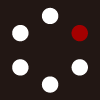
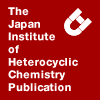
HETEROCYCLES
An International Journal for Reviews and Communications in Heterocyclic ChemistryWeb Edition ISSN: 1881-0942
Published online by The Japan Institute of Heterocyclic Chemistry
e-Journal
Full Text HTML
Received, 24th February, 2014, Accepted, 19th May, 2014, Published online, 27th May, 2014.
DOI: 10.3987/COM-14-S(K)10
■ Unusually Efficient Deformylative Synthesis of 1,2,8,9-Tetrasubstituted Dipyrrins from 4,5-Disubstituted Pyrrole-2-carbaldehydes
Mitsunori Oda,* Yurie Fujiwara, Yoshimitsu Kumai, Akira Ohta, and Ryuta Miyatake
Department of Chemistry, Faculty of Science, Shinshu University, Asahi 3-1-1, Matsumoto, Nagano 390-8621, Japan
Abstract
Upon heating in a mixture of hydrobromic acid and acetic acid, 4-arylmethyl-5-(4-methoxyphenyl)pyrrole-2-carbaldehydes (9a–c) react to give 2,8-bis(arylmethyl)-1,9-bis(4-methoxyphenyl)dipyrrins (10a–c) in high yields, demonstrating the first example of an unusually efficient deformylative transformation of pyrrole-2-carbaldehyde to dipyrrin. Dipyrrins 10 show a clear color change from red to blue, when exposed to Brønsted acid. Structure of 10a·H+ was determined by X-ray crystallographic analysis. The absorption change of 10a in the presence of a metal ion was also studied.INTRODUCTION
Dipyrrins, also called dipyrromethenes, consist of an important class of nitrogen heterocycles.1,2 The structure of dipyrrin can be found as a core carbon skeleton in 4,4-difluoro-4-bora-3a,4a-diaza-s-indacene (BODIPY) dyes,3 and some dipyrrins can be used as synthetic key intermediates for porphyrins and their analogs.4 Therefore, a wide variety of substituted dipyrrins are already known of. Among many reported methods for preparation of dipyrrins, condensations between pyrrole and pyrrole-2-carbaldehyde under acidic conditions and between pyrroles and acid chloride have been well employed (Scheme 1). In the former condensation, besides formation of 3, dipyrrin 5 has often been reported to form in low yields by deformylative condensation (Scheme 2).2,3 The deformylative formation of 5 from 1 under acidic conditions is a minor process and, hence, the synthetic importance of this method has never been emphasized.
Meanwhile, we reported the facile synthesis of 2,3-disubstituted pyrroles 8 from 2-substituted-1-pyrrolines 6 via aldol condensation intermediates 7 (Scheme 3).5 This easy access to these 2,3-disubstituted pyrroles allowed us to extend our synthetic study toward its further derivatization. During the course of this study, we have found that the α-formyl derivatives of 8 undergoes efficient condensation to produce dipyrrins. In this paper, we describe this unusual deformylative condensation, though the reaction is limited to three examples. We also describe the absorption change of the dipyrrins in the presence of Brønsted acid and a metal ion, and formation of its complexes including its BODIPY derivative.3 Furthermore, X-ray crystallographic analysis of CF3SO3H salt of one of the title compounds is disclosed.
RESULTS AND DISCUSSION
Aldehydes 9, 4-arylmethyl-5-(4-methoxyphenyl)pyrrole-2-carbaldehydes, were obtained in good yields by Vilsmeier-Haack reaction6 of 3-arylmethyl-2-(4-methoxyphenyl)pyrroles (8). Upon heating in an aqueous medium containing a Brønsted acid, 9a transformed to 2,8-dibenzyl-1,9-bis(4-methoxyphenyl)dipyrrin (10a) (Scheme 4). Table 1 shows the results of the condensation under various acidic reaction conditions.
Although acetic acid was ineffective as a key acid (entry 5–6), not as a solvent, other stronger acids worked effectively to give dipyrrin 10a in good to high yields. The highest yield was achieved by the way of entry 2. Under the conditions of entry 2, 10b and 10c were obtained also in high yields (entry 8–9). A proposed reaction mechanism is shown in Scheme 5. The deformylation at the pyrrole ring7 probably proceeds through the hydrate 11, which undergoes protonation on the pyrrole ring to give 12. Release of formic acid from 12 provides 8, which adds to protonated 9 to give 13. Finally, dehydration from 13 yields 10·H+. Indeed, it was independently confirmed that 8a reacts with 9a in HBr/AcOH at room temperature to give 10a in 80% yield. It is worth noting that the yield of 10a in the deformylative self-condensation of 9a, requiring a high reaction temperature, is greater than that in the condensation between 8a and 9a at room temperature. In contrast to the results of 9a–c having a 4-methoxyphenyl group, 9d–e having a phenyl group behaves differently. Upon heating in refluxing HBr/AcOH, 9d reacts slightly slower than 9a–c, resulting in the formation of a complex mixture. Although the existence of 10d in the mixture can be deduced by 1H NMR analysis,8 it could not be isolated from the mixture because of its low yield and particularly instability for SiO2 chromatographic purification. A similar result was observed in the reaction of 9e. Although the stability of 10d–e remains uncertain, it is possible that a methoxyl group of the aryl substituents at the 1,9 positions of 10a–c might contribute to their stability. The relatively higher reactivity of 9a–c in comparison with 9d–e in their reactions may be attributable to a more favorable protonation for 12 based on its increased π-electron density at the pyrrole ring induced by the 4-methoxyphenyl groups.
