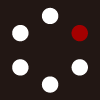
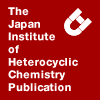
HETEROCYCLES
An International Journal for Reviews and Communications in Heterocyclic ChemistryWeb Edition ISSN: 1881-0942
Published online by The Japan Institute of Heterocyclic Chemistry
e-Journal
Full Text HTML
Received, 30th June, 2014, Accepted, 30th July, 2014, Published online, 8th August, 2014.
DOI: 10.3987/COM-14-S(K)60
■ Synthesis of α-Halobutenolides Using the Nucleophilicity of Magnesium Alkylidene Carbenoids
Tsutomu Kimura,* Kazuki Fukuda, Gaku Kashiwamura, and Tsuyoshi Satoh*
Tokyo University of Science, Kagurazaka 1-3, Shinjuku-ku, Tokyo 165-0035, Japan
Abstract
α-Halobutenolides were synthesized from halomethyl p-tolyl sulfoxides, α-bromoketones, and phenyl chloroformate in three steps using the nucleophilicity of magnesium alkylidene carbenoids. The reaction of α-bromoketones with [halo(p-tolylsulfinyl)methyl]lithiums and the subsequent basification of the reaction mixture using an aqueous NaOH solution afforded 1-chloro-3-hydroxyprop-1-enyl p-tolyl sulfoxides in 83–99% yield. A phenoxycarbonyl group was then introduced to the hydroxyl group of the sulfoxides by reacting with phenyl chloroformate in the presence of pyridine. The sulfoxide/magnesium exchange reaction of the cyclization precursors with i-PrMgCl•LiCl led to the formation of α-halobutenolides in moderate to good yields.α,β-Unsaturated γ-lactones, also referred to as butenolides and furan-2(5H)-ones, are a fundamental class of heterocyclic compounds. The butenolide motif is present in many natural products, and the synthesis and synthetic utility of butenolides have been studied in great depth.1 Among the butenolides, α-halobutenolides play an important role in organic synthesis (Figure 1). α-Bromobutenolides are used for the synthesis of α-arylbutenolides, including the naturally occurring eutypoid A, microperfuranone, gymnoascolide A,2 and anti-inflammatory drug rofecoxib3 via the cross-coupling reaction. α-(1-Hydroxyalkyl)butenolides isolated from the fermentation of TÜ994 and seed germination stimulants5 are synthesized from α-halobutenolides via the halogen/lithium exchange reaction and the subsequent reaction of the resulting α-lithiated butenolides with electrophiles. Furthermore, an α-halobutenolide skeleton is found in rubrolides6 and mutagen X,7 which is a contaminant in chlorinated water.8 The typical synthetic method of α-halobutenolides consists of constructing a multi-substituted α,β-unsaturated lactone ring in several steps and a halogenation-dehydrohalogenation process. The development of a novel method for the synthesis of α-halobutenolides from readily available materials in short steps with a de novo lactone ring formation is expected to contribute to the further advancement of the above chemistry.
Magnesium alkylidene carbenoids are a class of organomagnesium compounds in which magnesium and halogen atoms are attached to a vinylic carbon atom.9 Intriguingly, magnesium alkylidene carbenoids act as electrophiles toward the carbon and heteroatom nucleophiles.10 We have reported the synthesis of multi-substituted alkenes using the electrophilicity of magnesium alkylidene carbenoids.11 However, the synthetic applications of the nucleophilicity of magnesium alkylidene carbenoids have not been fully explored.12,13 We recently found that magnesium carbenoids with an electrophilic functional group, such as cyano and (phenoxycarbonyl)oxy groups, can be generated from the corresponding 1-chloroalkyl p-tolyl sulfoxides via the sulfoxide/magnesium exchange reaction.14 The resulting magnesium carbenoids act as nucleophiles toward the intramolecular electrophilic functional group to yield cyclized products. If magnesium alkylidene carbenoids with the [(phenoxycarbonyl)oxy]methyl group can be generated, the resulting carbenoids would cyclize to give α-halobutenolides (Scheme 1). Herein, we report a novel synthetic method of α-halobutenolides using the nucleophilicity of magnesium alkylidene carbenoids.
