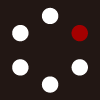
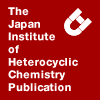
HETEROCYCLES
An International Journal for Reviews and Communications in Heterocyclic ChemistryWeb Edition ISSN: 1881-0942
Published online by The Japan Institute of Heterocyclic Chemistry
e-Journal
Full Text HTML
Received, 30th June, 2014, Accepted, 18th July, 2014, Published online, 24th July, 2014.
DOI: 10.3987/COM-14-S(K)66
■ Studies toward the Total Synthesis of Amphidinolide N: Stereocontrolled Synthesis of the C13–C29 Segment
Makoto Sasaki,* Yuki Kawashima, and Haruhiko Fuwa
Laboratory of Biostructural Chemistry, Graduate School of Life Sciences, Tohoku University, 2-1-1, Katahira, Aoba-ku, Sendai, 980-8577, Japan
Abstract
A stereocontrolled synthesis of the C13–C29 segment of amphidinolide N, a marine macrolide natural product that is extremely potent cytotoxic, is described.INTRODUCTION
Amphidinolides are a large family of cytotoxic macrolide natural products that are produced by the symbiotic marine dinoflagellate, Amphidinium sp., which has been isolated from the Okinawan flatworm, Amphiscolops sp. More than 30 macrolides with diverse structures have so far been isolated from a variety of strains of Amphidinium sp. by Kobayashi and co-workers.1 Amphidinolide N (1, Figure 1), which was isolated from the cultured Amphidinium sp. (Y-5 strain) by the Kobayashi group in 1994, is the most potent cytotoxic member of the amphidinolide family discovered so far and its IC50 values against murine lymphoma L1210 and human epidermoid carcinoma KB cells are 0.05 and 0.06 ng/mL, respectively.2 The gross structure of 1, including its partial stereochemical assignment, was proposed on the basis of 2D NMR spectroscopic studies. The structure has recently been revised, and the relative configuration has been reported.3 Amphidinolide N consists of a 26-membered macrolide skeleton containing a six-membered hemiacetal, 2,5-trans-disubstituted tetrahydrofuran, allylic epoxide, and 13 stereogenic centers. Soon after amphidinolide N had been isolated, Shimizu and co-workers isolated a closely related macrolide, caribenolide I (2, Figure 1), from the cultured free-swimming Caribbean dinoflagellate, Amphidinium sp. S1-36-5.4 Compound 2 has the same gross structure as 1, and it was found to exhibit in vivo antitumor activity.
The potent cytotoxicity of amphidinolides and their diverse and complex molecular architectures make them important targets for total synthesis. Total syntheses of this family of macrolides have been documented by many research groups,5 but total synthesis of amphidinolide N has not yet been reported in the literature.6,7 Here, we describe a stereocontrolled synthesis of the C13–C29 segment 3 (Figure 1) of amphidinolide N.
RESULTS AND DISCUSSION
Our retrosynthetic plan for the C13–C29 segment 3 is outlined in Scheme 1. We envisaged that 3 would be obtained from two fragments, 4 and 5, via a Horner–Wadsworth–Emmons (HWE) reaction followed by a 1,4-reduction and chelation-controlled reduction of the product. We expected the 2,5-trans-disubstituted tetrahydrofuran of 5 to be constructed via an intramolecular cyclization of diol mesylate 6, which in turn would be derived through a chemoselective asymmetric dihydroxylation of the precursor diene 7.
The synthesis of β-keto phosphonate 4 started with (S)-4-benzyl-3-((2-benzyloxy)acetyl)oxazolidin-2-one (8)8 (Scheme 2). Evans aldol reaction9 of the boron enolate derived from 8 with acrolein afforded alcohol 9 in 90% yield as a single stereoisomer. The chiral auxiliary was removed by converting 9 into the corresponding Weinreb amide,10 and the secondary hydroxy group was protected as its triethylsilyl (TES) ether to give 10 in 79% yield for the two steps. Treating amide 10 with lithiated dimethyl methylphosphonate afforded β-keto phosphonate 4 in 94% yield. The absolute configurations at the C1411 and C15 positions, which were generated in the Evans aldol reaction, were unambiguously established by converting alcohol 9 into the known triol 1212 in a three-step sequence including TES-protection, ozonolysis followed by reduction using NaBH4, and deprotection of the TES ether.
The synthesis of aldehyde 5 commenced with the known aldehyde 13.13 Keck asymmetric allylation14 of 13 afforded homoallylic alcohol 7 in 87% yield (Scheme 3). The absolute configuration of the C21 stereogenic center was established by a modified Mosher analysis,15 as shown in Figure 2. The enantiomer ratio (er) of alcohol 7 was found to be 99.2:0.8 from HPLC analysis of the corresponding p-methoxybenzoate derivative. Alcohol 7 was then converted into the corresponding mesylate, which was subjected to Sharpless asymmetric dihydroxylation (SAD) using AD-mix-β.16 Chemoselective dihydroxylation of the disubstituted internal olefin proceeded with concomitant partial cyclization17 to give a mixture of the corresponding diol 6 and tetrahydrofuran 14. The obtained unpurified mixture was treated with 1,8-diazabicyclo[5.4.0]undec-7-ene (DBU) in toluene at 100 °C to complete the cyclization, and the desired 1418 was produced in 73% yield over the three steps as a single stereoisomer. The absolute configuration of the C25 stereogenic center, which was generated in the SAD, was unambiguously established using a modified Mosher analysis (Figure 3A).15 The 2,5-trans configuration of the tetrahydrofuran ring of 14 was surmised by the presumed reaction mechanism and established by NOE experiments (Figure 3B).19 Furthermore, the relative configuration of 14 was unambiguously confirmed by X-ray crystallographic analysis of its triol derivative 1520 as shown in Figure 4.21
Alcohol 14 was protected as its p-methoxybenzyl (MPM) ether, then olefin cross-metathesis22 of the resultant 1618 with methyl acrylate using the Grubbs second-generation catalyst (G-II)23 afforded α,β-unsaturated ester 17 in 83% yield with an E/Z ratio of greater than 20:1 (Scheme 3). Subsequent diisobutylaluminum hydride (DIBALH) reduction of 17, followed by Sharpless asymmetric epoxidation24 of the resultant allylic alcohol 18 using (+)-diethyl tartrate (DET) as a chiral ligand, produced epoxy alcohol 19 in high overall yield and as a single stereoisomer. Iodination of the primary alcohol of 19 followed by reduction of the product with zinc afforded secondary allylic alcohol 20 in 95% yield for the two steps. The absolute configuration at C19 in 20 was established using a modified Mosher analysis,15 as shown in Figure 5. Alcohol 20 was protected as its MPM ether (93%), and oxidative cleavage of the terminal double bond of the resulting 21 (OsO4, N-methylmorpholine N-oxide (NMO), then NaIO4) gave aldehyde 5 in 90% yield.
With the requisite fragments in hand, we proceeded to their coupling reaction. HWE reaction of 4 and 5 under Masamune–Roush conditions (LiCl, i-Pr2NEt, MeCN)25 proceeded smoothly to exclusively give (E)-enone 22 in 94% yield (Scheme 4). 1,4-Reduction of 22 using the Stryker reagent26 gave ketone 23 (95%), which was subjected to chelation-controlled reduction using Zn(BH4)227,28 to give alcohol 24 in 80% yield as a single stereoisomer. Finally, acidic cleavage of the TES ether and protection of the resultant diol 25 as its tert-butyldimethylsilyl (TBS) ether afforded bis-TBS ether 3. The absolute configuration of the newly generated stereogenic center at C16 was established using NOE experiments on the acetonide derivative 26 and from the 13C NMR chemical shifts29,30 for 26, as shown in Figure 6.
In conclusion, we have achieved a stereocontrolled synthesis of the C13–C29 segment 3 of amphidinolide N, an exceedingly cytotoxic marine macrolide. The synthesis involves the efficient construction of a 2,5-trans-disubstituted tetrahydrofuran via a chemoselective Sharpless asymmetric dihydroxylation–intramolecular cyclization sequence, Horner–Wadsworth–Emmons reaction of the fragments, and chelation-controlled reduction using Zn(BH4)2 to set the C16 stereogenic center. Further studies aimed at achieving the total synthesis of amphidinolide N are currently being conducted and will be reported in due course.