Dipyrrins 10a–c were obtained as red crystals. Their structures were confirmed by spectroscopic analyses. Numbers of 1H- and 13C-NMR signals of 10 in CDCl3 are evidence for its symmetric structure around the meso carbon at the 5 position and, hence, quick tautomerism between the 1H-pyrrole and azacyclopentadienylidene rings under the conditions of NMR measurements. The UV-vis and emission spectra of 10a in MeCN and 1%CF3CO2H(TFA)-MeCN are shown in Figure 1. The absorption maximum at the visible region is observed at 504 nm in MeCN and at 586 nm in 1%TFA-MeCN. While the color of 10a in a MeCN solution is red, it is blue in acidic media.9 The protonated compound, 10a·H+X, can be
isolated also as stable blue crystals. This change is visibly clear even in 10–6 M/L of 10a against a similar concentration of TFA. Thus, 10a can be used as a proton sensor in organic solvents.10 Emission upon exciting at the longest wavelength maximum of 10a was observed both in MeCN and 1%TFA-MeCN, but quantum yields of these emissions were less than 0.01% in 2 x 10–5 M/L solution. The solid-state structure of 10a·H+ triflate was determined by X-ray analysis. ORTEP drawings are shown in Figure 2. The core dipyrrin part of the X-ray structure is almost planar. Two phenyl rings of the benzyl groups direct vertically to the core plane in different directions. One of the anisyl groups attaches to the core plane distortedly with a torsion angle of 34.3˚, and the other with a torsion angle of 18.0˚. Two C–C bonds around the meso carbon atom are 1.371 and 1.409 Å long. Although these lengths are between those of typical C–C single (1.54 Å) and double (1.34 Å) bonds, those are meaningfully different, that is,
the dipyrrin part in the crystal structure has a bond alternation to some extent, apart from the complete resonance hybrid structure.
In addition to the proton affinity of 10a, interaction of 10a with a metal ion was studied in MeCN by absorption spectroscopy. While the absorption spectra of 10a in the presence of monovalent metal ions, such as Na+ and Ag+, resemble that in the absence of a metal ion, the spectra in the presence of divalent and trivalent metal ions (100 eq.) show a clear change. There are two kinds of spectral changes. The spectra in the presence of Mg2+, Al3+, Hg2+, and Pb2+ ions show an absorption band at 586 nm. Those spectra are very similar to that of 10a in 1%TFA-MeCN, suggesting that these bands arise from a type of
complex A and/or B in Chart 1. The spectra in the presence of Ca2+, Zn2+, and Cd2+ ions show two absorption bands at 530–540 nm and 585 nm (Figure 3). The absorption band observed at 540 nm can be assigned to deprotonated complex C, based on comparison of spectral data with that of independently isolated Zn(II) dipyrrinato complex 14 (Scheme 6). Therefore, 10a interacts with Ca2+, Zn2+, and Cd2+ ions to form a mixture of complex A and/or B and complex C, though the molar ratios between 10a and metal ions are unclear under the conditions. These results clearly indicate that the binding site around two nitrogen atoms in 10a only fits metal ions with an relatively smaller ionic radius ranging between 0.70 and 1.00 Å, and, hence, metal ions having either larger or smaller radii are forced to bind in a mode of complex A and/or B. Besides Zn(II) dipyrrinato complex 14, BF2 complex 15, a derivative of BODIPY,3b was also obtained. Both complexes 14 and 15 are isolated as dark red crystals, having a long-wavelength absorption maximum at 537 and 559 nm, respectively, and are stable under various purifications, such as chromatography and recrystallization. The metal to ligand ratio of the Zn(II) dipyrrinato complex was confirmed to be 1:2 by its FAB MS molecular ion peak (m/z 1134). The equivalency of the 1H NMR signals of the dipyrrinato ligands suggests that Zn2+ ion is four-coordinated with a tetrahedral configuration, as previously proposed.10,11 Upon exciting the absorption maximum, 14 emits light at 571 nm and 15 at 585 nm (Table 2). While the emission quantum yield of 14 was low, that of 15 was found to be 51%. With a substantial quantity of easily accessible 10 in hand, further complexation of 10 with other metal ions and its application to electronic materials and biochemical dyes are now in progress.
