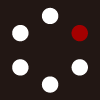
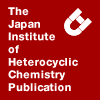
HETEROCYCLES
An International Journal for Reviews and Communications in Heterocyclic ChemistryWeb Edition ISSN: 1881-0942
Published online by The Japan Institute of Heterocyclic Chemistry
e-Journal
Full Text HTML
Received, 27th February, 2014, Accepted, 26th March, 2014, Published online, 2nd April, 2014.
DOI: 10.3987/COM-14-S(K)12
■ Synthesis of Unprotected CH2-Skipped Piperazine-Pyridine Alternating Cycles with Azide End-Group
Andi Kipper, Indrek Kalvet, Kaido Tämm, Lauri Sikk, Peeter Burk, Kuldar Kõiv, and Uno Mäeorg*
Institute of Chemistry, University of Tartu, Ravila 14a, Tartu 50411, Estonia
Abstract
This work describes synthesis of CH2-skipped alternating piperazine-pyridine cycles with azide end-group starting from piperazine, 1-methylpiperazine and pyridine-2,6-dicarboxylic acid. This compound can be used to enhance binding efficiency by shielding repulsion between negatively charged phosphate groups in DNA-oligonucleotide hybridization techniques.Piperazine derivatives are useful in the treatment or prevention of many diseases like inflammation,1 bone degradation,2 thrombosis3 and tumor metastasis.4 On the other hand oligomeric piperazine compounds could be source of potential positive charge which is important factor in interactions of small molecules with biological systems. Subsequent functionality and reactivity offer a good possibility to regulate hydrophilicity/hydrophobicity, geometry, π-interaction and as a result design the appropriate molecule for certain purposes.
The main factors affecting efficiency of techniques using hybridization between single-stranded DNA-helix and oligonucleotide (i.e. PCR, NASBA, LAMP, DNA-chips, etc.) are binding strength and -specificity of those two counterparts. It has been previously shown that functionalization of oligonucleotide with side chains containing amino groups is one way to improve hybridization efficiency.5 In the same article authors have shown that oligonucleotide-DNA helix hybridization temperature depends on the number of amino groups in the side-chain. According to our preliminary unpublished work, the most effective motifs bearing amino-groups would be piperazine rings.6
The aim of current work was to develop simple and cost-effective synthetic route to CH2 skipped piperazine-pyridine alternating structures with useful end-group “handle” for further functionalization. Similar unfunctionalized symmetrical structures have previously been synthesized by Rissanen et al. to analyze its complexation with Cd metal.7 According to our knowledge, synthesis of such asymmetrical molecules has not been previously described.
Synthesis of target molecule (Scheme 1) was performed starting only from pyridine-2,6-dicarboxylic acid, piperazine and N-methylpiperazine using simple reagents available in every organic chemistry laboratory.
Pyridine-2,6-dicarboxylic acid was converted into dimethyl ester 1 by chlorinating with thionyl chloride followed by refluxing with absolute methanol. Diester 2 was reduced with NaBH4 using slow methanol addition procedure described by Soai et al.8 Methanol reacts with NaBH4 and there are constantly small amounts of methoxyborohydrides in solution which have stronger reducing power than regular borohydride and able to reduce methyl esters. This also allows us to avoid more expensive and dangerous LiAlH4 which is commonly used for reduction of esters.
The key intermediate 3 was prepared by mono-bromination of 2 in concentrated aqueous HBr at reflux temperature. Care must be taken to optimize reaction time – reaction is difficult to monitor by TLC as diol 2 remains in water-phase during extraction therefore giving false impression of high conversion while in fact, most of the starting material is still unreacted. On the other hand too long reaction times increase the amount of dibrominated product and reduce the yield of 3.
Compound 3 was coupled with 1-methylpiperazine under mild conditions using potassium carbonate as a base giving 4 in excellent yield. The poor solubility of K2CO3 in reaction media (acetonitrile) did not prevent reaction from taking place but simplified following work-up (only filtration and removal of solvent). Compound 10 was obtained by treating 4 with thionyl chloride. The product of this reaction was not stable enough to store overnight and was therefore prepared just before final coupling reaction.
