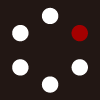
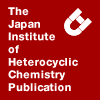
HETEROCYCLES
An International Journal for Reviews and Communications in Heterocyclic ChemistryWeb Edition ISSN: 1881-0942
Published online by The Japan Institute of Heterocyclic Chemistry
e-Journal
Full Text HTML
Received, 21st June, 2014, Accepted, 14th July, 2014, Published online, 17th July, 2014.
DOI: 10.3987/COM-14-S(K)40
■ Synthetic Study of Afritoxinone A: Stereoselective Construction of Furopyranone Moiety
Hideki Abe, Toshihiro Yoshie, Takumi Wagatsuma, Toyoharu Kobayashi, and Hisanaka Ito*
School of Life Sciences, Tokyo University of Pharmacy and Life Sciences, 1432-1 Horinouchi, Hachioji, Tokyo 192-0392, Japan
Abstract
Stereoselective construction of the furopyranone moiety of the natural product afritoxinone A was demonstrated. This synthetic methodology features (i) stereoselective 1,4-addition of a tricyclic ring system, (ii) oxidative cleavage of the diol, followed by hydration of the resulting dialdehyde group, and (iii) acetalization via cationic cyclization of the pyranediol with the tert-butyl ester tether.Afritoxinones A (1) and B (2) were isolated from the liquid culture of Diplodia africane, a fungal pathogen responsible for branch dieback of Phoenician junipers in Italy.1 In addition, structurally related
natural products, oxysporone (3),2-4 pestalotines A (4), and B (5),5 were isolated from Pestalotiopsis sp. HC02. These natural compounds contain a dihydrofuropyranone moiety in their structures, and possess phytotoxic activity. Although these structures are very simple, their total synthesis has not been reported to date. Furthermore, only a few reports on the construction of the perhydrofuropyranone skeleton, e.g., ring opening of cyclopropanated derivatives,6-9 lactonization via single electron transfer protocol,10 TiCl4 mediated combination of pyruvate ester and dihydropyran,11 and the enzymatic Baeyer–Villiger oxidation,12 are known. In this report, we describe the stereoselective construction of the dihydrofuropyranone moiety toward the total synthesis of natural product afritoxinone A (1).
Our synthetic plan for afritoxinone A (1), based on Diels–Alder/retro-Diels–Alder reaction concepts13 to construct the chiral carbons stereoselectively, is outlined in Scheme 1. The target natural product would be obtained by construction of the olefin group in the dihydrofuropyranone skeleton using the retro-Diels–Alder reaction of tetracyclic compound 6. Tetracyclic compound 6 would be constructed by the cationic dehydrocyclization of pyrandiol 7 having a tert-butyl ester moiety. Pyrandiol 7 would be synthesized by Rubottom oxidation of silyl enol ether 9, and reduction of the carbonyl group of the resulting α-hydroxy ketone 8, followed by oxidative cleavage of the diol group. The silyl enol ether 9 would be obtained by stereoselective introduction of the β-ketoester of tricyclic enone 10 from the concave face of the tricyclic ring system. Tricyclic compound 10 would be prepared from the known silyloxy enone 12 and pentamethylcyclopentadiene (11).
The investigation began by preparing tricyclic enone derivative 1015 as shown in Scheme 2. Diels–Alder reaction of the known cyclopentenone 12,16 prepared from furfuryl alcohol in two steps, with 1,2,3,4,5-pentamethylcyclopentadiene (11) in refluxing toluene gave tricyclic adduct 13 in 66% yield. Treatment of 13 with tetra-n-butylammonium fluoride afforded the tricyclic enone 10 as a mixture of two diastereoisomers (dr = 10:1) at C8 position in 95% yield. Stereoselective 1,4-addition reaction17 of the tricyclic enone 10 was achieved by use of TMSOTf as a Lewis acid and Reformatsky reagent, prepared from tert-butyl bromoacetate and Zn powder, to yield alkylated ketone 14 as a single isomer at the β position. Although the stereochemistry at the β position of 14 was not confirmed at this stage, it is assumed to be that depicted in Scheme 2.