EXPERIMENTAL
Melting points were measured on a Yanaco MP-3 and were not corrected. IR spectra were recorded on a JASCO FT/IR-4100 spectrometer. UV-vis spectra were measured on a Shimadzu UV-2550 spectrometer. Emission spectra were recorded on a Shimadzu RF-5300PC spectrometer. 1H and 13C-NMR spectra were recorded with tetramethylsilane as internal standard on JEOL λ400 and ECA500 NMR instruments. Chemical shifts of protons on nitrogen atoms are omitted because they shift depending on concentration of the substrate and also on an amount of water in CDCl3. Mass spectra were measured on a JMS-700 mass spectrometer. Ionization mode was carried out by an EI method, except for 10b-c and 14, which were ionized by a FAB method. Column chromatography was done with Silica gel 60N from Kanto Chem., Inc. DMF and MeCN were purified by distillation over CaH2. Phosphorus oxychloride, BF3·OEt2 and zinc acetate were purchased from Wako Chem. and used without purification. TFA and TfOH were purchased from Kotyo Chem. Ind. and used without purification. Emission quantum yields were determined by comparison of a total emission area with that of anthracene (Φ = 0.27, upon excitation at 356 nm in EtOH), if not cited. 2,3-Disubstituted pyrroles 8a–e were prepared by our previously reported method.5 Spectral data of new pyrroles, 8b–c, are as follows.
8b: A dark brown oil. 1H NMR (CDCl3) δ = 3.80 (s, 3H, –OCH 3), 3.89 (s, 2H, CH2), 6.03 (t, J = 2.7 Hz, 1H, H–4), 6.74 (t, J = 2.7 Hz, 1H, H–5), 6.90 (d, J = 8.8 Hz, 2H, 4-MeOC6H4), 7.06 (d, J = 8.1 Hz, 2H, 4-BrC6H4), 7.24 (d, J = 8.8 Hz, 2H, 4-MeOC6H4), 7.36 (d, J = 8.1 Hz, 2H, 4-BrC6H4) ppm; 13C NMR (CDCl3) δ = 31.9, 55.3, 111.1, 114.2, 117.2, 117.7, 119.4, 125.9, 128.2, 129.0, 130.2, 131.3, 141.4, 158.4 ppm; IR (film) νmax = 3432s, 1512vs, 1250vs, 833s cm–1; MS (70 eV) m/z (rel int) 343 (M+, 98), 341 (M+, 100), 340 (21), 262 (9), 187 (10), 186 (73). HRMS m/z Calcd for C18H1679BrNO (M+) 341.0415, found: 341.0417.
8c: Brown solids. Mp 70–71 ˚C. 1H NMR (CDCl3) δ = 3.82 (s, 3H, –OCH3), 4.10 (s, 2H, CH2), 6.20 (t, J = 2.7 Hz, 1H, H–4), 6.78 (t, J = 2.7 Hz, 1H, H–5), 6.81 (m, 1H, –C4H3S), 6.91 (d, J = 3.5 Hz, 1H, –C4H3S), 6.93 (dm, J = 8.5 Hz, 2H, 4–MeOC6H4), 7.11 (dd, J = 5.2, 1.1 Hz, 1H, –C4H3S), 7.32 (dm, J = 8.5 Hz, 2H, 4–MeOC6H4) ppm; 13C NMR (CDCl3) δ = 27.0, 55.3, 111.0, 114.2, 117.1, 118.2, 123.2, 124.2, 125.9, 126.7, 128.3, 128.9, 146.3, 158.5 ppm; IR (KBr) νmax = 3357s, 1509vs, 1253s, 851m, 828m, 816s cm–1; MS (70 eV) m/z (rel int) 269 (M+, 100), 254 (6), 204 (23). HRMS m/z Calcd for C16H15NOS (M+) 269.0874, found: 269.0876.
4-Benzyl-5-(4-methoxyphenyl)pyrrole-2-carbaldehyde (9a)
To a solution of 0.486 mL (6.28 mmol) of DMF in 20 mL of CH2Cl2 at 0 ˚C was added 0.840 mL (9.03 mmol) of POCl3. After being stirred at the same temperature for 2 h, a solution of 1.58 g (6.00 mmol) of 8a in 10 mL of CH2Cl2 was added dropwise to the mixture. Then, the mixture was stirred at room temperature for 3 h, and then was poured into a saturated aqueous NaHCO3 solution and was extracted with CH2Cl2 (50 mL x 3). The combined organic layer was washed with brine and was dried over MgSO4. The solvent was evaporated and the residue was purified by SiO2 chromatography (3%AcOEt-CH2Cl2) to give 1.41 g of 9a (81% yield) as colorless microcrystals. Mp 124–125 °C. 1H NMR (CDCl3) δ = 3.84 (s, 3H, –OCH3), 3.97 (s, 2H, CH2), 6.77 (d, J = 2.8 Hz, 1H, H–3), 6.96 (dm, J = 8.8 Hz, 2H, 4-MeOC6H4), 7.19–7.24 (m, 3H, o- and p-H of Ph), 7.31 (t, J = 7.2 Hz, 2H, m-H of Ph), 7.40 (dm, J = 8.8 Hz, 2H, 4-MeOC6H4), 9.40 (s, 1H, –CHO) ppm; 13C NMR (CDCl3) δ = 32.5, 55.4, 114.5, 122.2, 123.4, 123.7, 126.1, 128.46, 128.52, 128.8, 131.4, 137.4, 140.9, 159.8, 178.4 ppm; IR (KBr) νmax = 3295s, 1633vs, 1243s cm–1; MS (70 eV) m/z (rel int) 291 (M+, 100), 290 (21), 262 (23), 215 (9), 214 (55), 186 (5), 184 (6), 115 (7). HRMS m/z Calcd for C19H17NO2 (M+) 291.1259, found: 291.1260.