EXPERIMENTAL
General remarks. All reactions sensitive to moisture and/or air were carried out under an atmosphere of argon in dry, freshly distilled solvents under anhydrous conditions using oven-dried glassware unless otherwise noted. Anhydrous tetrahydrofuran (THF), diethyl ether (Et2O), and toluene were purified by a Glass Contour solvent purification system under an atmosphere of argon immediately prior to use. Anhydrous dichloromethane (CH2Cl2) was purchased from Kanto Chemical Co., Inc. and used as received. N,N-Dimethylformamide (DMF) was distilled from magnesium sulfate under reduced pressure. Acetonitrile (MeCN), diisopropylethylamine (i-Pr2NEt), 2,6-lutidine, and triethylamine (Et3N) were distilled from calcium hydride under an atmosphere of argon. All other chemicals were purchased at highest commercial grade and used as received. Analytical thin-layer chromatography was performed using E. Merck silica gel 60 F254 plates (0.25-mm thickness). Flash column chromatography was carried out using Kanto Chemical silica gel 60N (40–100 mesh, spherical, neutral) or Fuji Silysia silica gel BW-300 (200–400 mesh). Melting points were measured on a Yanagimoto melting points apparatus and are uncorrected. Optical rotations were recorded on a JASCO P-1020 digital polarimeter. IR spectra were recorded on a JASCO FT/IR-4100 spectrometer. 1H and 13C NMR spectra were recorded on a JEOL JNM ECA-600 spectrometer. Chemical shift values were reported in δ (ppm) downfield from tetramethylsilane with reference to internal residual solvent [1H NMR, CHCl3 (7.24), C6HD5 (7.15); 13C NMR, CDCl3 (77.0), C6D6 (128.0)]. Coupling constants (J) were reported in hertz (Hz). The following abbreviations were used to designate the multiplicities: s = singlet; d = doublet; t = triplet; m = multiplet; q = quartet; br = broad. ESI-TOF mass spectra were measured on a Bruker microTOFfocus spectrometer. Diastereomer ratio (dr) was estimated by 1H NMR spectroscopic analysis (600 MHz), unless otherwise noted.
Alcohol 9. To a solution of (S)-4-benzyl-3-((2-benzyloxy)acetyl)oxazolidin-2-one (8) (100.3 mg, 308.3 µmol) in CH2Cl2 (3.0 mL) at 0 ºC were added n-Bu2BOTf (90 µL, 0.36 mmol) and Et3N (60 µL, 0.43 mmol), and the resultant solution was stirred at 0 ºC for 1 h. To this mixture at –78 ºC was added a solution of acrolein (40 µL, 0.60 mmol). The resultant solution was stirred at –78 ºC for 1 h and allowed to warm to 0 ºC over a period of 1 h. To the solution were added a mixture of MeOH/pH 7 phosphate buffer (7:3, v/v, 3 mL) and a mixture of MeOH/30% aqueous H2O2 solution (2:1, v/v, 2 mL), and the resultant mixture was stirred at 0 °C for 1 h. The resultant mixture was extracted with EtOAc, and the organic layer was washed with saturated aqueous NaHCO3 solution and brine, dried over Na2SO4, filtered, and concentrated under reduced pressure. Purification of the residue by column chromatography (silica gel, 25 to 40% EtOAc/hexanes) gave alcohol 9 (105.1 mg, 90%) as a colorless oil: [α]D24 +23.5 (c 1.00, CHCl3); IR (film) 3480, 3062, 3030, 2924, 2869, 1777, 1705, 1391, 1212, 1112, 700 cm–1; 1H NMR (600 MHz, C6D6) δ 7.38–7.36 (m, 2H), 7.16–7.13 (m, 2H), 7.08–6.98 (m, 4H), 6.84–6.82 (m, 2H), 6.12 (ddd, J = 17.0, 10.6, 5.0 Hz, 1H), 5.47 (d, J = 3.2 Hz, 1H), 5.42 (ddd, J = 17.0, 1.4, 1.4 Hz, 1H), 5.10 (ddd, J = 10.6, 1.3, 1.3 Hz, 1H), 4.71 (m, 1H), 4.66 (d, J = 11.5 Hz, 1H), 4.37 (d, J = 11.5 Hz, 1H), 4.11–4.08 (m, 1H), 3.39 (dd, J = 8.3, 1.9 Hz, 1H), 3.09 (dd, J = 8.3, 8.3 Hz, 1H), 2.92 (dd, J = 13.8, 3.2 Hz, 1H), 2.70 (br s, 1H), 2.28 (dd, J = 13.8, 9.2 Hz, 1H); 13C NMR (150 MHz, CDCl3) δ 170.2, 153.4, 136.9, 136.4, 135.0, 129.4 (2C), 129.0 (2C), 128.5 (2C), 128.4 (2C), 128.2, 127.5, 117.1, 79.6, 73.6, 73.3, 67.0, 55.5, 37.7; HRMS (ESI) calcd for C22H23NO5Na [(M + Na)+] 404.1468, found 404.1487.
TES ether 10. To a solution of MeNH(OMe)·HCl (108.9 mg, 1.116 mmol) in THF (1.5 mL) at 0 ºC was added Me3Al (1.09 M solution in n-hexane, 1.0 mL, 1.1 mmol), and the resultant solution was stirred at room temperature for 35 min. To this mixture at 0 ºC was added a solution of alcohol 9 (136.7 mg, 358.4 µmol) in THF (0.5 mL + 0.5 mL rinse), and the resultant solution was stirred at room temperature for 3.3 h. The mixture was diluted with saturated aqueous potassium sodium tartrate solution, and the resultant biphasic mixture was vigorously stirred at room temperature until the layers became clear. The resultant mixture was diluted with EtOAc, washed with H2O and brine, dried over Na2SO4, filtered, and concentrated under reduced pressure to give crude Weinreb amide (168.2 mg), which was contaminated with some impurities and used in the next reaction without further purification.
To a solution of the above Weinreb amide (168.2 mg) in CH2Cl2 (3 mL) at 0 ºC were added 2,6-lutidine (200 µL, 1.73 mmol) and TESOTf (240 µL, 1.06 mmol), and the resultant solution was stirred at 0 ºC for 1 h. The reaction mixture was diluted with saturated aqueous NaHCO3 solution and EtOAc. The organic layer was washed with H2O and brine, dried over Na2SO4, filtered, and concentrated under reduced pressure. Purification of the residue by column chromatography (silica gel, 5 to 10% EtOAc/hexanes) gave TES ether 10 (107.8 mg, 79% for the two steps) as a pale yellow oil: [α]D24 –9.4 (c 1.00, CHCl3); IR (film) 2954, 2911, 2876, 1671, 1457, 1089, 1005, 739 cm–1; 1H NMR (600 MHz, CDCl3) δ 7.41–7.22 (m, 5H), 5.81 (ddd, J = 17.0, 10.6, 6.8 Hz, 1H), 5.23 (d, J = 17.0 Hz, 1H), 5.09 (d, J = 10.5 Hz, 1H), 4.67 (d, J = 12.4 Hz, 1H), 4.55 (d, J = 12.4 Hz, 1H), 4.46 (dd, J = 6.8, 6.8 Hz, 1H), 4.30 (d, J = 6.8 Hz, 1H), 3.43 (s, 3H), 3.10 (s, 3H), 0.92 (t, J = 7.8 Hz, 9H), 0.60 (q, J = 7.8 Hz, 6H); 13C NMR (150 MHz, CDCl3) δ 171.3, 138.0, 137.0, 128.2 (2C), 127.9 (2C), 127.5, 116.7, 79.4, 75.6, 72.4, 61.2, 32.3, 6.8 (3C), 4.9 (3C); HRMS (ESI) calcd for C20H33NO4SiNa [(M + Na)+] 402.2071, found 402.2080.