Azide functionality was introduced into target structure by treating 3 with NaN3 yielding compound 5, which in turn was converted into an electrophilic compound upon treatment with MsCl. Thus obtained compound 6 was coupled with N-Boc-piperazine under previously described N-alkylation conditions giving 8. Instead of Boc-protection, the use of excess of unprotected piperazine could be considered but subsequently one has to be aware of the tedious removal of the leftover piperazine. 8 was then deprotected with trifluoroacetic acid (TFA) and coupled with freshly prepared 10 under aforementioned N-alkylation conditions to give target compound 11 in acceptable yield.
The main reason for the low yield of 10 and 11 is probably the instability of tertiary amines bearing chloride group. Relative stability of compound 10 in solution of DCM and its decomposition upon concentration suggests that the main reason of instability is intermolecular quaternary ammonium salt formation.
Synthesis of target molecule was performed in 11 steps (Scheme 1) from easily available, low-cost starting materials and scalable synthetic methods in 6% yield from pyridine-2,6-dicarboxylic acid.
EXPERIMENTAL
Starting materials, reagents and analytical-grade solvents were purchased from commercial sources. Preparative chromatographic separations were performed on silica gel (70-230 mesh, Machery-Nagel Kieselgel 60) and reaction progress was monitored by analytical thin-layer chromatography (TLC) on pre-coated silica gel (Machery-Nagel ALUGRAM(R)) plates, spots were detected by UV light (λ 254 nm) or KMnO4 staining. IR spectra were measured on a Perkin-Elmer Spectrum BXII FTIR spectrometer using ATR technique with ZnSe. 1H and 13C NMR spectra were recorded on a Bruker Avance-II or Bruker Avance-III at field strength 200/400 and 50/100 MHz for 1H and 13C respectively and samples measured in CDCl3 (unless otherwise indicated) using TMS (δ 0.00) or residual CHCl3 (1H NMR δ 7.26 and 13C NMR δ 77.16) as an internal standard. Chemical shifts (δ) are expressed in ppm and coupling constants (J) in Hz, multiplicity (br=broad, s=singlet, d=doublet, t=triplet, m=multiplet) and integrals are indicated. Moisture and air sensitive reactions were carried out in vacuum-dried, septum capped flasks under nitrogen atmosphere. Solvents were dried over 3Å molecular sieves.
Synthesis of dimethyl pyridine-2,6-dicarboxylate (1): Compound was synthesized according to previously published procedure in 93% yield; NMR data matched literature values.9
Synthesis of 2,6-di(hydroxymethyl)pyridine (2): Compound was synthesized according to previously published procedure in 92% yield;8 NMR data matched literature values.10
Synthesis of 2-(bromomethyl)-6-(hydroxymethyl)pyridine (3): Compound was synthesized according to previously published procedure in 33% yield; NMR data matched literature values.11
Synthesis of 2-(hydroxymethyl)-6-[(4-methylpiperazine-1-yl)methyl]pyridine (4): Compound 3 (200 mg, 0.99 mmol) and N-methylpiperazine (109 μL, 1.2 mmol) were dissolved in anhydrous acetonitrile (ACN) (8 mL) under nitrogen atmosphere. Anhydrous K2CO3 was added and reaction mixture stirred at room temperature for 1 h. K2CO3 was filtered off and solvent evaporated under reduced pressure. Product (197 mg, 0.89 mmol) was obtained as yellow oil in 90% yield. IR 3214 cm-1. 1H NMR (200 MHz, CDCl3) δ = 7.65 (t, J = 7.7, 1H, Py), 7.30 (d, J = 7.6, 1H, Py), 7.20 (d, J = 7.6, 1H, Py), 4.77 (s, 1H, OH), 4.74 (s, 2H, CH2OH), 3.68 (s, 2H, PyCH2N), 2.56 (m, 8H, CH2), 2.32 (s, 3H, CH3). 13C NMR (50 MHz, CDCl3) δ 159.2 (Py), 157.4 (Py), 137.1 (Py), 121.7 (Py), 118.9 (Py), 64.3 (NCH2Py), 64.1 (PyCH2OH), 55.0 (NCH2CH2N), 52.9 (NCH2CH2N), 45.8 (CH3).