4-(4-Bromophenylmethyl)-5-(4-methoxyphenyl)pyrrole-2-carbaldehyde (9b) and 5-(4-methoxyphenyl)-4-(2-thienylmethyl)pyrrole-2-carbaldehyde (9c)
By the same procedure to the synthesis of 9a, 9b and 9c were synthesized from 8b and 8c in 87 and 64% yields, respectively. 9b: Creamy white solids. Mp 144–145 ˚C. 1H NMR (CDCl3) δ = 3.84 (s, 3H, –OCH3), 3.91 (s, 2H, CH2), 6.74 (d, J = 2.6 Hz, 1H, H–3), 6.96 (dm, J = 8.8 Hz, 2H, 4-MeOC6H4), 7.06 (dm, J = 8.4 Hz, 2H, 4-BrC6H4), 7.36 (dm, J = 8.8 Hz, 2H, 4-MeOC6H4), 7.41 (dm, J = 8.4 Hz, 2H, 4-BrC6H4), 9.41 (s, 1H, –CHO) ppm; 13C NMR (CDCl3) δ = 32.0, 55.4, 114.6, 120.0, 121.5, 123.1, 123.5, 128.8, 130.2, 131.5, 131.6, 137.5, 139.9, 159.9, 178.5 ppm; IR (KBr) νmax = 1640vs, 1609s, 1253s, 838s cm–1; MS (70 eV) m/z (rel int) 371 (M+, 100), 370 (34), 369 (M+, 100), 368 (14), 342 (14), 340 (15), 210 (40). HRMS m/z Calcd for C19H1679BrNO2 (M+) 369.0364, found: 369.0362. 9c: Creamy white solids. Mp 132–134 ˚C. 1H NMR (CDCl3) δ = 3.84 (s, 3H, –OCH3), 4.12 (s, 2H, CH2), 6.81 (m, 1H, –C4H3S), 6.90 (d, J = 2.8 Hz, 1H, H–3), 6.94 (d, J = 2.8 Hz Hz, 1H, –C4H3S), 6.96 (dm, J = 9.5 Hz, 2H, 4–MeOC6H4), 7.15 (dd. J = 5.2, 1.3 Hz, 1H, –C4H3S), 7.41 (dm, J = 9.5 Hz, 2H, 4–MeOC6H4), 9.43 (s, 1H, –CHO) ppm; 13C NMR (CDCl3) δ = 27.0, 55.4, 114.5, 121.8, 123.1, 123.5, 123.8, 124.8, 126.9, 129.0, 131.5, 137.5, 144.4, 159.9, 178.6 ppm; IR (KBr) νmax = 3254vs, 1642vs, 1251s, 848s, 839s, 813m cm–1; MS (70 eV) m/z (rel int) 297 (M+, 100), 268 (45), 214 (10). HRMS m/z Calcd for C17H15NO2S (M+) 297.0824, found: 297.0823.
4-Benzyl-5-phenylpyrrole-2-carbaldehyde (9d) and 5-phenyl-4-(2-thienylmethyl)pyrrole-2-carbaldehyde (9e)
To a solution of 116 µL (1.50 mmol) of DMF in 10 mL of CH2Cl2 at 0 ˚C was added 140 µL (1.50 mmol) of POCl3. After being stirred at the same temperature for 2 h, a solution of 233 mg (1.00 mmol) of 8d in 4 mL of CH2Cl2 was added dropwise to the mixture. Then, the mixture was stirred at room temperature for 3 h, and then was poured into a saturated aqueous NaHCO3 solution and was extracted with CH2Cl2 (50 mL x 3). The combined organic layer was washed with brine and was dried over MgSO4. The solvent was evaporated and the residue was purified by SiO2 chromatography (1%AcOEt-CH2Cl2) to give 251 mg of 9d (96% yield) as colorless microcrystals. Mp 105–107 °C. 1H NMR (CDCl3) δ = 4.00 (s, 2H, CH2), 6.77 (d, J = 2.5 Hz, 1H, H–3), 7.19–7.23 (m, 3H, o- and p-H of Ph), 7.30 (t, J = 7.5 Hz, 2H, m-H of Bn), 7.37 (t, J = 7.5 Hz, 1H, p-H of Bn), 7.41– 7.48 (m, 4H, m-H of Ph and o-H of Bn), 9.42 (s, 1H, –CHO) ppm; 13C NMR (CDCl3) δ = 32.4, 122.8, 123.3, 126.1, 127.5, 128.48 (2C), 128.51, 129.0, 131.2, 131.8, 137.3, 140.8, 178.8 ppm; IR (KBr) νmax = 3289s, 1644vs, 692s cm–1; MS (70 eV) m/z (rel int) 261 (M+, 100), 260 (26), 233 (10), 232 (46), 185 (10), 184 (68), 154 (21), 128 (13), 127 (13), 77 (13). HRMS m/z Calcd for C18H15NO (M+) 261.1154, found: 261.1151.