β-Keto phosphonate 4. To a solution of dimethyl methylphosphonate (50 µL, 0.47 mmol) in THF (1.0 mL) at –78 ºC was added n-BuLi (1.60 M solution in n-hexane, 0.40 mL, 0.64 mmol), and the resultant solution was stirred at –78 ºC for 45 min. To this solution at –78 ºC was added a solution of TES ether 10 (60.1 mg, 0.158 mmol) in THF (0.2 mL + 0.2 mL rinse), and the resultant solution was stirred at –78 °C for 1 h. The reaction was quenched with saturated aqueous NH4Cl solution. The resultant mixture was diluted with EtOAc, washed with H2O and brine, dried over Na2SO4, filtered, and concentrated under reduced pressure. Purification of the residue by column chromatography (silica gel, 40% EtOAc/hexanes) gave β-keto phosphonate 4 (65.8 mg, 94%) as a colorless oil: [α]D24 +28.9 (c 1.00, CHCl3); IR (film) 2955, 2877, 1722, 1258, 1032, 742 cm–1; 1H NMR (600 MHz, CDCl3) δ 7.36–7.26 (m, 5H), 5.86 (ddd, J = 17.0, 10.5, 4.1 Hz, 1H), 5.25 (ddd, J = 17.0, 1.4, 1.4 Hz, 1H), 5.13 (ddd, J = 10.5, 1.4, 1.4 Hz, 1H), 4.74 (d, J = 12.0 Hz, 1H), 4.43 (d, J = 12.0 Hz, 1H), 4.40 (dddd, J = 4.1, 4.1, 1.4, 1.4 Hz, 1H), 4.12 (d, J = 4.1 Hz, 1H), 3.75 (d, J = 11.0 Hz, 3H), 3.71 (d, J = 11.0 Hz, 3H), 3.34 (dd, J = 22.4, 14.7 Hz, 1H), 3.23 (dd, J = 22.4, 14.7 Hz, 1H), 0.89 (t, J = 7.8 Hz, 9H), 0.54 (q, J = 7.8 Hz, 6H); 13C NMR (150 MHz, CDCl3) δ 201.9 (d, J = 7.2 Hz), 137.5, 136.4, 128.4 (2C), 128.2 (2C), 128.0, 116.4, 87.1, 74.4, 73.0, 53.0 (d, J = 5.7 Hz), 52.9 (d, J = 5.7 Hz), 39.0 (d, J = 129.0 Hz), 6.8 (3C), 4.8 (3C); HRMS (ESI) calcd for C21H35O6PSiNa [(M + Na)+] 465.1833, found 465.1847.
TES ether 11. To a solution of alcohol 9 (233.0 mg, 0.6109 mmol) in CH2Cl2 (6 mL) at 0 ºC were added 2,6-lutidine (0.14 mL, 1.2 mmol) and TESOTf (0.17 mL, 0.75 mmol), and the resultant solution was stirred at 0 ºC for 2 h 10 min. The reaction was quenched with saturated aqueous NaHCO3 solution. The resultant mixture was extracted with EtOAc, and the organic layer was washed with saturated aqueous CuSO4 solution and brine, dried over Na2SO4, filtered, and concentrated under reduced pressure. Purification of the residue by column chromatography (silica gel, 2 to 40% EtOAc/hexanes) gave TES ether 11 (22.7 mg, 73%) as a colorless oil: [α]D24 +58.7 (c 1.00, CHCl3); IR (film) 2954, 2912, 2876, 1783, 1706, 1210, 1108, 735 cm–1; 1H NMR (600 MHz, CDCl3) δ 7.39–7.37 (m, 2H), 7.33–7.23 (m, 6H), 7.17–7.16 (m, 2H), 5.94 (ddd, J = 17.0, 10.1, 6.0 Hz, 1H), 5.42 (d, J = 6.0 Hz, 1H), 5.21 (ddd, J = 17.0, 1.4, 1.4 Hz, 1H), 5.14 (ddd, J = 10.1, 1.4, 1.4 Hz, 1H), 4.68–4.64 (m, 2H), 4.49 (m, 1H), 4.45 (dd, J = 6.0, 6.0 Hz, 1H), 4.10 (dd, J = 8.7, 2.3 Hz, 1H), 4.06 (dd, J = 8.7, 8.7 Hz, 1H), 3.13 (dd, J = 13.8, 3.2 Hz, 1H), 2.57 (dd, J = 13.8, 9.7 Hz, 1H), 0.89 (t, J = 7.8 Hz, 9H), 0.56 (q, J = 7.8 Hz, 6H); 13C NMR (150 MHz, CDCl3) δ 170.6, 153.1, 137.6, 136.8, 135.3, 129.4 (2C), 128.9 (2C), 128.4 (2C), 128.3 (2C), 127.9, 127.3, 116.7, 79.7, 74.9, 73.5, 66.4, 55.7, 37.8, 6.7 (3C), 4.7 (3C); HRMS (ESI) calcd for C28H37NO5SiNa [(M + Na)+] 518.2333, found 518.2326.
Triol 12. Ozone was bubbled through a solution of TES ether 11 (51.2 mg, 0.103 mmol) in CH2Cl2/MeOH (1:1, v/v, 2 mL) at –78 °C until a pale blue color persisted (ca. 25 min). Oxygen was bubbled through the reaction mixture to remove excess ozone, and then NaBH4 (25.3 mg, 0.669 mmol) was added at –78 °C. The resultant mixture was allowed to warm to room temperature over 8 h 20 min. The reaction was quenched with saturated aqueous NH4Cl solution. The resultant mixture was diluted with EtOAc, washed with H2O and brine, dried over Na2SO4, filtered, and concentrated under reduced pressure. The residue was roughly purified by column chromatography (silica gel, 30% EtOAc/hexanes) to give crude diol (17.3 mg), which was used in the next reaction without further purification.
To a solution of the above diol (17.3 mg) in MeOH/CH2Cl2 (1:1, v/v, 0.6 mL) was added PPTS (2.1 mg, 8.4 µmol), and the resultant solution was stirred at room temperature for 1 h 35 min. The reaction mixture was neutralized with Et3N and concentrated under reduce pressure. Purification of the residue by column chromatography (silica gel, 50% EtOAc/hexanes to EtOAc) gave triol 12 (10.3 mg, 47% for the two steps) as a white solid, which was recrystallized from CHCl3/hexanes: mp 73.8–76.1 ºC [lit.12 mp 75.3–76.6 °C]; [α]D24 –15.6 (c 0.50, MeOH) [lit.12 [α]D25 –16.5 (c 1.08, EtOH)]; 1H NMR (600 MHz, CDCl3) δ 7.39–7.18 (m, 5H), 4.71 (d, J = 11.5 Hz, 1H), 4.58 (d, J = 11.5 Hz, 1H), 3.88 (dd, J = 11.8, 4.6 Hz, 1H), 3.83 (dd, J = 9.2, 4.6 Hz, 1H), 3.76–3.72 (m, 2H), 3.68 (dd, J = 11.8, 4.1 Hz, 1H), 3.57 (dd, J = 9.2, 3.9 Hz, 1H), (three protons missing due to H/D exchange); 13C NMR (150 MHz, CDCl3) δ 137.6, 128.7 (2C), 128.2, 128.0 (2C), 79.2, 72.5, 71.6, 63.0, 61.0. The spectroscopic data of triol 12 were consistent with those reported in the literature.12
Homoallylic alcohol 7. To a mixture of (R)-BINOL (407.3 mg, 1.422 mmol), freshly activated 4 Å molecular sieves (2.94 g) in CH2Cl2 (16 mL) was added Ti(Oi-Pr)4 (400 µL, 1.35 mmol), and the resultant mixture was heated under reflux for 1 h. To the reaction mixture at room temperature was added aldehyde 13 (1.91 g, 13.7 mmol) in CH2Cl2 (2 mL + 2 × 1 mL rinse), and the resultant mixture was stirred for 10 min. The reaction mixture was cooled to –78 °C and treated with allyltributylstannane (6.40 mL, 20.6 mmol). The resultant mixture was stirred at –20 ºC for 3 days. The reaction was quenched with saturated aqueous NaHCO3 solution at –78 °C. The resultant mixture was diluted with CH2Cl2 (15 mL), allowed to warm to room temperature over 1.5 h, and filtered through a pad of Celite. The filtrate was extracted with Et2O, and the organic layer was washed with brine, dried over MgSO4, filtered, and concentrated under reduced pressure. Purification of the residue by column chromatography (silica gel, 2 to 10% EtOAc/hexanes) gave homoallylic alcohol 7, which was contaminated with the starting aldehyde 13. This material was further purified twice by column chromatography (silica gel, 10% EtOAc/hexanes to EtOAc) to give homoallylic alcohol 7 (2.17 g, 87%) as a pale yellow oil: [α]D26 –5.6 (c 1.00, CHCl3); IR (film) 3356, 2956, 2926, 2872, 2854, 2360, 1641, 1437, 993, 967, 913 cm–1; 1H NMR (600 MHz, CDCl3) δ 5.81 (m, 1H), 5.46–5.37 (m, 2H), 5.13 (m, 1H), 5.11 (m, 1H), 3.64 (m, 1H), 2.27 (m, 1H), 2.16–2.04 (m, 3H), 1.96 (ddd, J = 6.6, 6.6, 6.6 Hz, 2H), 1.52–1.49 (m, 2H), 1.32–1.25 (m, 4H), 0.86 (t, J = 7.4 Hz, 3H), (one proton missing due to H/D exchange); 13C NMR (150 MHz, CDCl3) δ 134.5, 130.8, 129.2, 117.7, 70.0, 41.6, 36.2, 31.9, 31.4, 28.6, 21.9, 13.6.