Introduction of the hydroxyl group at the α position of the carbonyl group, carried out in a two-step operation, involved formation of a silyl enol ether (63%), followed by Rubottom oxidation18 of the resulting silyl enol ether to give α-hydroxy ketone 8 in 56% yield as shown in Scheme 3. Although assignment of the stereochemistry of the asymmetric carbon at the carbonyl α position was not performed, it was proposed that the hydroxyl group approached the convex side since mCPBA approached the double bond from the convex side. Reduction of the carbonyl group in 8 gave diol 15 in 95% yield. X-ray crystallographic analysis of diol 1519 indicated that the stereochemistry of the newly constructed asymmetric carbon was 1R*,2R*,3S* as depicted in Scheme 3. These results indicate that the three reactions, nucleophilic attack of Reformatsky reagent to the β position of enone 10, Rubottom oxidation with mCPBA of the silyl enol ether 9, and hydride reduction of ketone 8 with NaBH4, occurred from the concave side.
With the diol derivative in hand, our attention turned to the construction of the furopyranone moiety. Oxidative glycol cleavage of the 1,2-dihydroxyl moiety in 15 with sodium periodate in THF–H2O afforded tetrahydropyranediol derivative 7 as a mixture of two diastereoisomers, which was a hydrated
compound of the dialdehyde produced by glycol cleavage. Construction of the target furopyranone skeleton was accomplished by dehydrocondensation of 7 under acidic conditions in MeOH to give the tetracyclic furopyranone derivative 6 in 85% yield as a single diastereoisomer. The stereochemistry of tetracyclic compound 6 was determined by X-ray crystallographic analysis as depicted in Scheme 4.20 The relative configuration between C3a and C9b of tetracyclic compound 6 is cis, which is identical to that of afritoxinone A (1).
Finally, completion of our project by reconstruction of the olefin group and epimerization at C5 position was attempt. Unfortunately, cleavage of the pentamethylcyclopentadiene ring for deprotection of the protected double bond by retro-Diels–Alder reaction under several conditions [heated solvent (e.g., xylene, diphenylether) with/without a diene compound (e.g., maleic anhydride)] did not proceed.21 Thus, our main goal, the total synthesis of afritoxinone A (1), could not be completed.
In conclusion, we demonstrated the stereoselective construction of the furopyranone moiety in the natural product afritoxinone A (1). This synthetic methodology involving stereoselective 1,4-addition of Reformatsky reagent with the tricyclic enone, oxidative cleavage of the diol, and acetalization of the pyranediol with the tert-butyl ester tether under acidic conditions is a useful method for the stereoselective synthesis of natural products afritoxinones and pestalotines having the furopyranone skeleton. Synthetic projects of these natural products are now in progress in our laboratory.
EXPERIMENTAL
(3aR*,4R*,7R*,7aS*,8R*)-3-(tert-Butyldimethylsilyloxy)-4,5,6,7,8-pentamethyl-2,3,3a,4,7,7a-hexahydro-1H-4,7-methanoinden-1-one (13):
A solution of the enone 12 (5.00 g, 23.5 mmol) and 1,2,3,4,5-pentamethylcyclopentadiene (11) (7.6 mL, 6.41 g, 47.1 mmol) in toluene (78 mL) was refluxed for 3 h. After the solvent was removed in vacuo, the resulted residue was purified by silica gel column chromatography (hexane–AcOEt, 15:1) to give 13 (5.41 g, 66%) as pale yellow oil.
IR (neat) 2953, 1731, 1471, 1454, 1378, 1252, 1161, 1093, 1056, 926, 835, 776 cm–1; 1H NMR (400 MHz, CDCl3) δ 0.03 (3H, s), 0.06 (3H, s), 0.52 (3H, d, J = 6.4 Hz), 0.86 (9H, s), 1.18 (3H, s), 1.19 (3H, s), 1.37–1.43 (4H, m), 1.56 (3H, s), 1.90–2.04 (2H, m), 2.55–2.61 (2H, m), 4.02 (1H, dd, J = 5.9, 1.7 Hz); 13C NMR (100 MHz, CDCl3) δ –4.7 (2C), 7.0, 11.2, 12.1, 14.5, 14.5, 17.9, 25.7 (3C), 50.5, 55.9, 58.4, 59.2, 62.2, 64.6, 68.9, 132.6, 134.0, 219.8. HRESIMS calcd for C21H37O2Si [M+H]+ 349.2563, found 349.2567.