By the same procedure to the synthesis of 9d, 9e was synthesized from 8e in 72% yield. 9e: Colorless plates. Mp 105–106 ˚C. 1H NMR (CDCl3) δ = 4.15 (s, 2H, CH2), 6.82 (dd, J = 3.5, 1,1 Hz, 1H, –C4H3S), 6.92 (d, J = 2.6 Hz, 1H, H–3), 6.94 (dd, J = 5.0, 3.5 Hz, 1H, –C4H3S), 7.16 (dd, J = 5.0, 1,1 Hz, 1H, –C4H3S), 7.34 (tt, J = 7.1, 2.0 Hz, 1H, p-H of Ph), 7.45 (tt, J = 7.1, 2.0 Hz, 2H, m-H of Ph), 7.48 (dt, J = 7.1, 2.0 Hz, 2H, o-H of Ph), 9.47 (s, 1H, –CHO) ppm; 13C NMR (CDCl3) δ = 27.0, 122.4, 122.8, 123.8, 124.8, 126.9, 127.6, 128.7, 129.1, 131.1, 131.8, 137.2, 144.2, 178.8 ppm; IR (KBr) νmax = 3236s, 1645vs, 922m, 850m, 807m cm–1; MS (70 eV) m/z (rel int) 267 (M+, 100), 266 (37), 239 (11), 228 (65), 154 (10). HRMS m/z Calcd for C16H13NOS (M+) 267.0718, found: 267.0716.
2,8-Dibenzyl-1,9-bis(4-methoxyphenyl)dipyrrin (10a) from 9a
A mixture of 209 mg (0.718 mmol) of 9a in a mixture of AcOH (5 mL) and 48% HBr (5 mL) was heated at 85 ˚C on an oil bath under nitrogen atmosphere for 4 h. The resulted blue reaction mixture was carefully poured into a cold aqueous NaHCO3 solution and extracted with CHCl3 (20 mL x 3). The combined organic layer was washed with brine and dried over Na2SO4. The solvent was removed and the red residue was purified by SiO2 chromatography (10%AcOEt-CHCl3) to give 179 mg (93% yield) of 10a as red microcrystals. Mp 161–163 °C. 1H NMR (CDCl3) δ = 3.85 (s, 6H, –OCH3), 4.02 (s, 4H, CH2), 6.55 (s, 2H, H–3,7), 6.62 (s, 1H, H–5), 6.94 (dm, J = 8.0 Hz, 4H, 4-MeOC6H4), 7.19–7.23 (m, 6H, o- and p-H of Ph), 7.29 (t, J = 8.0 Hz, 4H, m-H of Ph), 7.64 (dm, J = 8.0 Hz, 4H, 4-MeOC6H4) ppm; 13C NMR (CDCl3) δ = 33.7, 55.4, 114.1, 126.0, 126.7, 128.4, 128.7, 128.9, 129.0, 130.2, 139.5, 140.7, 152.6, 159.8, 169.7 ppm; IR (KBr) νmax = 1604s, 1252vs, 1175m cm–1; UV-vis (MeOH) λmax = 291 (logε = 4.32), 504 (4.42) nm; MS (70 eV) m/z (rel int) 536 (M+, 100), 445 (7), 276 (19), 268 (9), 264 (7), 263 (36), 262 (5), 186 (10). HRMS m/z Calcd for C37H32N2O2 (M+) 536.2464, found: 536.2467. The TfOH salt of 10a, 10a·HOTf, was obtained quantitatively as blue microplates from 10a and TfOH in EtOH. Mp 197–199 °C. 1H NMR (DMSO-d6) δ = 3.84 (s, 6H, –OCH3), 4.14 (s, 4H, CH2), 7.13 (d, J = 8.5 Hz, 4H, 4-MeOC6H4), 7.22-7.24 (m, 8H, o- and p-H of Ph and H–3,7), 7.31 (t, J = 7.5 Hz, 4H, m-H of Ph), 7.71 (d, J = 8.5 Hz, 4H, 4-MeOC6H4), 7.76 (s, 1H, H–5) ppm; 13C NMR (DMSO-d6) δ = 32.3, 55.5, 114.7, 121.2, 120.5 (q, J = 322.2 Hz, –CF3), 126.3, 127.3, 127.7, 128.2, 128.5, 129.7, 131.5, 132.9, 139.2, 148.8, 161.1 ppm; IR (KBr) νmax =1598s, 1258s, 1243s, 1167s cm–1. A sample for X-ray analysis was obtained by recrystallization from hexane-CH2Cl2. Similarly, the HBr salt, 10a·HBr, was obtained as blue microcrystals. Mp 185–186 °C. 1H NMR (DMSO-d6) δ = 3.84 (s, 6H, –OCH3), 4.14 (s, 4H, CH2), 7.12 (d, J = 8.5 Hz, 4H, 4-MeOC6H4), 7.20–7.24 (m, 8H, o- and p-H of Ph and H–3,7), 7.31 (t, J = 8.0 Hz, 4H, m-H of Ph), 7.71 (d, J = 8.5 Hz, 4H, 4-MeOC6H4), 7.76 (s, 1H, H–5) ppm; 13C NMR (CDCl3) δ = 32.3, 55.4, 114.7, 121.1, 126.2, 127.2, 127.7, 128.1, 128.5, 129.7, 131.5, 132.8, 139.1, 148.7, 161.1 ppm; IR (KBr) νmax =1597vs, 1256vs, 1243s, 1179s, 1169s, 1160s cm–1; UV-vis (MeOH) λmax = 279 (logε = 4.20), 317sh (3.92), 376 (3.49), 585 (4.81) nm. Anal. Calcd C37H33BrN2O2·H2O: C 69.92, H 5.55, N, 4.41%. Found C 69.75, H 5.69, N 4.28%.