p-Methoxybenzoylation of alcohol 7. To a solution of homoallylic alcohol 7 (12.7 mg, 69.7 µmol) in pyridine (0.5 mL) were added DMAP (11.9 mg, 97.4 µmol) and p-methoxybenzoyl chloride (71.6 mg, 420 µmol). The resultant solution was stirred at room temperature for 15 h. The reaction was quenched with H2O. The resultant mixture was extracted with EtOAc, and the organic layer was washed four times with 1 M aqueous HCl solution, saturated aqueous NaHCO3 solution, and brine. The organic layer was dried over Na2SO4, filtered, and concentrated under reduced pressure. Purification of the residue by column chromatography (silica gel, 2% EtOAc/hexanes) gave the corresponding p-methoxybenzoate (18.5 mg, 84%) as a colorless oil: [α]D24 –27.1 (c 1.00, CHCl3); IR (film) 2956, 2928, 2853, 1711, 1606, 1510, 1274, 1256, 1168, 1101, 1033, 769 cm–1; 1H NMR (600 MHz, CDCl3) δ 7.99–7.96 (m, 2H), 6.91–6.88 (m, 2H), 5.82 (dddd, J = 17.0, 10.1, 6.9, 6.9 Hz, 1H), 5.41–5.34 (m, 2H), 5.12 (dddd, J = 11.0, 11.0, 5.9, 5.9 Hz, 1H), 5.08 (ddd, J = 17.0, 3.2, 1.0 Hz, 1H), 5.03 (ddd, J = 10.1, 1.0, 1.0 Hz, 1H), 3.84 (s, 3H), 2.41 (dd, J = 7.3, 7.3 Hz, 2H), 2.10–2.01 (m, 2H), 1.94 (ddd, J = 6.4, 6.4, 6.4 Hz, 2H), 1.78–1.65 (m, 2H), 1.30–1.25 (m, 4H), 0.85 (t, J = 7.3 Hz, 3H); 13C NMR (150 MHz, CDCl3) δ 165.9, 163.2, 133.7, 131.5 (2C), 131.2, 128.9, 123.1, 117.7, 113.5 (2C), 73.1, 55.4, 38.7, 33.6, 32.2, 31.6, 28.5, 22.2, 13.9; HRMS (ESI) calcd for C20H28O3Na [(M + Na)+] 339.1931, found 339.1926. The enantiomer ratio of this benzoate was determined to be 99.2:0.8 by chiral HPLC analysis in comparison with authentic racemic material (CHIRALCEL OJ-H, 4.6 mm I.D. × 250 mm, flow rate: 1.0 mL/min, UV detection: 254 nm, eluent: hexanes, major peak: t = 16.8 min; minor peak: t = 14.1 min).
Tetrahydrofuran 14. To a solution of homoallylic alcohol 7 (2.12 g, 11.6 mmol) in CH2Cl2 (100 mL) at 0 ºC were added Et3N (2.10 mL, 15.1 mmol) and MsCl (1.00 mL, 12.9 mmol). The resultant solution was stirred at 0 ºC for 30 min. The reaction mixture was diluted with EtOAc and washed successively with 1 M aqueous HCl solution, saturated aqueous NaHCO3 solution, and brine. The organic layer was dried over Na2SO4, filtered, and concentrated under reduced pressure to give crude mesylate (3.23 g), which was used in the next reaction without further purification.
To a solution of the above mesylate (3.23 g) in t-BuOH/H2O (1:1, v/v, 100 mL) at 0 ºC were added MeSO2NH2 (1.11 g, 11.7 mmol) and AD-mix-β (16.3 g). The resultant mixture was vigorously stirred at 0 ºC for 5 h 20 min. The reaction was quenched with solid Na2SO3. The resultant mixture was stirred at room temperature for 1 h and then extracted with EtOAc. The organic layer was washed with 3 M aqueous NaOH solution and brine, dried over Na2SO4, filtered, and concentrated under reduced pressure to give crude product (3.56 g), which was used in the next reaction without further purification.
To a solution of the above material (3.56 g) in toluene (50 mL) was added DBU (2.00 mL, 13.4 mmol), and the resultant solution was stirred at 100 ºC for 1.5 h. The reaction mixture was cooled to room temperature and concentrated under reduced pressure. Purification of the residue by column chromatography (silica gel, 0 to 1% EtOAc/benzene) gave tetrahydrofuran 14 (1.68 g, 73% for the three steps, dr >20:1 estimated by 600 MHz 1H NMR spectroscopy) as a pale yellow oil: [α]D26 +13.8 (c 1.00, CHCl3); IR (film) 3464, 3077, 2957, 2931, 2871, 2861, 2360, 2340, 1642, 1065, 914 cm–1; 1H NMR (600 MHz, CDCl3) δ 5.78 (dddd, J = 17.0, 10.1, 6.9, 6.9 Hz, 1H), 5.07 (ddd, J = 17.0, 3.2, 1.4 Hz, 1H), 5.03 (dddd, J = 10.1, 3.2, 1.4, 1.4 Hz, 1H), 3.95 (dddd, J = 7.8, 6.9, 6.9, 6.9 Hz, 1H), 3.79 (ddd, J = 6.9, 6.9, 6.9 Hz, 1H), 3.35 (ddd, J = 6.9, 6.9, 5.0 Hz, 1H), 2.35 (ddddd, J = 13.8, 6.9, 6.9, 1.4, 1.4 Hz, 1H), 2.21 (ddddd, J = 13.8, 6.9, 6.9, 1.4, 1.4 Hz, 1H), 2.02–1.93 (m, 2H), 1.63–1.54 (m, 2H), 1.47 (m, 1H), 1.41–1.28 (m, 5H), 0.88 (t, J = 7.3 Hz, 3H), (one proton missing due to H/D exchange); 13C NMR (150 MHz, CDCl3) δ 134.8, 116.9, 82.2, 78.5, 74.1, 39.9, 33.0, 31.8, 28.3, 27.8, 22.8, 14.0; HRMS (ESI) calcd for C12H22O2Na [(M + Na)+] 221.1512, found 221.1538.
