexane) to afford the allylic alcohol 14 (381 mg, 6.54 mmol, 63%) as a yellow oil.
1H NMR (400 MHz, CDCl3) δ 7.30 (d, J = 8.7 Hz, 2H), 7.13 (d, J = 4.6 Hz, 1H), 7.12 (d, J = 4.6 Hz, 1H), 7.03 (t, J = 4.6 Hz, 1H), 6.86 (d, J = 8.7 Hz, 2H), 6.00 (t, J = 3.2 Hz, 1H), 5.58 (brs, 1H), 5.18 (s, 2H), 4.99 (d, J = 10.5 Hz, 1H), 4.66 (d, J = 10.5 Hz, 1H), 4.60 (d, J = 11.4 Hz, 1H), 4.54 (d, J = 11.4 Hz, 1H), 4.18 (d, J = 11.0 Hz, 1H), 4.13 (d, J = 11.0 Hz, 1H), 3.79 (s, 3H), 3.47 (s, 3H), 3.38 (d, J = 2.7 Hz, 1H), 2.78 (d, J = 3.2 Hz, 2H), 1.78 (s, 3H), 1.22 (s, 3H), 0.88 (s, 9H), 0.77 (3H, s), 0.06 (3H, s), 0.05 (3H, s); 13C NMR (100 MHz, CDCl3) δ 159.4, 155.9, 145.6, 143.4, 135.5, 129.9, 129.7, 129.5, 129.3, 129.2, 124.6, 121.7, 121.3, 113.96, 113.85, 95.2, 72.4, 68.9, 62.4, 58.9, 56.2, 55.3, 38.2, 33.5, 28.3, 26.0, 25.9, 19.2, 18.4, –5.3, –5.4; IR (Neat) 3449, 2955, 2930, 2856, 1612, 1585, 1514, 1466, 1250, 837 cm–1; HRMS (ESI-TOF) calcd for C34H50O6SiNa [M+Na]+ 605.3248, found 605.3269.
Epoxide 15
To a solution of the allylic alcohol 14 (300 mg, 0.52 mmol) in dry benzene (5.2 mL) was added TBHP (~5.5 M in decane solution, 0.18 mL, 0.98 mmol, 1.9 equiv) and VO(acac)2 (6.84 mg, 26 µmol, 0.05 equiv) at 0 °C under argon. After being stirred at room temperature for 1.5 h, the reaction mixture was quenched with 10% aqueous Na2S2O3 at 0 °C. The organic layer was separated and the aqueous layer was extracted twice with EtOAc. The combined organic layers were washed with saturated aqueous NaHCO3 and brine, and dried over Na2SO4. The filtrate was concentrated in vacuo and the resulting residue was purified by column chromatography on silica gel (25% EtOAc in hexane) to afford the epoxide 15 (239 mg, 0.399 mmol, 77%) as a yellow oil.
1H NMR (400 MHz, CDCl3) δ 7.29 (d, J = 8.8 Hz, 2H), 7.23 (t, J = 8.0 Hz, 1H), 7.05 (dd, J = 0.7, 8.0 Hz, 1H), 7.02 (dd, J = 0.7, 8.0 Hz, 1H), 6.86 (d, J = 8.8 Hz, 2H), 5.56 (s, 1H), 5.20 (d, J = 6.6 Hz, 1H), 5.14 (d, J = 6.6 Hz, 1H), 4.81 (d, J = 10.6 Hz, 1H), 4.78 (d, J = 10.6 Hz, 1H), 4.56 (d, J = 11.3 Hz, 1H), 4.51 (d, J = 11.3 Hz, 1H), 4.10 (d, J = 11.4 Hz, 1H), 4.06 (d, J = 11.4 Hz, 1H), 3.79 (s, 3H), 3.46 (s, 3H), 3.40 (s, 1H), 2.88 (brs, 1H), 2.51 (s, 2H), 1.69 (s, 3H), 1.30 (s, 3H), 0.89 (s, 3H), 0.88 (s, 9H), 0.05 (s, 6H); 13C NMR (100 MHz, CDCl3) δ 159.1, 156.0, 142.2, 134.0, 129.4, 129.0, 125.5, 125.1, 120.8, 114.2, 113.6, 94.9, 72.2, 66.3, 66.0, 62.2, 58.9, 57.7, 56.2, 55.3, 38.6, 31.8, 26.0, 22.2, 19.8, 18.4, –5.3, –5.4; IR (Neat) 3472, 2953, 2930, 1614, 1587, 1513, 1470, 1250, 837 cm–1; HRMS (FAB) calcd for C34H50O7SiNa [M+Na]+ 621.3223, found 621.3225.