Synthesis of 2-(azidomethyl)-6-(hydroxymethyl)pyridine (5): Compound was synthesized according to previously published procedure in 80% yield; NMR data matched literature values.12
Synthesis of 2-(azidomethyl)-6-(methanesulofonylmethyl)pyridine (6): Compound 5 (340 mg, 2.07 mmol) and Et3N (375 μL, 2.69 mmol) were dissolved in DCM (15 mL). MsCl (192 μL, 2.49 mmol) in DCM (200 μL) was added dropwise over 15 min. After stirring for 15 min at room temperature, solvent was removed under vacuum and residue filtered through plug of silica and silica washed with EtOAc. Filtrate was concentrated and dried in vacuum. Title compound (435 mg, 1.80 mmol) was obtained as red oil in 87% yield. 1H NMR (200 MHz, CDCl3) δ = 7.81 (t, J = 7.8, 1H, Py), 7.44 (d, J = 7.6, 1H, Py), 7.35 (d, J = 7.9, 1H, Py), 5.34 (s, 2H, CH2OMs), 4.48 (s, 2H, CH2N3), 3.11 (s, 3H, CH3). 13C NMR (50 MHz, CDCl3) δ 156.1 (Py), 154.0 (Py), 138.3 (Py), 122.0 (Py), 121.6 (Py), 71.4 (PyCH2OMs), 55.5 (CH2N3), 38.2 (CH3). HRMS (ESI) calcd for C8H10N4O3S [M+H+] 243.05464, found 243.05576.
Synthesis of tert-butyl piperazine-1-carboxylate (7): Compound was synthesized according to previously published procedure in 92% yield; NMR data matched literature values.13
Synthesis of 1-{[6-(azidomethyl)pyridin-2-yl]methyl}piperazine (9): Compound 6 (400 mg, 1.65 mmol), compound 7 (333 mg, 1.79 mmol) and anhydrous K2CO3 (200 mg, 1.44 mmol) were suspended in anhydrous ACN (10 mL) in nitrogen atmosphere. Reaction mixture was stirred for 10 h at room temperature, after which it was filtered and filtrate concentrated in vacuum. Product was purified by column chromatography (DCM:MeOH 20:1 → 10:1) which afforded compound 8 (477 mg, 1.43 mmol) as a yellow oil in 87% yield. Compound 8 (477 mg, 1.43 mmol) was dissolved in DCM (37 mL) and trifluoroacetic acid (2.4 mL, 31.5 mmol) was added at room temperature. After 6 h DCM was added (20 mL) and solution neutralized with 1M NaOH solution (40 mL), the organic phase was further washed with 1M NaOH solution (30 mL) and brine (15 mL). Combined water fractions were further extracted with DCM (4 x 20 mL), organic fractions combined, dried over anhydrous Na2SO4, filtered and solute evaporated under reduced pressure. After drying in vacuum, title compound (330 mg, 1.42 mmol) was obtained as yellow oil in 99% yield. IR 3378, 2099 cm-1. 1H NMR (200 MHz, CDCl3) δ = 7.61 (t, J = 7.7, 1H, Py), 7.29 (d, J = 7.7, 1H, Py), 7.14 (d, J = 7.6, 1H, Py), 4.37 (s, 2H, CH2N3), 3.95 (s, 1H, NH), 3.58 (s, 2H, CH2NCH2Py), 3.08–2.76 (m, 4H, CH2NCH2Py), 2.62–2.21 (m, 4H, CH2NH). 13C NMR (50 MHz, CDCl3) δ 158.5 (Py), 155.1 (Py), 137.3 (Py), 122.4 (Py), 120.3 (Py), 64.6 (PyCH2N), 55.5 (CH2N3), 53.5 (NCH2CH2N), 45.4 (NCH2CH2N). HRMS (ESI) calcd for C11H16N6 [M+H+] 233.15092, found 233.15009.