2,8-Bis(4-bromophenylmethyl)-1,9-bis(4-methoxyphenyl)dipyrrin (10b) and 1,9-bis(4-methoxyphenyl)-2,8-bis(2-thienylmethyl)dipyrrin (10c)
By the same procedure to the synthesis of 10a, 10b and 10c were synthesized from 9b and 9c in 95 and 90% yields, respectively. 10b: Red prisms. Mp 156–158 ˚C. 1H NMR (CDCl3) δ = 3.85 (s, 6H, –OCH3), 3.97 (s, 4H, CH2), 6.55 (s, 2H, H–3, 7), 6.64 (s, 1H, H–5), 6.95 (dm, J = 8.6 Hz, 4H, 4–MeOC6H4), 7.10 (dm, J = 8.6 Hz, 4–BrC6H4), 7.41 (dm, J = 8.6 Hz, 4H, 4–MeOC6H4), 7.61 (dm, J = 8.6 Hz, 4H, 4–BrC6H4) ppm; 13C NMR (CDCl3) δ = 33.3, 55.5, 114.3, 120.0, 122.4, 126.6, 129.0, 129.1, 129.6, 130.5, 131.6, 139.6, 139.8, 152.8, 160.0 ppm; IR (KBr) νmax = 1607s, 1251s, 602m cm-1; UV–vis (MeCN) λmax = 220sh (logε = 4.57), 272sh (4.33), 292 (4.44), 505 (4.55) nm; UV–vis (1%TFA–MeCN) λmax = 278 (4.19), 321 (3.91), 381 (3.57), 586 (5.00) nm; FABMS m/z (rel int) 697 (M++H, 35), 695 (M++H, 56), 693 (M++H, 34), 307 (13), 289 (11), 252 (31), 154 (nitrobenzyl alcohol++H, 100).12 HRMS m/z Calcd for C37H3179Br2N2O2 (M++H) 693.0758, found: 693.0753. 10c: Red microcrystals. Mp 160–161 ˚C. 1H NMR (CDCl3) δ = 3.86 (s, 6H, –OCH3), 4.19 (s, 4H, CH2), 6.71 (s, 1H, H–5), 6.75 (s, 2H, H–3, 7), 6.84 (m, 2H, –C4H3S), 6.93 (d, J = 3.5 Hz, 2H, –C4H3S), 6.96 (dm, J = 9.1 Hz, 4H, 4–MeOC6H4), 7.16 (dd, J = 5.2, 0.9 Hz, 2H, –C4H3S), 7.65 (dm, J = 9.1 Hz, 4H, 4–MeOC6H4) ppm; 13C NMR (CDCl3) δ = 28.1, 55.4, 114.2, 122.7, 123.7, 124.9, 126.5, 126.9, 128.9, 129.1, 129.6, 139.5, 144.1, 152.6, 159.9 ppm; IR (KBr) νmax = 1610s, 1254s, 839m, 818m, 800m cm–1; UV–vis (MeCN) λmax = 232 (logε = 4.37), 291 (4.37), 349sh (3.64), 503 (4.46) nm; UV–vis (1%TFA–MeCN) λmax = 237 (4.22), 279 (4.14), 322 (3.83), 386 (3.55), 585 (4.88) nm; FABMS m/z (rel int) 549 (M++H, 100), 307 (23), 289 (18), 154 (nitrobenzyl alcohol++H, 100).12 HRMS m/z Calcd for C33H29N2O2S2 (M++H) 549.1670, found: 549.1676.
2,8-Dibenzyl-1,9-bis(4-methoxyphenyl)dipyrrin (10a) from 8a and 9a
A mixture of 22 mg (0.086 mmol) of 8a and 25 mg (0.086 mmol) of 9a in a mixture of AcOH (1 mL) and 48% HBr (1 mL) was stirred at room temperature under nitrogen atmosphere for 15 h. The resulted blue reaction mixture was carefully poured into a cold aqueous NaHCO3 solution and extracted with CHCl3 (20 mL x 3). The combined organic layer was washed with brine and dried over Na2SO4. The solvent was removed and the red residue was purified by SiO2 column chromatography (10%AcOEt–CHCl3) to give 36 mg (80% yield) of 10a as red solids.