Diol 16
To a solution of epoxide 15 (500 mg, 0.84 mmol) in dry Et2O (8.4 mL) was added a solution of LiAlH4 (ca. 0.35 M solution in dry Et2O, 29 mL, 10 mmol, 12.0 equiv) dropwise at 0 °C under argon. After being stirred at reflux for 5 h, the reaction mixture was diluted with Et2O, quenched with saturated aqueous Na2SO4 and dissolved with 1 M aqueous HCl at 0 °C. The organic layer was separated and the aqueous layer was extracted twice with EtOAc. The combined organic layers were washed with saturated aqueous NaHCO3 and brine, and dried over MgSO4. The filtrate was concentrated in vacuo and the resulting crude mixture of the diol 16 and desilylated product was used for the next reaction without further purification.
To a solution of the above crude mixture in dry CH2Cl2 (8.4 mL) was added imidazole (171 mg, 2.5 mmol, 3.0 equiv) and TBSCl (139 mg, 0.92 mmol, 1.1 equiv) at 0 °C under argon. After being stirred at room temperature for 1.5 h, the reaction mixture was quenched with saturated aqueous NH4Cl at 0 °C. The organic layer was separated and the aqueous layer was extracted twice with EtOAc. The combined organic layers were washed with saturated aqueous NaHCO3 and brine, and dried over MgSO4. The filtrate was concentrated in vacuo and the resulting residue was purified by column chromatography on silica gel (20% EtOAc in hexane) to afford the diol 16 (367 mg, 0.611 mmol, 73%) as a colorless oil.
1H NMR (400 MHz, CDCl3) δ 7.24-7.26 (m, 2H), 7.25 (d, J = 8.2 Hz, 1H), 7.02 (d, J = 8.4 Hz, 1H), 6.85 (d, J = 8.2 Hz, 2H), 5.15 (s, 2H), 4.84 (brs, 3H), 4.49 (s, 2H), 4.17 (s, 2H), 3.78 (s, 3H), 3.45 (s, 3H), 1.85(m, 1H), 1.65 (s, 3H), 1.30-1.58 (m, 2H), 1.27 (m, 1H), 1.23 (s, 3H), 1.20 (s, 3H), 0.92 (s, 9H), 0.10 (s, 3H), 0.09 (s, 3H); 13C NMR (100 MHz, CDCl3) δ 159.1, 144.3, 135.8, 131.2, 129.5, 129.1, 125.0, 113.7, 95.0, 72.1, 59.3, 56.20, 56.17, 55.3, 43.8, 29.3, 28.0, 26.1, 24.1, 19.4, 18.4, –5.2; IR (Neat) 3443, 2955, 2930, 1612, 1585, 1514, 1464, 1250, 837, 773 cm–1; HRMS (FAB) calcd for C34H52O7SiNa [M+Na]+ 623.3380, found 621.3416.
Allylic alcohol 17
To a solution of the diol 16 (100 mg, 0.166 mmol) in dry MeCN (3.3 mL) was added CDI (541 mg, 3.3 mmol, 20 equiv) at 0 °C under argon. After being stirred at reflux for 2.5 h, the reaction mixture was quenched with 1 M aqueous HCl at 0 °C. The organic layer was separated and the aqueous layer was extracted twice with EtOAc. The combined organic layers were washed with saturated aqueous NaHCO3 and brine, and dried over Na2SO4. The filtrate was concentrated in vacuo, and the resulting crude carbonate was used for next reaction without further purification.
To a solution of the crude carbonate in dry THF (1.7 mL) was added TBAF (1.0 M in THF solution, 2.5 mL, 2.5 mmol, 15 equiv) at 0 °C under argon. After being stirred at room temperature for 3 h, the reaction mixture was quenched with 1 M aqueous HCl at 0 °C. The organic layer was separated and the aqueous layer was extracted twice with EtOAc. The combined organic layers were washed with saturated aqueous NaHCO3 and brine, and dried over Na2SO4. The filtrate was concentrated in vacuo, and the resulting residue was purified by column chromatography on silica gel (50% EtOAc in hexane) to afford the allylic alcohol 17 (77.7 mg, 0.15 mmol, 91%) as a yellow oil.