We designed cyclization precursors 4 consisting of a 1-chlorovinyl p-tolyl sulfoxide unit and a phenyl carbonate unit (Scheme 1). Therefore, the 1-chloro-3-hydroxyprop-1-enyl p-tolyl sulfoxides 3 are key synthetic intermediates. Inspired by the Darzens reaction,15 we developed a useful method for the synthesis of sulfoxides 3 from halomethyl p-tolyl sulfoxides 1 and α-bromoketones 2 (Table 1).16 For instance, chloromethyl p-tolyl sulfoxide (1a) was treated with LDA in THF at –78 °C, and 1-bromo-4-phenylbutan-2-one (2a) was added to the reaction mixture (entry 1). The reaction mixture was then basified using an aqueous NaOH solution. As a result, sulfoxide 3a was formed as a 1:1 mixture of geometric isomers in 99% yield. The reaction seems to proceed as follows. Nucleophilic addition of [chloro(p-tolylsulfinyl)methyl]lithium to α-bromoketone 2a gives the adduct, and the subsequent nucleophilic substitution of the resulting lithium alkoxide with an intramolecular bromomethyl unit gives β,γ-epoxy sulfoxide. The addition of an aqueous NaOH solution to the reaction mixture containing β,γ-epoxy sulfoxide results in β-elimination, to give sulfoxide 3a.17 A diverse range of sulfoxides 3b–g with an alkyl or aryl substituent at the 2-position could be prepared from sulfoxide 1a and the corresponding α-bromoketones 2b–g in 83–98% yield (entries 2–7). The synthetic method was applicable to the synthesis of bromo analog 4h (entry 8). The reaction of sulfoxides 3 with phenyl chloroformate in the presence of pyridine yielded cyclization precursors 4 with high efficiency.18
With the key cyclization precursors 4 in hand, we attempted the sulfoxide/metal exchange reaction leading to the formation of α-halobutenolides 5. The choice of organometallic reagent for the reaction is a key issue because the sulfoxide/metal exchange reaction of the organometallic reagent with the sulfoxide unit should take precedence over the reaction of the organometallic reagent with the phenyl carbonate unit for the generation of bifunctional (nucleophilic/electrophilic) chemical species.19 In addition, the organometallic reagent must be unreactive to the α-halobutenolides 5 as products. We examined the reaction of sulfoxide 4a with organometallic reagents, including BuLi, i-PrMgCl, i-PrMgBr, i-PrMgCl•LiCl, and c-C5H9MgCl, under various reaction conditions and found that i-PrMgCl•LiCl (turbo Grignard reagent) was the reagent of choice for our purpose.20 Sulfoxide 4a was treated with i-PrMgCl•LiCl in THF at 0 °C, and the reaction mixture was stirred at room temperature for 30 min (Table 2, entry 1).21 The desired α-chlorobutenolide 5a was obtained in 76% yield. When the reaction was conducted with each of the geometric isomers (E)-4a and (Z)-4a, α-chlorobutenolide 5a was obtained in 78% and 74% yield, respectively (entries 2 and 3). Because the reaction with both (E)- and (Z)-isomers gave α-chlorobutenolide 5a in good yields, the substrate scope was explored using a mixture of geometric isomers. A variety of β-alkyl- and β-aryl-substituted α-chlorobutenolides 5b–g were obtained in 41–65% yield (entries 4–9). In all cases, vinyl chlorides, which originated from the protonation of unreacted magnesium alkylidene carbenoids, were formed as side products in 10–46% yield. The reaction of 2-methyl-substituted sulfoxide 4b with i-PrMgCl•LiCl afforded α-chloro-β-methylbutenolide 5b in 33% yield, and the use of i-PrMgCl instead of i-PrMgCl•LiCl improved the reaction efficiency (entry 4). It is substantially important to use i-PrMgBr for the reaction with bromo analog 4h (entry 10). Otherwise, both α-bromobutenolide 5h and α-chlorobutenolide 5a were formed in 30% and 21% yield, respectively.12 Total synthesis of rubrolides L and M has been achieved using α-chlorobutenolide 4d with a 4-methoxyphenyl group at the β-position as a key synthetic intermediate via vinylogous aldol condensation (Figure 1).6