Synthesis of 1-{6-[(4-{[6-{azidomethyl}pyridin-2-yl]methyl}piperazin-1-yl)methyl]pyridin-2-yl}-methyl-4-methylpiperazine (11): Compound 4 (47 mg, 0.21 mmol) was added to SOCl2 (2 mL) at -15 °C under nitrogen atmosphere. After 45 min of stirring at -15 °C, H2O (2 mL) was added dropwise, followed by neutralization with saturated aqueous solution of Na2CO3. Mixture was extracted with DCM (3 x 5 mL) and combined organic fractions were dried over anhydrous Na2SO4 and filtered. Filtrate was concentrated under reduced pressure. Obtained chloride was dissolved in anhydrous ACN (3 mL) to which compound 8 (48 mg, 0.21 mmol) and anhydrous K2CO3 (20 mg, 0.14 mmol) were added under nitrogen atmosphere at -15 °C. Reaction mixture was heated to 50 °C and stirred for 1 h. Solid K2CO3 was filtered off and filtrate concentrated under reduced pressure. Title compound (20 mg, 0.046 mmol) was obtained in 22% yield from 8, after purification by column chromatography (CHCl3:MeOH:NH4OH, 100:35:3). IR 2100 cm-1. 1H NMR (400 MHz, CDCl3) δ = 7.68 (t, J = 7.8 Hz, 1H, Py), 7.61 (t, J = 7.6 Hz, 1H, Py), 7.40 (d, J = 7.6 Hz, 1H, Py), 7.3 (m, 2H, Py), 7.21 (d, J = 7.6 Hz, 1H, Py), 4.46 (s, 2H, CH2N3), δ = 3.68 (m, 6H,PyCH2N), 2.58–2.48 (m, 16H, NCH2CH2N), 2.30 (s, 3H, CH3). 13C NMR (50 MHz, CDCl3) δ = 159.0 (Py), 158.2 (Py), 158.0 (Py), 155.0 (Py), 137.3 (Py), 136.6 (Py), 122.3 (Py), 122.2 (Py), 121.2 (Py), 121.2 (Py), 120.2 (Py), 64.5 (PyCH2N), 64.4 (PyCH2N), 64.4 (PyCH2N), 55.7 (CH2N3), 55.1 (NCH2CH2N), 53.3 (NCH2CH2N), 53.3 (NCH2CH2N), 53.2 (NCH2CH2N), 46.0 (CH3) HRMS (ESI) calcd for C23H33N9 [M+H+] 436.29317, found 436.29141.
ACKNOWLEDGEMENTS
This work was supported by Quattromed HTI Laborid OÜ, Estonian Science Targeted Projects No SF 0180032s12, Estonian Science Council (IUT20-17) and the Estonian Science Foundation Grants no. 8794.
References
1. M. A. Sherlock, US4125612, 1978.
2. P. Alexanders, I. Byrjalsen, and C. Christiansen, Br. J. Obstet. Gynaecol., 2000, 107, 356. CrossRef
3. X. Wang, L. Wang, T. Li, Z. Huang, Y. Lai, H. Ji, X. Wan, J. Xu, J. Tian, and Y. Zhang, J. Med. Chem., 2013, 56, 3078. CrossRef
4. E. A. Amin and W. J. Welsh, J. Med. Chem., 2001, 44, 3849. CrossRef
5. R. Noir, M. Kotera, B. Pons, J.-S. Remy, and J.-P. Behr, J. Am. Chem. Soc., 2008, 130, 13500. CrossRef
6. Unpublished results, Molcode Ltd.
7. J. Ratilainen, K. Airola, E. Kolehmainen, and K. Rissanen, Chem. Ber./Recueil., 1997, 1353.
8. K. Soai, H. Oyamada, M. Takase, and A. Ookawa, Bull. Chem. Soc. Jpn., 1984, 57, 1948. CrossRef
9. B. Lewandowski and S. Jarosz, Org. Lett., 2010, 12, 2532. CrossRef
10. H. Su, C. Wu, J. Zhu, T. Miao, D. Wang, C. Xia, X. Zhao, Q. Gong, B. Song, and H. Ai, Dalton Trans., 2012, 41, 14480. CrossRef
11. M. Newcomb, J. M. Timko, D. M. Walba, and D. J. Cram, J. Am. Chem. Soc., 1977, 99, 6392. CrossRef
12. L. Díaz, J. Casas, J. Bujons, A. Llebaria, and A. Delgado, J. Med. Chem., 2011, 54, 2069. CrossRef
13. Y. S Jo, A. J. van der Vlies, J. Gantz, T. N. Thacher, S. Antonijevic, S. Cavadini, D. Demurtas, N. Stergiopulos, and J. A. Hubbell, J. Am. Chem. Soc., 2009, 131, 14413. CrossRef