1H NMR (400 MHz, CDCl3) δ 7.34 (t, J = 8.0 Hz, 1H), 7.24 (d, J = 8.6 Hz, 2H), 7.14 (d, J = 8.0 Hz, 1H), 6.98 (d, J = 8.0 Hz, 2H), 6.86 (d, J = 8.6 Hz, 2H), 6.10 (s, 1H), 5.17 (s, 2H), 4.77 (d, J = 11.2 Hz, 1H), 4.46 (d, J = 11.2 Hz, 1H), 4.40 (d, J = 11.2 Hz, 2H), 4.18 (d, J = 11.8 Hz, 1H), 4.13 (d, J = 11.8 Hz, 1H), 3.80 (s, 3H), 3.46 (s, 3H), 2.01 (m, 1H), 1.75 (m, 1H), 1.67 (s, 3H), 1.53 (m, 2H), 1.26 (s, 3H), 1.23 (s, 3H); 13C NMR (100 MHz, CDCl3) δ 159.2, 155.7, 155.1, 136.8, 134.3, 133.2, 130.0, 129.4, 129.3, 125.2, 121.1, 114.9, 113.7, 94.9, 90.1, 78.3, 71.8, 61.0, 58.8, 56.3, 55.3, 42.7, 28.9, 24.9, 22.8, 22.6, 19.3; IR (Neat) 3501, 2957, 2934, 2905, 1790, 1612, 1587, 1514, 1464, 1250, 750 cm–1; HRMS (ESI-TOF) calcd for C29H36O8Na [M+Na]+ 535.2302, found 535.2286.
Chloride 18
To a solution of the allylic alcohol 17 (170 mg, 0.33 mmol) and LiCl (28 mg, 0.66 mmol, 2.00 equiv) in dry THF (1.0 mL) and dry CH2Cl2 (5.0 mL) was added triethylamine (0.463 mL, 3.3 mmol, 10.0 equiv) and MsCl (64.2 µL, 0.83 mmol, 2.5 equiv) at 0 °C under argon. After being stirred at room temperature for 1 h, the reaction mixture was quenched with 1 M aqueous HCl at 0 °C. The organic layer was separated and the aqueous layer was extracted three times with EtOAc. The combined organic layers were washed with saturated aqueous NaHCO3 and brine, and dried over Na2SO4. The filtrate was concentrated in vacuo, and the resulting residue was used for next reaction without further purification.
To a solution of the crude PMB ether in CH2Cl2 (3 mL) and H2O (176 µL) was added DDQ (113 mg, 0.50 mmol, 1.50 equiv) at 0 °C. After being stirred at room temperature for 30 min, the reaction mixture was quenched with saturated aqueous NaHCO3. The organic layer was separated and the aqueous layer was extracted twice with EtOAc. The combined organic layers were washed with brine, and dried over Na2SO4. The filtrate was concentrated in vacuo, and the resulting residue was purified by flash column chromatography on silica gel (8% EtOAc in toluene) to afford the benzyl alcohol 14 (93 mg, 0.23 mmol, 63% in 2 steps) as a colorless oil.
1H NMR (400 MHz, CDCl3) δ 7.35 (t, 1H, J = 8.0 Hz, 1H), 7.17 (d, J = 8.0 Hz, 1H), 6.97 (d, J = 8.0 Hz, 1H), 5.95 (s, 1H), 5.25 (s, 2H), 4.71 (d, J = 12.4 Hz, 1H), 4.63 (d, J = 12.4 Hz, 1H), 4.26 (d, J = 12.4 Hz, 1H), 4.16 (d, J = 12.4 Hz, 1H), 3.51 (s, 3H), 2.06 (m, 1H), 1.83 (m, 1H), 1.74 (s, 3H), 1.69 (m, 1H), 1.57 (m, 1H), 1.41 (s, 3H), 1.30 (s, 3H); 13C NMR (100 MHz, CDCl3) δ 155.9, 154.9, 137.0, 135.4, 131.2, 129.3, 128.5, 121.5, 115.4, 115.2, 95.3, 89.6, 56.5, 55.5, 43.2, 41.1, 28.9, 24.8, 23.6, 21.7, 19.3; IR (Neat) 3410, 2974, 2914, 1798, 1589, 1466, 1256, 750 cm-1; HRMS (ESI-TOF) calcd for C21H27O6ClNa [M+Na]+ 433.1388, found 433.1373.
Nine-membered ether 2b
To a suspension of NaH (43.9 mg, 1.83 mmol, 25 equiv) in dry benzene (10 mL, 137 mL/mmol) was added 18-crown-6-ether (96.5 mg, 0.365 mmol, 5.0 equiv) at room temperature. To the mixture was added a solution of the benzylic alcohol 18 (30.0 mg, 73.0 µmol) in dry benzene (2.5 mL) dropwise at room temperature over a period of 30 min. After being stirred at room temperature for 24 h, the reaction mixture was quenched with 1 M aqueous HCl at 0 °C. The organic layer was separated and the aqueous layer was extracted twice with EtOAc. The combined organic layers were washed with saturated aqueous NaHCO3 and brine, and dried over MgSO4. The filtrate was concentrated in vacuo, and the resulting residue was purified by flash column chromatography on silica gel (25% EtOAc in hexane) to afford the nine-membered ether 2b (13.4 mg, 35.8 mmol, 49%).