(3aR*,4S*,7R*,7aS*,8R*)-4,5,6,7,8-Pentamethyl-3a,4,7,7a-tetrahydro-1H-4,7-methanoinden-1-one (10):
To a solution of the adduct 13 (5.41 g, 15.5 mmol) in THF (155 mL) was added tetra-n-butylammonium fluoride (1.0 M in THF solution, 18.6 mL, 18.6 mmol) at 0 °C, and the mixture was stirred at room temperature for 2 h. After the reaction mixture was quenched by addition with water, the mixture was extracted with AcOEt. The combined organic layer was washed with brine, dried over MgSO4, and the solvent was removed. The resulted residue was purified by silica gel column chromatography (hexane–AcOEt, 20:1) to give 1015 (3.19 g, 95%) as pale yellow oil.
IR (neat) 2954, 2925, 2868, 1696, 1439, 1377, 1184, 1162, 1066, 912, 744 cm–1; 1H NMR (400 MHz, CDCl3) δ 0.59 (3H, d, J = 6.4 Hz), 1.15 (3H, s), 1.24 (3H, s), 1.34 (3H, s), 1.44 (3H, s), 1.62 (1H, q, J = 6.4 Hz), 2.33 (1H, d, J = 5.3 Hz), 3.08 (1H, ddd, J = 5.3, 2.6, 1.4 Hz), 5.88 (1H, dd, J = 5.7, 1.4 Hz), 7.39 (1H, dd, J = 5.7, 2.6 Hz); 13C NMR (100 MHz, CDCl3) δ 8.1, 11.1, 11.2, 14.5, 14.7, 56.0, 56.5, 57.1, 57.2, 65.8, 131.3, 132.6, 136.3, 163.1, 210.5. HRESIMS calcd for C15H21O [M+H]+ 217.1592, found 217.1597.
tert-Butyl 2-((1R*,3aS*,4R*,7S*,7aR*,8R*)-4,5,6,7,8-pentamethyl-3-oxo-2,3,3a,4,7,7a-hexahydro-1H-4,7-methanoinden-1-yl)acetate (14):
To a suspention of Reformatsky reagent, prepared from tert-butyl bromoacetate (1.36 mL, 1.80 g, 9.25 mmol) and zinc dust (605 mg, 9.25 mmol) in THF (10 mL), were added dropwise a solution of enone 10 (500 mg, 2.31 mmol) in THF (23 mL) and trimethylsilyl trifluoromethanesulfonate (1.67 mL, 2.05 g, 9.25 mmol) at –78 °C. After stirring for 2 h at –78 °C, the reaction was quenched by addition of saturated NaHCO3 aqueous solution, and the mixture was extracted with AcOEt, washed with brine, and dried over MgSO4. After the solvent was removed in vacuo, the resulted residue was purified by silica gel column chromatography (hexane–AcOEt, 15:1) to give 14 (677 mg, 92%) as colorless oil.
IR (neat) 2954, 2927, 2871, 1732, 1454, 1367, 1254, 1154, 1073, 951, 845 cm–1; 1H NMR (400 MHz, CDCl3) δ 0.52 (3H, d, J = 6.4 Hz), 1.13 (3H, s), 1.20 (3H, s), 1.37 (1H, q, J = 6.4 Hz), 1.42–1.46 (12H, m), 1.63 (3H, s), 1.78–1.84 (1H, m), 1.98–2.05 (1H, m), 2.16–2.22 (2H, m), 2.24–2.27 (2H, m), 2.49 (1H, br d, J = 8.4 Hz); 13C NMR (100 MHz, CDCl3) δ 7.2, 11.3, 12.3, 14.3, 14.5, 28.0 (3C), 30.7, 43.7, 46.1, 56.8, 57.0, 58.7, 59.7, 64.4, 80.6, 133.3, 133.9, 171.4, 220.8. HRESIMS calcd for C21H32O3Na [M+Na]+ 355.2249, found 355.2253.
tert-Butyl 2-((1R*,3aS*,4R*,7S*,7aR*,8R*)-4,5,6,7,8-pentamethyl-3-(trimethylsilyloxy)-3a,4,7,7a-tetrahydro-1H-4,7-methanoinden-1-yl)acetate (9):
To a solution of ketone 14 (4.12 g, 12.4 mmol) in THF (62 mL) was added LHMDS (1.0 M in THF, 24.8 mL, 24.8 mmol) at –78 °C, and stirred for 0.5 h at –78 °C. To this mixture was added dropwise trimethylsilylchloride (2.35 mL, 2.02 g, 18.6 mmol) at –78 °C, and stirred for 0.5 h at the same temperature. The reaction was quenched by addition of saturated NaHCO3 aqueous solution, and the mixture was extracted with AcOEt, washed with brine, and dried over MgSO4. After the solvent was removed in vacuo, the resulted residue was purified by silica gel column chromatography (hexane–AcOEt, 10:1) to give 9 (3.18 g, 63%) as colorless oil.