A plausible reaction mechanism for the formation of α-halobutenolides 5 is depicted in Scheme 2. Sulfoxide/magnesium exchange reaction of sulfoxides 4 with i-PrMgCl•LiCl generates magnesium alkylidene carbenoids. Magnesium alkylidene carbenoids generated from (E)-isomers (E)-4 can react with an intramolecular carbonyl group to give α-halobutenolides 5. However, magnesium alkylidene carbenoids generated from (Z)-isomers (Z)-4 are not expected to cyclize because the magnesium atom and the [(phenoxycarbonyl)oxy]methyl group are located trans to each other. Nevertheless, the reaction with (Z)-isomer gave α-chlorobutenolide (Table 2, entry 3). This result suggests that the geometry of the magnesium alkylidene carbenoids isomerizes gradually. In fact, geometric isomerization of magnesium alkylidene carbenoids is known to occur even at –78 °C.12
In summary, we developed a novel method for the synthesis of α-halobutenolides from readily available materials in three steps. Cyclization precursors were prepared from α-bromoketones, halomethyl p-tolyl sulfoxides, and phenyl chloroformate with high efficiency, and the chemoselective sulfoxide/magnesium exchange reaction of the cyclization precursors with i-PrMgCl•LiCl led to the formation of α-halobutenolides. Further investigation of the synthetic applications of magnesium alkylidene carbenoids using their nucleophilicity is currently ongoing and will be reported in due course.
ACKNOWLEDGEMENTS
This work was supported by JSPS KAKENHI Grant Number 25810030 (T.K.), MEXT KAKENHI Grant Number 22590021 (T.S.), and a TUS Grant for Research Promotion from the Tokyo University of Science, which are gratefully acknowledged.
SUPPORTING INFORMATION
General methods and characterization data for cyclization precursors 4 and α-halobutenolides 5 are available.
References
1. Y. S. Rao, Chem. Rev., 1976, 76, 625; CrossRef D. W. Knight, Contemp. Org. Synth., 1994, 1, 287; CrossRef A. Hashem and E. Kleinpeter, Adv. Heterocycl. Chem., 2001, 81, 107; CrossRef N. B. Carter, A. E. Nadany, and J. B. Sweeney, J. Chem. Soc., Perkin Trans. 1, 2002, 2324; CrossRef M. V. N. De Souza, Mini-Rev. Org. Chem., 2005, 2, 139; CrossRef S. Singh, P. K. Sharma, N. Kumar, and R. Dudhe, Pharm. Sci. Monitor, 2011, 2, 51.
2. A. N. Parker, M. J. Lock, and J. M. Hutchison, Tetrahedron Lett., 2013, 54, 5322. CrossRef
3. R. Rossi, F. Bellina, and E. Raugei, Synlett, 2000, 1749.
4. G. Grossmann, M. Poncioni, M. Bornand, B. Jolivet, M. Neuburger, and U. Séquin, Tetrahedron, 2003, 59, 3237. CrossRef
5. K. Sun, Y. Chen, T. Wagerle, D. Linnstaedt, M. Currie, P. Chmura, Y. Song, and M. Xu, Tetrahedron Lett., 2008, 49, 2922. CrossRef
6. S. Miao and R. J. Andersen, J. Org. Chem., 1991, 56, 6275; CrossRef F. Bellina, C. Anselmi, and R. Rossi, Tetrahedron Lett., 2002, 43, 2023; CrossRef J. Boukouvalas and L. C. McCann, Tetrahedron Lett., 2010, 51, 4636. CrossRef
7. R. T. LaLonde, H. Perakyla, and M. P. Hayes, J. Org. Chem., 1990, 55, 2847; CrossRef R. T. LaLonde, L. Bu, A. Henwood, J. Fiumano, and L. Zhang, Chem. Res. Toxicol., 1997, 10, 1427. CrossRef
8. V. M. Dembritskii and G. A. Tolstikov, Khim. Interesakh Ustoich. Razvit., 2003, 11, 699.
9. T. Satoh, Chem. Rec., 2004, 3, 329; CrossRef T. Satoh, 'The Chemistry of Organomagnesium Compounds,' ed. by Z. Rappoport and I. Marek, John Wiley & Sons Ltd., Chichester, UK, 2008, pp. 717–769; CrossRef T. Satoh, Heterocycles, 2012, 85, 1; CrossRef T. Satoh and T. Kimura, J. Synth. Org. Chem. Jpn., 2013, 71, 1033; CrossRef T. Kimura, 'Density Functional Theory: Principles, Applications and Analysis,' ed. by J. Morin and J. M. Pelletier, Nova Science Publishers, Inc., Hauppauge, NY, 2013, pp. 59–93.