1H NMR (400 MHz, CDCl3) δ 7.23 (m, 2H), 7.12 (d, J = 7.8 Hz, 1H), 6.22 (s, 1H), 5.23 (s, 2H), 5.00 (d, J = 14.8 Hz, 1H), 4.46 (d, J = 14.8 Hz, 1H), 4.34 (d, J = 12.9 Hz, 1H), 4.28 (d, J = 12.9 Hz, 1H), 3.48 (s, 3H), 2.24 (m, 1H), 2.05 (m, 2H), 1.54 (s, 3H), 1.18 (s, 3H), 1.14 (s, 3H), 0.89 (m, 1H); 13C NMR (100 MHz, CDCl3) δ 155.6, 153.8, 140.1, 133.3, 130.1, 127.7, 127.4, 119.1, 114.1, 94.3, 91.4, 77.7, 66.0, 64.8, 56.2, 39.4, 28.1, 27.5, 23.5, 21.0, 20.4; IR (Neat) 2932, 2853, 1800, 1585, 1461, 1288, 1256, 754 cm–1; HRMS (ESI-TOF) calcd for C21H26O6Na [M+Na]+ 397.1622, found 397.1614.
Procedure for MTT assay
The cytotoxicities of compounds against KB-3-1 and MDR KB-G2 cells were examined according to the following procedure. In brief, the cells were seeded into 96-well culture plates at 2×103 cells/well and pre-cultured for 24 h. After the pre-culture, the cells were cultured with test compounds for 72 h. In the experiments to examine the activities of the compounds to reverse P-glycoprotein-mediated MDR, MDR KB-G2 cells were cultured with various concentrations of them in the presence of paclitaxel. After a 72 h-incubation with the compounds, the cells were treated with 500 µg/mL MTT reagent for 4 h, and then 10% (w/v) SDS in PBS (100 µL/well) was added to solubilize MTT-formazan. After overnight incubation, absorbances at 570 nm and 630 nm were measured using a Multiskan JX system (Dainippon Pharmaceuticals Co., Osaka, Japan) as test and reference wavelengths, respectively.
Procedure for ATPase assay
The effects of the compounds on the ATPase activities of plasma membrane fractions prepared from the cells were measured according to the following procedure with plasma membrane fractions prepared from P-glycoprotein-expressing Sf9 cells.9 In brief, cell membranes (1 μg of protein per well) were incubated with compounds in 96-well plates with 40 μL of the incubation medium [50 mM Tris-Mes (pH 6.8), 2 mM EGTA, 2 mM dithiothreitol, 50 mM potassium chloride, 5 mM sodium azide, 2 mM ouabain, and 2 mM ATP/Mg] at 37 °C for 30 min. The reaction was stopped by the addition of 20 μL of 5% trichloroacetic acid followed by 42 μL of solution A [2 M hydrochloric acid : 0.1 M sodium molybdate = 4 : 3] and 18 μL of solution B [0.084% (w/v) malachite green in 1% (w/ v) polyvinyl alcohol]. Thereafter, 120 μL of solution C [7.8% (v/v) sulfuric acid] was added to the mixture in the well plates. One hour after incubation at room temperature, the absorbance of the reaction mixture in each well was measured at a wavelength of 630 nm using a Multiskan JX system (Dainippon Pharmaceuticals Co., Osaka, Japan). The amount of liberated phosphate was quantified on the basis of the calibration line established with inorganic phosphate standards. Km and Vmax values for test compounds were calculated from the Lineweaver-Burk plots of (compound concentration) × (compound-stimulated ATPase activity)-1 vs (compound concentration).
ACKNOWLEDGEMENTS
This work was supported by Astellas Foundation for Research on Metabolic Disorders (T.D.) and a Grant-in-Aid from MEXT (No.18201041, T.I.).
References
1. For a recent review, see; T. Ishikawa, ‘Nature Encyclopedia of the Human Genome,’ Vol. 4, ed. by D. N. Cooper, Nature Publishing Group, London, 2003, pp. 154–160.