MPM ether 16. To a solution of alcohol 14 (1.68 g, 8.47 mmol) in DMF (40 mL) at 0 ºC was added NaH (60% in mineral oil, 0.53 g, 13 mmol), and the resultant mixture was stirred at room temperature for 35 min. To the resultant mixture at 0 ºC were added n-Bu4NI (319.5 mg, 0.8650 mmol) and MPMCl (1.50 mL, 11.0 mmol), and the resultant mixture was stirred at 35 ºC for 17 h 20 min. The reaction was quenched with saturated aqueous NH4Cl solution at 0 °C. The resultant mixture was diluted with Et2O, washed with H2O and brine, dried over Na2SO4, filtered, and concentrated under reduce pressure. Purification of the residue by column chromatography (silica gel, 5% EtOAc/hexanes) gave MPM ether 16 (2.64 g, 98%) as a colorless oil: [α]D24 +21.9 (c 1.00, CHCl3); IR (film) 3074, 2955, 2932, 2861, 1613, 1514, 1248, 1088, 1038, 821 cm–1; 1H NMR (600 MHz, CDCl3) δ 7.28–7.26 (m, 2H), 6.86–6.82 (m, 2H), 5.81 (dddd, J = 17.0, 10.1, 6.9, 6.9 Hz, 1H), 5.07 (ddd, J = 17.0, 3.7, 1.8 Hz, 1H), 5.02 (ddd, J = 10.1, 3.7, 1.8 Hz, 1H), 4.64 (d, J = 11.0 Hz, 1H), 4.51 (d, J = 11.0 Hz, 1H), 4.04 (ddd, J = 8.2, 6.9, 6.9 Hz, 1H), 3.98 (dddd, J = 8.2, 6.9, 6.9, 6.9 Hz, 1H), 3.78 (s, 3H), 3.28 (m, 1H), 2.36 (ddd, J = 13.7, 6.9, 6.9 Hz, 1H), 2.21 (ddd, J = 13.7, 6.9, 6.9 Hz, 1H), 1.97 (m, 1H), 1.90 (m, 1H), 1.63 (m, 1H), 1.51 (m, 1H), 1.44–1.42 (m, 3H), 1.32–1.23 (m, 3H), 0.85 (t, J = 7.3 Hz, 3H); 13C NMR (150 MHz, CDCl3) δ 159.0, 135.2, 131.4, 129.5 (2C), 116.6, 113.6 (2C), 81.2, 81.1, 78.6, 72.4, 55.3, 40.2, 31.6, 30.5, 28.3, 28.0, 22.8, 14.1; HRMS (ESI) calcd for C20H30O3Na [(M + Na)+] 341.2087, found 341.2095.
α,β-Unsaturated ester 17. To a solution of MPM ether 16 (914.1 mg, 2.870 mmol) in degassed CH2Cl2 (28 mL) were added methyl acrylate (2.60 mL, 29.0 mmol) and a solution of the Grubbs second-generation catalyst (26.4 mg, 31.1 µmol) in degassed CH2Cl2 (1 mL + 1 mL rinse), and the resultant solution was stirred at room temperature for 14 h 50 min. The mixture was concentrated under reduced pressure. Purification of the residue by column chromatography (silica gel, 5 to 10% EtOAc/hexanes) gave α,β-unsaturated ester 17 (901.4 mg, 83%) as a colorless oil: [α]D24 +13.0 (c 1.00, CHCl3); IR (film) 2952, 2870, 1725, 1514, 1248, 1037, 822 cm–1; 1H NMR (600 MHz, CDCl3) δ 7.26–7.24 (m, 2H), 6.96 (ddd, J = 15.5, 7.3, 7.3 Hz, 1H) 6.86–6.83 (m, 2H), 5.89 (ddd, J = 15.5, 1.4, 1.4 Hz, 1H), 4.61 (d, J = 11.0 Hz, 1H), 4.51 (d, J = 11.0 Hz, 1H), 4.08–4.02 (m, 2H), 3.78 (s, 3H), 3.70 (s, 3H), 3.26 (m, 1H), 2.47 (dddd, J = 14.0, 6.7, 6.7, 1.4 Hz, 1H), 2.37 (dddd, J = 14.0, 6.9, 6.9, 1.4 Hz, 1H), 2.01 (m, 1H), 1.91 (m, 1H), 1.64 (m, 1H), 1.50 (m, 1H), 1.46–1.41 (m, 3H), 1.32–1.23 (m, 3H), 0.86 (t, J = 7.3 Hz, 3H); 13C NMR (150 MHz, CDCl3) δ 166.9, 159.0, 145.7, 131.3, 129.5 (2C), 122.8, 113.6 (2C), 81.4, 81.0, 77.5, 72.4, 55.3, 51.4, 38.5, 31.8, 30.5, 28.3, 27.9, 22.8, 14.1; HRMS (ESI) calcd for C22H32O5Na [(M + Na)+] 399.2142, found 399.2126.
Allylic alcohol 18. To a solution of α,β-unsaturated ester 17 (809.0 mg, 2.149 mmol) in CH2Cl2 (20 mL) at –78 ºC was added DIBALH (1.02 M in n-hexane, 6.30 mL, 6.43 mmol), and the resultant solution was stirred at –78 ºC for 35 min. The reaction was quenched with MeOH. The mixture was diluted with EtOAc and saturated aqueous potassium sodium tartrate solution, and the resultant biphasic mixture was vigorously stirred at room temperature until the layers became clear. The resultant mixture was diluted with EtOAc, and the organic layer was washed with H2O and brine, dried over Na2SO4, filtered, and concentrated under reduced pressure. Purification of the residue by column chromatography (silica gel, 10 to 30% EtOAc/hexanes) gave allylic alcohol 18 (734.2 mg, 98%) as a colorless oil: [α]D24 +17.4 (c 1.00, CHCl3); IR (film) 3420, 2954, 2932, 2861, 1612, 1513, 1247, 1085, 1036, 821 cm–1; 1H NMR (600 MHz, CDCl3) δ 7.26–7.24 (m, 2H), 6.86–6.83 (m, 2H), 5.73–5.66 (m, 2H), 4.64 (d, J = 11.0 Hz, 1H), 4.52 (d, J = 11.0 Hz, 1H), 4.08–4.06 (m, 2H), 4.04 (ddd, J = 8.3, 6.0, 6.0 Hz, 1H), 3.97 (dddd, J = 8.7, 6.4, 6.4, 6.4 Hz, 1H), 3.78 (s, 3H), 3.27 (m, 1H), 2.34 (m, 1H), 2.20 (m, 1H), 1.97 (m, 1H), 1.90 (m, 1H), 1.63 (m, 1H), 1.50 (m, 1H), 1.46–1.41 (m, 3H), 1.32–1.23 (m, 3H), 0.86 (t, J = 7.3 Hz, 3H), (one proton missing due to H/D exchange); 13C NMR (150 MHz, CDCl3) δ 159.0, 131.3, 131.2, 129.5 (2C), 129.2, 113.6 (2C), 81.13, 81.07, 78.6, 72.4, 63.7, 55.2, 38.5, 31.6, 30.4, 28.3, 27.9, 22.8, 14.1; HRMS (ESI) calcd for C21H32O4Na [(M + Na)+] 371.2193, found 371.2182.
Epoxy alcohol 19. To a mixture of allylic alcohol 18 (592.9 mg, 1.701 mmol), freshly activated 4 Å molecular sieves (1.06 g), and (+)-diethyl tartrate (113.4 mg, 0.550 mmol) in CH2Cl2 (20 mL) at –20 ºC was added Ti(Oi-Pr)4 (100 µL, 0.338 mmol), and the resultant mixture was stirred at –20 ºC for 30 min. To this mixture was added t-BuOOH (4.43 M solution in isooctane, 800 µL, 3.54 mmol), and the resultant mixture was stirred at –20 ºC for 4 h 20 min. The reaction mixture was diluted with tert-butyl methyl ether (TBME, 10 mL) and treated with 1 M aqueous NaOH solution (30 mL) at 0 ºC. The resultant biphasic mixture was vigorously stirred at 0 ºC for 1 h. The resultant mixture was filtered through a pad of Celite. The filtrate was diluted with TBME, washed with H2O and brine, dried over Na2SO4, filtered, and concentrated under reduced pressure. Purification of the residue by column chromatography (silica gel, 30 to 40% EtOAc/hexanes) gave epoxy alcohol 19 (576.0 mg, 93%, dr >20:1 as judged by 600 MHz 1H NMR) as a colorless oil: [α]D24 –6.9 (c 1.00, CHCl3); IR (film) 3434, 2954, 2934, 2869, 1612, 1512, 1465, 1248, 1086, 1035, 822 cm–1; 1H NMR (600 MHz, CDCl3) δ 7.28–7.26 (m, 2H), 6.86–6.83 (m, 2H), 4.64 (d, J = 11.0 Hz, 1H), 4.53 (d, J = 11.0 Hz, 1H), 4.12 (dddd, J = 8.7, 8.7, 6.0, 5.0 Hz, 1H), 4.01 (ddd, J = 8.7, 6.4, 6.4 Hz, 1H), 3.86 (m, 1H), 3.78 (s, 3H), 3.60 (m, 1H), 3.27 (m, 1H), 3.08 (ddd, J = 7.3, 5.0, 2.3 Hz, 1H), 2.94 (ddd, J = 5.0, 2.3, 2.3 Hz, 1H), 2.06 (m, 1H), 1.92 (m, 1H), 1.81 (ddd, J = 13.7, 8.7, 5.0 Hz, 1H), 1.67–1.61 (m, 3H), 1.52 (m, 1H), 1.45–1.38 (m, 3H), 1.32–1.23 (m, 3H), 0.86 (t, J = 7.3 Hz, 3H); 13C NMR (150 MHz, CDCl3) δ 159.0, 131.3, 129.4 (2C), 113.6 (2C), 81.3, 81.1, 76.4, 72.5, 61.7, 58.6, 55.2, 53.8, 38.2, 32.3, 30.6, 28.5, 27.8, 22.8, 14.0; HRMS (ESI) calcd for C21H32O5Na [(M + Na)+] 387.2142, found 387.2161.