IR (neat) 2961, 1731, 1639, 1456, 1367, 1324, 1253, 1213, 1146 cm–1; 1H NMR (400 MHz, C6D6) δ 0.14 (9H, s), 0.65 (3H, d, J = 6.4 Hz), 1.11 (3H, s), 1.16 (1H, q, J = 6.4 Hz), 1.27 (3H, s), 1.41 (9H, s), 1.64 (3H, s), 1.66 (3H, s), 1.96 (1H, dd, J = 8.4, 2.4 Hz), 2.16–2.19 (2H, m), 2.54–2.60 (1H, m), 2.73 (1H, ddd, J = 8.4, 2.9, 1.1 Hz), 4.48–4.50 (1H, m); 13C NMR (100 MHz, C6D6) δ –0.2 (3C), 8.2, 11.7, 13.0, 14.9, 15.4, 28.2 (3C), 39.1, 45.2, 56.0, 56.2, 57.4, 59.5, 63.7, 79.3, 107.0, 131.9, 134.1, 156.8, 171.8. HRESIMS calcd for C24H40O3NaSi [M+Na]+ 427.2644, found 427.2642.
tert-Butyl 2-((1R*,2R*,3aS*,4R*,7S*,7aR*,8R*)-2-hydroxy-4,5,6,7,8-pentamethyl-3-oxo-2,3,3a,4,7,7a-hexahydro-1H-4,7-methanoinden-1-yl)acetate (8):
To a solution of silyl enol ether 9 (50.0 mg, 0.124 mmol) in CH2Cl2 (1 mL) was added NaHCO3 (32.3 mg, 0.384 mmol) and m-chloroperbenzoic acid (29.7 mg, 0.133 mmol) at 0 °C, and the mixture was stirred at the same temperature. After stirring for 1 h, the reaction was quenched by addition of saturated NaHCO3 aqueous solution, and the mixture was extracted with CHCl3, washed with brine, and dried over MgSO4. After the solvent was removed in vacuo, the resulted residue was purified by silica gel column chromatography (hexane–AcOEt, 10:1) to give 8 (23.9 mg, 56%) as colorless oil.
IR (neat) 3444, 2955, 2928, 2871, 1738, 1732, 1715, 1454, 1392, 1379, 1367, 1334, 1256, 1150, 1087 cm–1; 1H NMR (400 MHz, CDCl3) δ 0.52 (3H, d, J = 6.4 Hz), 1.13 (3H, s), 1.15 (3H, s), 1.36 (3H, s), 1.37–1.42 (10H, m, including 9H, s, at δ 1.40), 1.63 (3H, s), 1.99 (1H, dd, J = 15.7, 8.0 Hz), 2.22 (1H, d, J = 8.8 Hz), 2.36 (1H, dd, J = 15.7, 5.6 Hz), 2.46–2.59 (3H, m), 3.51 (1H, d, J = 9.4 Hz); 13C NMR (100 MHz, CDCl3) δ 7.6, 11.2, 12.3, 14.2, 14.6, 28.0 (3C), 34.4, 36.7, 54.5, 56.8 (2C), 59.2, 64.5, 76.6, 80.6, 134.1, 134.8, 172.5, 218.7. HRESIMS calcd for C21H32O4Na [M+Na]+ 371.2198, found 371.2213.
tert-Butyl 2-((1R*,2R*,3S*,3aS*,4R*,7S*,7aR*,8R*)-2,3-dihydroxy-4,5,6,7,8-pentamethyl-2,3,3a,4,7,7a-hexahydro-1H-4,7-methanoinden-1-yl)acetate (15):
To a solution of α-hydroxy ketone 8 (379 mg, 1.09 mmol) in MeOH (10 mL) was added sodium borohydride (49.4 mg, 1.31 mmol) at 0 °C, and the mixture was stirred for 0.5 h at the same temperature. After the mixture was quenched by addition of saturated NaHCO3 aqueous solution, and the mixture was extracted with Et2O, washed with brine, and dried over MgSO4. After the solvent was removed in vacuo, the resulted residue was purified by silica gel column chromatography (hexane–AcOEt, 2:1) to give 15 (364 mg, 95%) as colorless prism.