Zinc(II) bis[2,8-dibenzyl-1,9-bis(4-methoxyphenyl)dipyrrinato] (14)
A mixture of 53.6 mg (0.100 mmol) of 10a, 10.1 mg (0.0550 mmol) of zinc acetate, and 10 mL of piperidine in MeOH (3 mL) was stirred at room temperature under nitrogen atmosphere for 2 h. The solids formed were collected and washed well with water and, then recrystallized from hexane-CHCl3 to give 28.0 mg (49% yield) of 14 as dark red microcrystals. Mp 188–190 °C. 1H NMR (CDCl3) δ = 3.70 (s, 12H –OCH3), 3.71 (s, 8H, CH2), 6.48 (m, 6H, H–3, 5, 7), 6.50 (dm, J = 9.0 Hz, 8H, 4-MeOC6H4), 6.99 (dm, J = 9.0 Hz, 8H, 4-MeOC6H4), 7.07 (d, J = 7.3 Hz, 8H, o-H of Bn), 7.21 (t, J = 7.3 Hz, 4H, p-H of Bn), 7.31 (t, J = 7.3 Hz, 8H, m-H of Bn) ppm; 13C NMR (CDCl3) δ = 32.8, 55.3, 113.0, 125.7, 127.1, 128.2, 128.5, 128.9, 129.3, 131.5, 138.5, 141.8, 153.7, 158.2, 158.8 ppm; IR (KBr) νmax = 1594vs, 1223s, 1168s, 1023vs, 983s cm–1; UV (MeOH) λmax = 290 (logε = 4.49), 347 (3.97), 537 (5.05) nm; FABMS m/z (rel int) 1134 (M+, 5), 307 (10), 154 (nitrobenzyl alcohol++H, 100).12 HRMS m/z Calcd for C74H62N4O4Zn (M+) 1134.4063, found: 1134.4057.
2,3-Dibenzyl-4,4-difluoro-3,5-bis(4-methoxyphenyl)-4-bora-3a,4a,-diaza-s-indacene (15)
A mixture of 268 mg (0.500 mmol) of 10a, 2.76 mL (22.5 mmol) of BF3·OEt2, and 1.39 mL (10.0 mmol) of Et3N in CH2Cl2 (12 mL) was refluxed on an oil bath under nitrogen atmosphere for 3 h. The resulted reaction mixture was poured into a saturated aqueous NaHCO3 solution and was extracted with CH2Cl2 (50 mL x 3). The combined organic layer was washed with brine and was dried over MgSO4. The solvent was evaporated and the residue was purified by SiO2 chromatography (toluene-CH2Cl2) to give 104 mg of 15 (35% yield) as dark red microcrystals. Mp 183–185 °C. 1H NMR (CDCl3) δ = 3.68 (s, 4H, CH2), 3.80 (s, 6H, –OCH3), 6.75 (s, 2H, H–3, 7), 6.89 (dm, J = 9.0 Hz, 4H, 4-MeOC6H4), 7.02 (s, 1H, H–5), 7.09 (d, J = 7.3 Hz, 4H, o-H of Bn), 7.19 (t, J = 7.3 Hz, 2H, p-H of Bn), 7.26 (t, J = 7.3 Hz, 4H, m-H of Bn), 7.45 (dm, J = 9.0 Hz, 4H, 4-MeOC6H4) ppm; 13C NMR (CDCl3) δ = 47.1, 55.2, 113.4, 123.9, 126.1, 126.5, 128.4, 128.6, 129.1, 131.2, 133.0, 134.4, 140.3, 157.4, 160.1 ppm; IR (ATR) νmax = 1607vs, 1227s, 1177s, 1166s, 1065vs cm–1; UV-vis (MeOH) λmax = 264 (logε = 4.13), 313sh (3.79), 371 (4.00), 559 (4.80) nm; MS (70 eV) m/z (rel int) 584 (M+, 100), 583 (25), 507 (7), 475 (3), 244 (3), 243 (9). HRMS m/z Calcd for C37H31BF2N2O2 (M+) 584.2447, found: 584.2447.
Measurement of basicity of 10a
The pKa value of the conjugate acid, 10a·H+, was determined by measuring pH of a mixture of 10a and 10a·H+. The pH measurement and calculations for pKa value were carried out according to the method reported by Krumholz.13 Since 10a was entirely insoluble in water, measurements were done in 50%MeOH-H2O without adding inorganic salt to adjust ionic strength. The bromide salt was used for 10a·H+. A TPX-90Si glass electrode pH meter, available from TOKO Chemical Laboratories Co., was used for pH measurements.