10. T. Kimura and T. Satoh, J. Organomet. Chem., 2012, 715, 1; CrossRef T. Kimura and T. Satoh, Tetrahedron, 2013, 69, 6371; CrossRef T. Kimura and T. Satoh, Tetrahedron Lett., 2013, 54, 5072. CrossRef
11. T. Satoh, T. Sakamoto, M. Watanabe, and K. Takano, Chem. Pharm. Bull., 2003, 51, 966; CrossRef M. Watanabe, M. Nakamura, and T. Satoh, Tetrahedron, 2005, 61, 4409; CrossRef T. Satoh, Y. Ogino, and K. Ando, Tetrahedron, 2005, 61, 10262; CrossRef J. Sakurada and T. Satoh, Tetrahedron, 2007, 63, 3806; CrossRef N. Mori, K. Obuchi, T. Katae, J. Sakurada, and T. Satoh, Tetrahedron, 2009, 65, 3509; CrossRef T. Satoh, H. Kaneta, A. Matsushima, and M. Yajima, Tetrahedron Lett., 2009, 50, 6280; CrossRef T. Kimura, G. Kobayashi, M. Ishigaki, M. Inumaru, J. Sakurada, and T. Satoh, Synthesis, 2012, 44, 3623; CrossRef T. Kimura, M. Watanabe, G. Kashiwamura, J. Sakurada, and T. Satoh, Synthesis, 2013, 45, 659. CrossRef
12. T. Satoh, K. Takano, H. Ota, H. Someya, K. Matsuda, and M. Koyama, Tetrahedron, 1998, 54, 5557. CrossRef
13. V. A. Vu, I. Marek, and P. Knochel, Synthesis, 2003, 1797.
14. H. Saitoh, T. Sampei, T. Kimura, Y. Kato, N. Ishida, and T. Satoh, Tetrahedron Lett., 2012, 53, 3004; CrossRef T. Kimura, J. Nishida, G. Kashiwamura, G. Kobayashi, and T. Satoh, Tetrahedron Lett., 2014, 55, 1428. CrossRef
15. M. Ballester, Chem. Rev., 1955, 55, 283. CrossRef
16. Typical procedure: A 1.65 M solution of BuLi in hexane (2.18 mL, 3.60 mmol) was added to a solution of i-Pr2NH (364 mg, 3.60 mmol) in THF (40 mL) at 0 °C, and the mixture was stirred at 0 °C for 10 min. A solution of 1a (683 mg, 3.60 mmol) in THF (4.0 mL) was added to the solution of LDA in THF at –78 °C, and the mixture was stirred at –78 °C for 10 min. A solution of 2a (543 mg, 2.40 mmol) in THF (4.0 mL) was added to the mixture at –78 °C, and the mixture was stirred at –78 °C for 10 min. An aqueous NaOH solution (approximately 10%, 5.0 mL) was added to the mixture at –78 °C, and the mixture was stirred at room temperature for 30 min. The reaction was quenched with sat. aq NH4Cl (5.0 mL), and the mixture was extracted with CHCl3 (50 mL × 3) The combined organic layer was dried over MgSO4 and concentrated under reduced pressure. The residue was purified by column chromatography on silica gel (hexane-EtOAc, 3:1) to afford (E)-3a (Rf = 0.15, 387 mg, 1.16 mmol, 48.3%) and (Z)-3a (Rf = 0.10, 403 mg, 1.20 mmol, 50.4%). (E)-3a: Colorless crystals; mp 83.5–84.5 ℃ (hexane/EtOAc); IR (KBr): 3382 (OH), 3021, 2956, 2934, 2867, 