Mp 132–134 °C (benzene–hexane); IR (KBr) 3390, 2951, 2924, 2870, 1732, 1704, 1449, 1368, 1256, 1155, 1114, 1098, 1069, 1029 cm–1; 1H NMR (400 MHz, C6D6) δ 0.58 (3H, d, J = 6.4 Hz), 1.09 (3H, s), 1.15 (1H, q, J = 6.4 Hz), 1.19 (3H, s), 1.27–1.33 (1H, br s), 1.40 (9H, s), 1.42–1.48 (1H, br s), 1.56 (3H, s), 1.74 (3H, s), 1.86–2.00 (2H, m), 2.13 (1H, dd, J = 9.1, 9.0 Hz), 2.17–2.23 (1H, m), 2.63 (1H, dd, J = 15.4, 6.4 Hz), 3.53 (1H, dd, J = 8.7, 7.5 Hz), 3.85 (1H, dd, J = 9.0, 8.7 Hz); 13C NMR (100 MHz, C6D6) δ 7.8, 12.1, 12.7, 15.2, 16.2, 28.2 (3C), 36.9, 37.6, 52.7, 56.4, 56.5, 58.2, 64.8, 78.7, 79.8, 79.9, 134.1, 136.3, 173.5.
HRESIMS calcd for C21H35O4 [M+H]+ 351.2535, found 351.2535.
tert-Butyl 2-((4R*,4aR*,5S*,8R*,8aS*,9R*)-1,3-dihydroxy-5,6,7,8,9-pentamethyl-3,4,4a,5,8,8a-hexahydro-1H-5,8-methanoisochromen-4-yl)acetate (7):
To a solution of 15 (364 mg, 1.04 mmol) in THF–H2O (2:1, 15 mL) was added sodium periodate (444 mg, 2.08 mmol) at 0 °C, and the mixture was stirred for 3 h at room temperature. After the reaction mixture was diluted with H2O, the mixture was extracted with Et2O. The combined organic layer was washed with brine, dried over MgSO4, and concentrated in vacuo. The resulting residue was purified by silica gel column chromatography (hexane–AcOEt, 1:1) to give 7 (287 mg, 75%, mixture of two diastereomers, dr = 2:1) as colorless oil.
IR (neat) 3425, 2957, 2929, 2873, 1728, 1448, 1367, 1154, 1021 cm–1; 1H NMR (300 MHz, CDCl3, major isomer) δ 0.55 (3H, d, J = 6.4 Hz), 1.12 (3H, s), 1.14 (3H, s), 1.35 (1H, q, J = 6.4 Hz), 1.44 (9H, s), 1.59–1.70 (7H, m, including 3H, s, at δ 1.60, and 3H, s, at δ 1.62), 1.85–2.06 (2H, m), 2.23 (1H, dd, J = 15.9, 8.4 Hz), 2.69 (1H, dd, J = 15.9, 2.3 Hz), 3.16 (1H, d, J = 6.1 Hz), 3.72 (1H, d, J = 5.4 Hz), 4.78 (1H, dd, J = 7.8, 6.1 Hz), 5.01 (1H, dd, J = 8.6, 5.4 Hz); 13C NMR (75 MHz, CDCl3, major isomer) δ 7.5, 12.2, 12.5, 14.9, 16.0, 28.1 (3C), 38.1, 38.9, 48.6, 53.8, 54.6, 56.3, 62.3, 80.7, 92.9, 96.1, 133.8, 134.4, 172.6. HRESIMS calcd for C21H34O5Na [M+Na]+ 389.2304, found 389.2299.
(3aR*,5R*5aS*,6R*,9R*,9aR*,9bR*,10R*)-5-Methoxy-6,7,8,9,10-pentamethyl-5,5a,6,9,9a,9b-hexahydro-1H-6,9-methanofuro[2,3-c]isochromen-2(3aH)-one (6):
To a solution of 7 (287 mg, 0.783 mmol) in MeOH (8 mL) was added p-toluenesulfonic acid monohydrate (46.8 mg, 0.246 mmol) at room temperature, and the mixture was stirred for 0.5 h at the same temperature. After the solvent was removed in vacuo, the resulting residue was purified by silica gel column chromatography (hexane–AcOEt, 5:1) to give 6 (205 mg, 85%) as colorless needle.