Secondary allylic alcohol 20. To a solution of epoxy alcohol 19 (557.6 mg, 1.530 mmol) in THF (15 mL) were added imidazole (0.21 g, 3.1 mmol), PPh3 (0.72 g, 2.8 mmol), and I2 (0.71 g, 2.8 mmol), and the resultant solution was stirred at room temperature for 30 min. The reaction was quenched with saturated aqueous Na2SO3 solution at 0 ºC. The resultant mixture was extracted with EtOAc, and the organic layer was washed with H2O and brine, dried over Na2SO4, filtered, and concentrated under reduced pressure to give crude iodide, which was used in the next reaction without further purification.
To a solution of the above iodide in EtOH (15 mL) were added zinc powder (1.01 g, 15.4 mmol) and AcOH (0.18 mL, 3.1 mmol), and the resultant mixture was stirred at room temperature for 30 min. The reaction was quenched with saturated aqueous NaHCO3 solution at 0 ºC. The resultant mixture was filtered through a pad of Celite, and the filtrate was diluted with EtOAc. The organic layer was washed with H2O and brine, dried over Na2SO4, filtered, and concentrated under reduced pressure. Purification of the residue by column chromatography (silica gel, 5 to 15% EtOAc/hexanes) gave secondary allylic alcohol 20 (508.3 mg, 95% for the two steps) as a colorless oil: [α]D24 +18.7 (c 1.00, CHCl3); IR (film) 3439, 2955, 2934, 2870, 1613, 1514, 1248, 1085, 1037, 821 cm–1; 1H NMR (600 MHz, CDCl3) δ 7.27–7.24 (m, 2H), 6.86–6.84 (m, 2H), 5.91 (ddd, J = 17.0, 10.6, 5.0 Hz, 1H), 5.29 (ddd, J = 17.0, 1.3, 1.3 Hz, 1H), 5.11 (ddd, J = 10.6, 1.3, 1.3 Hz, 1H), 4.62 (d, J = 11.0 Hz, 1H), 4.50 (d, J = 11.0 Hz, 1H), 4.37 (m, 1H), 4.22 (dddd, J = 8.7, 5.5, 3.7, 3.7 Hz, 1H), 4.06 (ddd, J = 8.2, 6.0, 6.0 Hz, 1H), 3.77 (s, 3H), 3.26 (m, 1H), 2.00 (m, 1H), 1.91 (m, 1H), 1.83–1.72 (m, 2H), 1.66–1.53 (m, 2H), 1.44–1.38 (m, 3H), 1.32–1.22 (m, 3H), 0.86 (t, J = 7.3 Hz, 3H), (one proton missing due to H/D exchange); 13C NMR (150 MHz, CDCl3) δ 159.0, 140.7, 131.1, 129.5 (2C), 114.0, 113.7 (2C), 81.7, 81.1, 76.6, 72.6, 70.7, 55.2, 40.8, 32.3, 30.6, 28.3, 27.8, 22.8, 14.0; HRMS (ESI) calcd for C21H32O4Na [(M + Na)+] 371.2193, found 371.2215.
Bis-MPM ether 21. To a solution of allylic alcohol 20 (467.1 mg, 1.340 mmol) in DMF (15 mL) at 0 ºC was added NaH (60% in mineral oil, 101.3 mg, 2.533 mmol), and the resultant mixture was stirred at room temperature for 30 min. To the resultant mixture at 0 ºC were added n-Bu4NI (49.4 mg, 134 µmol) and MPMCl (270 µL, 1.98 mmol), and the resultant mixture was stirred at room temperature for 9 h 15 min. The reaction was quenched with saturated aqueous NH4Cl solution at 0 °C. The resultant mixture was extracted with TBME, and the organic layer was washed with H2O and brine, dried over Na2SO4, filtered, and concentrated under reduce pressure. Purification of the residue by column chromatography (silica gel, 5 to 10% EtOAc/hexanes) gave bis-MPM ether 21 (585.6 mg, 93%) as a colorless oil: [α]D24 –15.9 (c 1.00, CHCl3); IR (film) 2954, 2934, 2862, 1613, 1512, 1247, 1037, 821 cm–1; 1H NMR (600 MHz, CDCl3) δ 7.27–7.21 (m, 4H), 6.84–6.80 (m, 4H), 5.75 (ddd, J = 17.4, 10.6, 7.8 Hz, 1H), 5.22 (ddd, J = 17.4, 1.8, 0.9 Hz, 1H), 5.18 (ddd, J = 10.6, 1.8, 0.9 Hz, 1H), 4.66 (d, J = 11.0 Hz, 1H), 4.50 (d, J = 11.0 Hz, 1H), 4.49 (d, J = 11.0 Hz, 1H), 4.27 (d, J = 11.0 Hz, 1H), 4.17 (dddd, J = 8.2, 5.5, 4.1, 4.1 Hz, 1H), 4.02–3.96 (m, 2H), 3.77 (s, 3H), 3.74 (s, 3H), 3.26 (m, 1H), 2.00 (m, 1H), 1.90 (m, 1H), 1.74 (m, 1H), 1.67 (m, 1H), 1.63–1.56 (m, 2H), 1.48–1.38 (m, 3H), 1.32–1.22 (m, 3H), 0.86 (t, J = 7.3 Hz, 3H); 13C NMR (150 MHz, CDCl3) δ 159.0, 139.2, 131.5, 130.8, 129.5 (2C), 129.3 (2C), 116.5, 113.7 (2C), 113.6 (2C), 81.4, 81.3, 78.1, 75.5, 72.6, 71.4, 70.2, 55.25, 55.20, 42.2, 32.5, 30.7, 28.7, 27.9, 22.8, 14.1; HRMS (ESI) calcd for C29H40O5Na [(M + Na)+] 491.2768, found 491.2787.