X-Ray crystallographic analysis of 10a·HOTf: Diffraction measurements were conducted using a Rigaku R-AXIS RAPID diffractometer at –120 ˚C. Crystal data are as follows; triclinic, space group; P1 (# 1), a; 5.5545 (1) Å, b; 10.8622 (2) Å, c; 14.3493 (3) Å, α; 79.0386 (9)°, β; 85.273 (1)°, γ; 78.4253 (9)°, V; 831.79 (3) Å3, Z; 1, R; 0.1072, wR2; 0.2854, R1; 0.0908 (I>2.00σ(I)), and S; 1.127. The relatively large R values are mainly attributed to thermal vibration of fluorine atoms of the triflate anion. Tables of fractional atomic coordinates, thermal parameters, bond lengths, and angles have been deposited at the Cambridge Crystallographic Data Centre, 12 Union Road, Cambridge CB2 1EZ, United Kingdom (CCDC 941475) [Derect line: +44 1223 762910, Fax: +44 (0) 1233 336033, e-mail: deposit@ccdc.cam.ac.uk].
ACKNOWLEDGEMENTS
A financial support from the Faculty of Science in Shinshu University (for M.O.) is greatly acknowledged.
References
1. In this paper we uniformly use dipyrrin, as a name of compound 3, not dipyrromethene, and its numbering system, which is shown in compound 3 in Scheme 1.
2. T. E. Wood and A. Thompson, Chem. Rev., 2007, 107, 1831. CrossRef
3. a) A. Loudet and L. Burgess, Chem. Rev., 2007, 107, 4891; CrossRef b) G. Ulrich, R. Ziessel, and A. Harriman, Angew. Chem. Int. Ed., 2008, 47, 1184; CrossRef c) M. Benstead, G. H. Mehl, and R. W. Boyle, Tetrahedron, 2011, 67, 3573. CrossRef
4. J. B. Paine, III, ‘The Porphyrins’ Vol. 1, ed. by D. Dolphin, Academic Press, New York, 1978.
5. Y. Fujiwara, M. Oda, T. Shoji, T. Abe, and S. Kuroda, Heterocycles, 2012, 85, 1187; CrossRef We also reported a simple synthetic method for 1,3-disubstituted pyrroles; M. Oda, Y. Fukuchi, S. Ito, N. C. Thanh, and S. Kuroda, Tetrahedron Lett., 2007, 48, 9159. CrossRef
6. L. Kürti and B. Czakó, ‘Strategic Applications of Named Reactions in Organic Synthesis’, Elsevier Academic Press, Amsterdam, 2005, pp. 468–469.
7. For an example of deformylation of pyrrolecarbaldehydes, see a following paper; K. M. Smith, M. Miura, and H. D. Tabba, J. Org. Chem., 1983, 48, 4779. CrossRef
8. 1H NMR signals of 10d-e in the crude products are as follows. 10d: δ (CDCl3) = 4.20 (s, 4H, CH2), 6.76 (s, 1H, H–5), 6.78 (s, 2H, H–3, 7), 6.85 (dd, J = 3.5, 1.2 Hz, 2H, H’-3 of C4H3S), 6.94 (dd, J = 6.5, 3.5 Hz, 2H, H’-4 of C4H3S), 7.16 (dd, J = 6.5, 1.2 Hz, 2H, H’-5 of C4H3S), 7.36 (tm, J = 7.6 Hz, 2H, p-H of Ph), 7.43 (tm, J = 7.6 Hz, 4H, m-H of Ph), 7.16 (dm, J = 7.6 Hz, 4H, o-H of Ph) ppm. 10e: δ (CDCl3) = 4.02 (s, 4H, CH2), 6.56 (s, 2H, H–3, 7), 6.66 (s, 1H, H–5), 7.21 (m, 6H, o- and p-H of Bn), 7.29 (d, J = 7.5 Hz, 4H, m-H of Bn), 7.35 (tm, J = 7.8 Hz, 2H, p-H of 1,9-Ph), 7.41 (tm, J = 7.8 Hz, 4H, m-H of 1,9-Ph), 7.69 (dm, J = 7.8 Hz, 4H, o-H of Ph) ppm.
9. Basicity (pKb value) of 10a was determined to be 8.53 ± 0.06 in 50%MeOH-H2O. For details, see Experimantal.
10. Murakami et al. reported electronic spectra of 1,9-diphenyldipyrrin and its HCl salt. Difference of wavelength of absorption maxima between them in chloroform is 40 nm; Y. Murakami and K. Sakata, Bull. Chem. Soc. Jpn., 1974, 47, 3025. Also, difference of wavelength of absorption maxima of the crude 10e in MeCN and 1%TFA-MeCN is 63 nm. It is worth noting that the difference (82 nm) between 10a and 10aH+ is far larger than those of other dipyrrins. CrossRef
11. I. V. Sazanovich, C. Kirmaier, E. Hindin, L. Yu, D. F. Bocian, J. S. Lindsey, and D. Holten, J. Am. Chem. Soc., 2004, 126, 2664. CrossRef
12. The molecular ion peak was not observed by measurements of an EI mode.
13. P. Krumholz, J. Am. Chem. Soc., 1951, 73, 3487. CrossRef