Mp 125–127 °C (benzene–hexane); IR (KBr) 2955, 2917, 2870, 1785, 1448, 1380, 1366, 1333, 1262, 1188, 1116, 1058, 982, 957 cm–1; 1H NMR (600 MHz, C6D6) δ 0.47 (3H, d, J = 6.4 Hz), 0.70 (3H, s), 0.82 (3H, s), 0.91 (1H, q, J = 6.4 Hz), 1.26 (3H, s), 1.44 (3H, s), 1.56 (1H, d, J = 10.1 Hz), 1.78 (1H, d, J = 10.1 Hz), 1.80 (1H, ddd, J = 10.9, 10.1, 7.8 Hz), 2.09 (1H, dd, J = 17.6, 10.9 Hz), 2.67 (1H, dd, J = 17.6, 10.1 Hz), 3.28 (3H, s), 4.46 (1H, s), 4.98 (1H, d, J = 7.8 Hz); 13C NMR (150 MHz, C6D6) δ 7.3, 12.0, 12.2, 13.3, 14.0, 29.2, 35.9, 45.4, 46.6, 55.1, 55.5, 55.8, 63.0, 97.6, 98.0, 131.3, 135.0, 174.8. HRESIMS calcd for C18H26O4Na [M+Na]+ 329.1729, found 329.1724.
ACKNOWLEDGEMENTS
This work was supported in part by a Grant-in-Aid for Platform for Drug Discovery, Informatics, and Structural Life Science, and a Grant-in-Aid for Scientific Research (C) (No. 26460016) from the Ministry of Education, Culture, Sports, Science and Technology, Japan.
References
1. A. Evidente, M. Masi, B. T. Linaldeddu, A. Franceschini, B. Scanu, A. Cimmino, A. Andolfi, A. Motta, and L. Maddau, Phytochemistry, 2012, 77, 245. CrossRef
2. E. K. Adesogan and B. I. Alo, Phytochemistry, 1979, 18, 1886. CrossRef
3. T. Nagata and Y. Ando, Agric. Biol. Chem., 1989, 53, 2811. CrossRef
4. P. Venkatasubbaiah, C. G. Van Dyke, and W. S. Chilton, Phytochemistry, 1991, 30, 1471. CrossRef
5. Y. L. Zhang, H. M. Ge, F. Li, Y. C. Song, and R. X. Tan, Chem. Biodiver., 2008, 5, 2402. CrossRef
6. S. D. Haveli, P. R. Sridhar, P. Suguna, and S. Chandrasekaran, Org. Lett., 2007, 9, 1331. CrossRef
7. O. Temme, S.-A. Taj, and P. G. Andersson, J. Org. Chem., 1998, 63, 6007. CrossRef
8. M. del Rosario-Chow, J. Ungwitayatorn, and B. L Currie, Tetrahedron Lett., 1991, 32, 1011. CrossRef
9. E. Wenkert, T. D. J. Halls, L. D. Kwart, G. Magnusson, and H. D. H. Showalter, Tetrahedron, 1981, 37, 4017. CrossRef
10. A. D’Annibale and C. Trogolo, Tetrahedron Lett., 1994, 35, 2083. CrossRef
11. M. C. Wills and S. E. Flower, Synlett, 2003, 1491. CrossRef
12. F. Petit and R. Furstoss, Tetrahedron: Asymmetry, 1993, 4, 1341. CrossRef
13. S. Kotha and S. Banerjee, RSC Adv., 2013, 3, 7642.
14. A. J. H. Klunder, J. Zhu, and B. Zwanenburg, Chem. Rev., 1999, 99, 1163. CrossRef
15. P. A. Grieco and N. Abood, J. Chem. Soc., Chem. Commun., 1990, 410. CrossRef
16. T. T. Curran, D. A. Hay, and C. P. Koegel, Tetrahedron, 1997, 53, 1983. CrossRef
17. S. Kim, W. S. Han, and J. M. Lee, Bull. Korean Chem. Soc., 1992, 13, 466. CrossRef
18. G. M. Rubottom, M. A. Vazquez, and D. R. Pelegrina, Tetrahedron Lett., 1974, 15, 4319. CrossRef
19. CCDC 1004142 contains the supplementary crystallographic data of 15 for this paper. These data can be obtained free of charge from the Cambridge Crystallographic Data Centre via www.ccdc.cam.ac.uk/data_request/cif.
20. CCDC 1006210 contains the supplementary crystallographic data of 6 for this paper. These data can be obtained free of charge from the Cambridge Crystallographic Data Centre via www.ccdc.cam.ac.uk/data_request/cif.
21. In most cases, the starting material 6 was recovered. The retro-Diels–Alder reaction of the diol 15 was also attempted in the same conditions. These examinations resulted in decomposition of 15.