1604, 1495, 1454, 1088, 1045, 1014, 958, 808, 697 cm–1; 1H NMR (300 MHz, CDCl3): δ = 2.42 (s, 3H), 2.48–2.53 (br m, 1H), 2.74–2.89 (m, 4H), 4.43 (dd, J = 7.6, 12.8 Hz, 1H), 4.68 (dd, J = 4.7, 12.8 Hz, 1H), 7.14–7.32 (m, 7H), 7.48 (d, J = 8.2 Hz, 2H); 13C NMR (126 MHz, CDCl3): δ = 21.4 (CH3), 32.8 (CH2), 34.5 (CH2), 61.2 (CH2), 124.9 (CH), 126.3 (CH), 128.4 (CH), 128.5 (CH), 129.8 (CH), 137.0 (C), 137.7 (C), 140.4 (C), 141.8 (C), 149.2 (C); MS (FAB+): m/z (%) = 335 ([M+H]+, 100), 317 (58), 91 (26); HRMS (FAB+): m/z [(M+H)+] calcd for C18H20ClO2S: 335.0873; found: 335.0873. (Z)-3a: Colorless crystals; mp 91.5–93.0 ℃ (hexane/EtOAc); IR (KBr): 3382 (OH), 3021, 2928, 1493, 1429, 1359, 1083, 1047, 1011, 811, 746, 700 cm–1; 1H NMR (300 MHz, CDCl3): δ = 1.88–1.98 (br m, 1H), 2.41 (s, 3H), 2.90–3.07 (m, 2H), 3.12–3.28 (m, 2H), 4.42 (dd, J = 6.5, 14.6 Hz, 1H), 4.48 (dd, J = 6.2, 14.6 Hz, 1H), 7.25–7.38 (m, 9H); 13C NMR (126 MHz, CDCl3): δ = 21.4 (CH3), 32.7 (CH2), 35.0 (CH2), 62.4 (CH2), 124.7 (CH), 126.5 (CH), 128.6 (CH), 128.7 (CH), 129.8 (CH), 133.8 (C), 137.5 (C), 140.3 (C), 141.8 (C), 150.1 (C); MS (FAB+): m/z (%) = 335 ([M+H]+, 100), 91 (18); HRMS (FAB+): m/z [(M+H)+] calcd for C18H20ClO2S: 335.0873; found: 335.0871.
17. A. D. Westwell, M. Thornton-Pett, and C. M. Rayner, J. Chem. Soc., Perkin Trans. 1, 1995, 847; CrossRef A. Arnone, P. Bravo, M. Frigerio, G. Salani, F. Viani, C. Zappalà, G. Cavicchio, and M. Crucianelli, Tetrahedron, 1995, 51, 8289; CrossRef R. Fernández de La Pradilla, M. V. Buergo, P. Manzano, C. Montero, J. Priego, A. Viso, F. H. Cano, and M. P. Martínez-Alcázar, J. Org. Chem., 2003, 68, 4797. CrossRef
18. Typical procedure: Phenyl chloroformate (1.96 g, 12.5 mmol) was added to a solution of pyridine (989 mg, 12.5 mmol) and 3a (837 mg, 2.50 mmol) in CHCl3 (25 mL) at 0 °C, and the mixture was stirred at 0 °C for 10 min. The reaction was quenched with sat. aq NH4Cl (4.0 mL), and the mixture was extracted with CHCl3 (20 mL × 3). The combined organic layer was dried over MgSO4 and concentrated under reduced pressure. The residue was purified by column chromatography on silica gel [Rf = 0.47 (hexane-EtOAc, 3:1)] to afford 4a (1.13 g, 2.48 mmol, 99%). (E)-4a: Colorless oil; IR (neat): 3062, 3028, 2926, 2867, 1770 (C=O), 1593, 1495, 1456, 1374, 1242, 1163, 1089, 1062, 1022, 811, 753 cm–1; 1H NMR (400 MHz, CDCl3): δ = 2.42 (s, 3H), 2.73–2.91 (m, 4H), 5.18 (d, J = 12.6 Hz, 1H), 5.26 (d, J = 12.6 Hz, 1H), 7.16–7.23 (m, 5H), 7.25–7.33 (m, 5H), 7.39–7.44 (m, 