2. E. M. Leslie, R. G. Deeley, and S. P. C. Cole, Toxcol. Appl. Pharm., 2005, 204, 216. CrossRef
3. a) J. Kobayashi, A. Ogiwara, H. Hosoyama, H. Shigemori, N. Yoshida, T. Sasaki, Y. Li, S. Iwasaki, M. Naito, and T. Tsuruno, Tetrahedron, 1994, 50, 7401; CrossRef b) J. Kobayashi, H. Hosoyama, X.–X. Wang, H. Shigemori, Y. Koiso, S. Iwasaki, T. Sasaki, M. Naito, and T. Tsuruno, Bioorg. Med. Chem. Lett., 1997, 7, 393; CrossRef c) I. Ojima, P. Bounaud, C. Takeuchi, P. Pera, and R. J. Bernacki, Bioorg. Med. Chem. Lett., 1998, 8, 189; CrossRef d) J. Kobayashi, H. Hosoyama, X.–X. Wang, H. Shigemori, Y. Sudo, and T. Tsuruno, Bioorg. Med. Chem. Lett., 1998, 8, 1555; CrossRef e) H. Hosoyama, H. Shigemori, A. Tomida, T. Tsuruno, and J. Kobayashi, Bioorg. Med. Chem. Lett., 1999, 9, 389; CrossRef f) I. Ojima, C. P. Borella, X. Wu, P. Bounaud, C. F. Oderda, M. Sturm, M. L. Miller, S. Chakravarty, J. Chen, Q. Huang, P. Pera, T. Brooks, M. R. Baer, and R. J. Bernacki, J. Med. Chem., 2005, 48, 2218. CrossRef
4. K. Morihara, T. Nishimori, H. Kusama, Y. Horiguchi, I. Kuwajima, and T. Tsuruno, Bioorg. Med. Chem. Lett., 1998, 8, 2973. CrossRef
5. T. Takahashi, K. Nakai, T. Doi, M. Yasunaga, H. Nakagawa, and T. Ishikawa, Bioorg. Med. Chem. Lett., 2005, 15, 2601. CrossRef
6. a) I. Ojima, C. M. Sun, M. Zucco, Y. H. Park, O. Duclos, and S. Kuduk, Tetrahedron Lett., 1993, 34, 4149; CrossRef b) R. A. Holton, H.–B. Kim, C. Somoza, F. Liang, R. J. Biediger, P. D. Boatman, M. Shindo, C. C. Smith, S. Kim, H. Nadizadeh, Y. Suzuki, C. Tao, P. Vu, S. Tang, P. Zhang, K. K. Murthi, L. N. Gentile, and J. H. Liu, J. Am. Chem. Soc., 1994, 116, 1599; CrossRef c) W. B. Young, J. J. Masters, and S. J. Danishefsky, J. Am. Chem. Soc., 1995, 117, 5228. CrossRef
7. Y. M. Ahn, D. G. V. Velde, and G. I. Georg, J. Org. Chem., 2002, 67, 7140. CrossRef
8. a) K. C. Nicolaou, P. G. Nantermet, H. Ueno, R. K. Guy, E. A. Couladouros, and E. J. Sorensen, J. Am. Chem. Soc., 1995, 118, 2843; b) W. B. Young, J. J. Masters, and S. J. Danishefsky, J. Am. Chem. Soc., 1995, 17, 5228. CrossRef
9. A. Sakurai, Y. Onishi, H. Hirano, M. Seigneuret, K. Obanayama, G. Kim, E. L. Liew, T. Sakaeda, K. Yoshimura, N. Niikawa, M. Sakurai, and T. Ishikawa, Biochemistry, 2007, 46, 7678. CrossRef
10. K. Tanaka, K. Nakanishi, and N. Berova, J. Am. Chem. Soc., 2003, 125, 10802. CrossRef
11. Detail of the method for determination of the absolute configuration is described in the supporting information.
12. Ojima et al. have reported that the taxanes with modifications at the C-7 position of 10-deacetylbaccatin III (DAB) proved to be very active with >95% reversal activity, see ref 3f.
13. a) K. C. Nicolaou, Z. Yang, E. J. Sorensen, and M. Nakada, J. Chem. Soc., Chem. Commun., 1993, 1024; CrossRef b) K. C. Nicolaou, J.–J. Liu, Z. Yang, H. Ueno, E. J. Sorensen, C. F. Claiborne, R. K. Guy, C.–K. Hwang, M. Nakada, and P. G. Nantermet, J. Am. Chem. Soc., 1995, 117, 634. CrossRef
14. Y. Oikawa, T. Yoshioka, and O. Yonemitsu, Tetrahedron Lett., 1982, 23, 885. CrossRef