Aldehyde 5. To a solution of olefin 21 (17.3 mg, 37.0 µmol) in THF/H2O (1:1, v/v, 0.8 mL) were added OsO4 (10 mg/mL solution in t-BuOH, 45 µL, 1.8 µmol) and NMO (4.8 M solution in H2O, 25 µL, 0.12 mmol), and the resultant mixture was stirred at room temperature for 15.5 h. To the reaction mixture was added NaIO4 (22.3 mg, 104 µmol), and the resultant mixture was stirred at room temperature for 50 min. The resultant mixture was treated with saturated aqueous Na2SO3 solution and stirred at room temperature for 40 min. The reaction mixture was diluted with EtOAc, washed with saturated aqueous NaHCO3 solution and brine, dried over Na2SO4, filtered, and concentrated under reduced pressure. Purification of the residue by column chromatography (silica gel, 10 to 20% EtOAc/hexanes) gave aldehyde 5 (15.7 mg, 90%) as a colorless oil: [α]D24 –19.8 (c 1.00, CHCl3); IR (film) 2954, 2868, 1732, 1613, 1513, 1249, 1088, 1035, 821 cm–1; 1H NMR (600 MHz, CDCl3) δ 9.61 (d, J = 1.8 Hz, 1H), 7.26–7.24 (m, 4H), 6.86–6.82 (m, 4H), 4.63 (d, J = 11.0 Hz, 1H), 4.59 (d, J = 11.0 Hz, 1H), 4.49 (d, J = 11.0 Hz, 1H), 4.49 (d, J = 11.0 Hz, 1H), 4.20 (dddd, J = 9.2, 6.0, 3.7, 3.7 Hz, 1H), 4.04 (ddd, J = 9.7, 3.7, 1.8 Hz, 1H), 4.00 (ddd, J = 8.2, 5.9, 5.9 Hz, 1H), 3.77 (s, 3H), 3.75 (s, 3H), 3.26 (ddd, J = 5.9, 5.9, 5.9 Hz, 1H), 2.04 (m, 1H), 1.92 (m, 1H), 1.83 (ddd, J = 13.7, 9.2, 3.7 Hz, 1H), 1.73 (ddd, J = 13.7, 9.7, 3.7 Hz, 1H), 1.64 (m, 1H), 1.53 (m, 1H), 1.46–1.40 (m, 3H), 1.32–1.22 (m, 3H), 0.87 (t, J = 7.3 Hz, 3H); 13C NMR (150 MHz, CDCl3) δ 203.1, 159.4, 159.0, 131.3, 129.7 (2C), 129.5, 129.4 (2C), 113.9 (2C), 113.6 (2C), 81.6, 81.34, 81.30, 74.8, 72.8, 72.5, 55.2, 55.2, 36.4, 32.3, 30.7, 28.7, 27.8, 22.8, 14.1; HRMS (ESI) calcd for C28H38O6Na [(M + Na)+] 493.2561, found 493.2544.
Enone 22. To a solution of β-keto phosphonate 4 (90.0 mg, 0.203 mmol) in MeCN (1.0 mL) were added flame dried LiCl (15.3 mg, 0.361 mmol) and i-Pr2NEt (60 µL, 0.34 mmol), and the resultant solution was stirred at room temperature for 15 min. To the mixture was added a solution of aldehyde 5 (77.9 mg, 0.166 mmol) in MeCN (0.5 mL + 0.5 mL rinse), and the resultant solution was stirred at room temperature for 13.5 h. The reaction was quenched with saturated aqueous NH4Cl solution at 0 °C. The resultant mixture was extracted with EtOAc, and the organic layer was washed with H2O and brine, dried over Na2SO4, filtered, and concentrated under reduced pressure. The residue was purified by column chromatography (silica gel, 10% EtOAc/hexanes) to give enone 22 (123.1 mg, 94%, E/Z >20:1 as judged by 600 MHz 1H NMR) as a colorless oil: [α]D24 –21.5 (c 1.00, CHCl3); IR (film) 2953, 2935, 2873, 1514, 1248, 1084, 1036, 742 cm–1; 1H NMR (600 MHz, CDCl3) δ 7.30–7.23 (m, 7H), 7.19–7.17 (m, 2H), 6.85–6.81 (m, 3H), 6.79–6.77 (m, 2H), 6.72 (dd, J = 15.6, 0.9 Hz, 1H), 5.86 (ddd, J = 17.0, 10.1, 6.4 Hz, 1H), 5.21 (ddd, J = 17.0, 1.4, 1.4 Hz, 1H), 5.10 (ddd, J = 10.1, 1.4, 1.4 Hz, 1H), 4.64 (d, J = 12.0 Hz, 1H), 4.64 (d, J = 11.0 Hz, 1H), 4.48 (d, J = 11.0 Hz, 1H), 4.45 (d, J = 11.0 Hz, 1H), 4.42 (d, J = 12.0 Hz, 1H), 4.41 (m, 1H), 4.26 (d, J = 11.0 Hz, 1H), 4.24–4.18 (m, 2H), 3.99 (ddd, J = 8.7, 6.4, 6.4 Hz, 1H), 3.87 (d, J = 4.6 Hz, 1H), 3.76 (s, 3H), 3.73 (s, 3H), 3.25 (ddd, J = 6.4, 6.4, 6.4 Hz, 1H), 2.00 (m, 1H), 1.89 (m, 1H), 1.71–1.40 (m, 6H), 1.30–1.23 (m, 4H), 0.90–0.85 (m, 12H), 0.53 (q, J = 8.0 Hz, 6H); 13C NMR (150 MHz, CDCl3) δ 199.8, 159.2, 159.0, 147.3, 137.3, 137.2, 131.4, 130.2, 129.5 (2C), 129.3 (2C), 128.3 (2C), 128.0 (2C), 127.8, 126.2, 116.4, 113.8 (2C), 113.6 (2C), 87.7, 81.3, 76.8, 76.2, 75.3, 75.1, 72.9, 72.6, 71.3, 55.24, 55.19, 41.6, 32.5, 30.7, 28.7, 27.9, 22.8, 14.1, 6.8 (3C), 4.8 (3C); HRMS (ESI) calcd for C47H66O8SiNa [(M + Na)+] 809.4419, found 809.4434.
Ketone 23. To a solution of [CuH(PPh3)]6 (68.3 mg, 34.8 µmol) in toluene (1.0 mL) was added a solution of enone 22 (69.1 mg, 87.7 µmol) in toluene (0.4 mL + 0.4 mL rinse), and the resultant solution was stirred at room temperature for 5 min. To this solution was added H2O (deoxygenated by bubbling N2 gas, 5 µL), and the resultant mixture was stirred at room temperature for 14 h 40 min. After being stirred for 1 h under air, the reaction mixture was diluted with EtOAc and filtered through a pad of Celite. The filtrate was concentrated under reduced pressure. Purification of the residue by column chromatography (silica gel, 5 to 10% EtOAc/hexanes) gave ketone 23 (65.7 mg, 95%) as a colorless oil: [α]D24 –20.0 (c 1.00, CHCl3); IR (film) 2954, 2935, 2874, 1716, 1613, 1514, 1248, 1085, 1036, 822, 744 cm–1; 1H NMR (600 MHz, CDCl3) δ 7.31–7.24 (m, 7H), 7.20–7.19 (m, 2H), 6.83–6.81 (m, 2H), 6.80–6.77 (m, 2H), 5.85 (ddd, J = 17.0, 10.6, 6.4 Hz, 1H), 5.21 (ddd, J = 17.0, 1.4, 1.4 Hz, 1H), 5.09 (ddd, J = 10.6, 1.4, 1.4 Hz, 1H), 4.65 (d, J = 11.0 Hz, 1H), 4.59 (d, J = 12.0 Hz, 1H), 4.49 (d, J = 11.0 Hz, 1H), 4.44 (d, J = 11.0 Hz, 1H), 4.41 (d, J = 12.0 Hz, 1H), 4.41 (d, J = 11.0 Hz, 1H), 4.38 (ddd, J = 6.4, 4.6, 1.4 Hz, 1H), 4.13 (m, 1H), 4.00 (ddd, J = 8.7, 6.4, 6.4 Hz, 1H), 3.78 (d, J = 4.6 Hz, 1H), 3.76 (s, 3H), 3.73 (s, 3H), 3.62 (dddd, J = 9.4, 5.5, 5.5, 5.5 Hz, 1H), 3.25 (ddd, J = 6.4, 6.4, 6.4 Hz, 1H), 2.68 (ddd, J = 18.3, 10.0, 5.5 Hz, 1H), 2.49 (ddd, J = 18.3, 10.0, 5.5 Hz, 1H), 2.00 (m, 1H), 1.90 (m, 1H), 1.81 (m, 1H), 1.72 (m, 1H), 1.66–1.53 (m, 4H), 1.45–1.40 (m, 3H), 1.31–1.23 (m, 3H), 0.88 (t, J = 7.8 Hz, 9H), 0.86 (t, J = 7.3 Hz, 3H), 0.54 (q, J = 7.8 Hz, 6H); 13C NMR (150 MHz, CDCl3) δ 210.9, 159.00, 158.97, 137.4, 137.1, 131.4, 131.0, 129.4 (2C), 129.3 (2C), 128.3 (2C), 128.0 (2C), 127.9, 116.2, 113.7 (2C), 113.6 (2C), 87.9, 81.5, 81.2, 75.9, 75.7, 75.0, 73.3, 72.6, 71.1, 55.23, 55.21, 41.1, 36.2, 32.7, 30.7, 28.6, 27.9, 27.3, 22.8, 14.1, 6.8 (3C), 4.8 (3C); HRMS (ESI) calcd for C47H68O8SiNa [(M + Na)+] 811.4576, found 811.4586.