2H), 7.51–7.54 (m, 2H); 13C NMR (126 MHz, CDCl3): δ = 21.4 (CH3), 32.7 (CH2), 34.5 (CH2), 65.6 (CH2), 120.8 (CH), 124.8 (CH), 126.3 (CH), 126.5 (CH), 128.3 (CH), 128.6 (CH), 129.6 (CH), 129.9 (CH), 137.5 (C), 139.9 (C), 142.0 (C), 142.2 (C), 142.3 (C), 150.9 (C), 153.2 (C); MS (FAB+): m/z (%) = 455 ([M+H]+, 29), 317 (100), 177 (18), 141 (19), 91 (32); HRMS (FAB+): m/z [(M+H)+] calcd for C25H24ClO4S: 455.1084; found: 455.1088. (Z)-4a: Colorless crystals; mp 99.0–100.0 ℃ (hexane/EtOAc); IR (KBr): 3061, 3030, 2962, 2934, 1762 (C=O), 1592, 1495, 1457, 1425, 1369, 1286, 1251, 1210, 1086, 1054, 1018, 947, 802, 748, 699 cm–1; 1H NMR (400 MHz, CDCl3): δ = 2.41 (s, 3H), 2.90–3.04 (m, 2H), 3.11–3.23 (m, 2H), 5.02 (d, J = 14.0 Hz, 1H), 5.08 (d, J = 14.0 Hz, 1H), 7.16–7.19 (m, 2H), 7.25–7.29 (m, 6H), 7.33–7.42 (m, 6H); 13C NMR (126 MHz, CDCl3): δ = 21.4 (CH3), 32.9 (CH2), 35.0 (CH2), 66.9 (CH2), 120.8 (CH), 124.7 (CH), 126.3 (CH), 126.7 (CH), 128.5 (CH), 128.8 (CH), 129.6 (CH), 129.9 (CH), 137.5 (C), 138.2 (C), 139.7 (C), 142.0 (C), 142.6 (C), 150.9 (C), 153.2 (C); MS (FAB+): m/z (%) = 455 ([M+H]+, 100), 317 (48), 154 (46), 136 (43), 93 (55); HRMS (FAB+): m/z [(M+H)+] calcd for C25H24ClO4S: 455.1084; found: 455.1087.
19. P. Knochel, W. Dohle, N. Gommermann, F. F. Kneisel, F. Kopp, T. Korn, I. Sapountzis, and V. A. Vu, Angew. Chem. Int. Ed., 2003, 42, 4302. CrossRef
20. A. Krasovskiy and P. Knochel, Angew. Chem. Int. Ed., 2004, 43, 3333. CrossRef
21. Typical procedure: A solution of 4a (45.5 mg; 0.100 mmol) in THF (0.6 mL) was added to a solution of i-PrMgCl•LiCl (0.40 mmol) in THF (4.4 mL) at 0 °C. The reaction mixture was allowed to warm to room temperature and stirred at room temperature for 30 min. The reaction was quenched with sat. aq NH4Cl (2.0 mL), and the mixture was extracted with CHCl3 (15 mL × 3). The combined organic layer was dried over MgSO4 and concentrated under reduced pressure. The residue was purified by column chromatography on silica gel [Rf = 0.19 (hexane-EtOAc, 3:1)] to afford 5a (22.2 mg, 0.076 mmol, 76%) as colorless crystals. Mp 71.0–72.0 ℃ (hexane/EtOAc); IR (KBr): 2932, 1756 (C=O), 1651, 1602, 1496, 1455, 1353, 1136, 1037, 996, 758, 708 cm–1; 1H NMR (300 MHz, CDCl3): δ = 2.81–2.95 (m, 4H), 4.55 (s, 2H), 7.16–7.19 (m, 2H), 7.22–7.35 (m, 3H); 13C NMR (126 MHz, CDCl3): δ = 28.7, 32.6, 71.3, 119.8, 126.9, 128.1, 128.9, 139.2, 159.0, 168.5; MS (EI): m/z (%) = 222 (M+, 6), 187 (7), 91 (100); HRMS (EI): m/z [M+] calcd for C12H11ClO2: 222.0448; found: 222.0446.