Alcohol 24. To a solution of ketone 23 (27.2 mg, 34.5 µmol) in Et2O (0.3 mL) at –78 °C was added Zn(BH4)2 (0.5 M solution in Et2O, 0.28 mL, 0.14 mmol). The resultant solution was stirred at –78 °C and allowed to warm to –40 ºC over a period of 2 h. The reaction was quenched with saturated aqueous NH4Cl solution. The resultant mixture was extracted with EtOAc, and the organic layer was washed with H2O and brine, dried over Na2SO4, filtered, and concentrated under reduced pressure. Purification of the residue by column chromatography (silica gel, 15% EtOAc/hexanes) gave alcohol 24 (21.8 mg, 80%, dr >20:1 as judged by 600 MHz 1H NMR) as a colorless oil: [α]D23 +15.1 (c 1.00, CHCl3); IR (film) 3502, 2954, 2935, 2874, 1514, 1247, 1076, 1036, 821, 743 cm–1; 1H NMR (600 MHz, CDCl3) δ 7.32–7.20 (m, 9H), 6.84–6.81 (m, 2H), 6.77–6.75 (m, 2H), 6.03 (ddd, J = 17.4, 10.6, 5.5 Hz, 1H), 5.31 (ddd, J = 17.4, 1.4, 1.4 Hz, 1H), 5.23 (ddd, J = 10.6, 1.4, 1.4 Hz, 1H), 4.68 (d, J = 11.0 Hz, 1H), 4.61 (d, J = 11.9 Hz, 1H), 4.59 (d, J = 11.9 Hz, 1H), 4.51 (d, J = 11.0 Hz, 1H), 4.48 (d, J = 11.0 Hz, 1H), 4.40 (d, J = 11.0 Hz, 1H), 4.39 (m, 1H), 4.17 (dddd, J = 13.8, 8.9, 5.0, 5.0 Hz, 1H), 4.01 (ddd, J = 8.7, 6.4, 6.4 Hz, 1H), 3.76 (s, 3H), 3.73 (s, 3H), 3.72 (m, 1H), 3.66 (m, 1H), 3.26 (ddd, J = 6.4, 6.4, 6.4 Hz, 1H), 3.24 (dd, J = 7.8, 4.1 Hz, 1H), 1.99 (m, 1H), 1.89 (m, 1H), 1.82–1.76 (m, 2H), 1.74–1.58 (m, 4H), 1.48–1.39 (m, 3H), 1.34–1.23 (m, 6H), 0.92 (t, J = 7.8 Hz, 9H), 0.86 (t, J = 7.3 Hz, 3H), 0.55 (q, J = 7.8 Hz, 6H); 13C NMR (150 MHz, CDCl3) δ 159.0, 158.9, 138.1, 136.2, 131.5, 131.2, 129.4 (2C), 129.3 (2C), 128.4 (2C), 128.0 (2C), 127.8, 116.3, 113.6 (2C), 113.6 (2C), 82.3, 81.4, 81.1, 76.5, 76.1, 74.2, 73.6, 72.6, 71.6, 71.0, 55.22, 55.19, 41.1, 32.7, 30.7, 29.9, 28.6, 28.5, 27.9, 22.8, 14.1, 6.7 (3C), 4.6 (3C); HRMS (ESI) calcd for C47H70O8SiNa [(M + Na)+] 813.4732, found 813.4738.
Diol 25. To a solution of alcohol 24 (10.1 mg, 12.8 µmol) in MeOH/CH2Cl2 (1:1, v/v, 0.5 mL) was added CSA (1.18 mg, 5.07 µmol), and the resultant solution was stirred at room temperature for 2 h 50 min. The reaction was quenched with Et3N, and the resultant mixture was concentrated under reduced pressure. Purification of the residue by column chromatography (silica gel, 20 to 40% EtOAc/hexanes) gave diol 25 (8.67 mg, quant) as a colorless oil: [α]D24 +22.2 (c 1.00, CHCl3); IR (film) 3421, 2953, 2933, 2869, 1612, 1514, 1248, 1072, 1036 cm–1; 1H NMR (600 MHz, CDCl3) δ 7.32–7.20 (m, 9H), 6.83–6.82 (m, 2H), 6.80–6.79 (m, 2H), 5.99 (ddd, J = 17.0, 10.6, 5.0 Hz, 1H), 5.35 (ddd, J = 17.0, 1.4, 1.4 Hz, 1H), 5.20 (ddd, J = 10.6, 1.4, 1.4 Hz, 1H), 4.64–4.56 (m, 3H), 4.51 (d, J = 11.0 Hz, 1H), 4.49 (d, J = 11.0 Hz, 1H), 4.42 (d, J = 11.0 Hz, 1H), 4.37 (m, 1H), 4.12 (m, 1H), 4.01 (ddd, J = 8.3, 6.4, 6.4 Hz, 1H), 3.76 (s, 3H), 3.74 (s, 3H), 3.73 (m, 1H), 3.64 (m, 1H), 3.29–3.25 (m, 2H), 2.02 (m, 1H), 1.91 (m, 1H), 1.82–1.60 (m, 9H), 1.50–1.39 (m, 4H), 1.34–1.24 (m, 3H), 0.86 (t, J = 7.3 Hz, 3H); 13C NMR (150 MHz, CDCl3) δ 159.2, 159.0, 137.9 (2C), 131.3, 130.4, 129.6 (2C), 129.4 (2C), 128.4 (2C), 128.0 (2C), 127.9, 115.8, 113.8 (2C), 113.6 (2C), 82.8, 81.4, 81.1, 76.8, 76.2, 73.4, 72.5, 72.19, 72.16, 71.3, 55.2 (2C), 40.8, 32.8, 30.65, 30.59, 28.9, 28.6, 27.8, 22.8, 14.1; HRMS (ESI) calcd for C41H56O8Na [(M + Na)+] 699.3867, found 699.3882.
Bis-TBS ether 3. To a solution of diol 25 (39.3 mg, 57.9 µmol) in CH2Cl2 (0.8 mL) at 0 °C were added 2,6-lutidine (30 µL, 0.26 mmol) and TBSOTf (40 µL, 0.17 mmol), and the resultant solution was stirred at 0 °C for 65 min. The reaction was quenched with saturated aqueous NaHCO3 solution at 0 °C. The resultant mixture was extracted with EtOAc, and the organic layer was washed with H2O and brine, dried over Na2SO4, filtered, and concentrated under reduced pressure. Purification of the residue by column chromatography (silica gel, 5% EtOAc/hexanes) gave bis-TBS ether 3 (47.1 mg, 90%) as a colorless oil: [α]D24 –2.4 (c 1.00, CHCl3); IR (film) 2954, 2930, 2856, 1514, 1249, 1069, 1038, 835, 776 cm–1; 1H NMR (600 MHz, CDCl3) δ 7.36–7.35 (m, 2H), 7.30–7.19 (m, 7H), 6.83–6.81 (m, 2H), 6.80–6.77 (m, 2H), 5.88 (ddd, J = 17.0, 10.6, 6.9 Hz, 1H), 5.21 (ddd, J = 17.0, 1.4, 1.4 Hz, 1H), 5.09 (ddd, J = 10.6, 1.4, 1.4 Hz, 1H), 4.83 (d, J = 11.0 Hz, 1H), 4.68 (d, J = 11.0 Hz, 1H), 4.65 (d, J = 11.0 Hz, 1H), 4.51 (d, J = 11.0 Hz, 1H), 4.45 (d, J = 11.0 Hz, 1H), 4.37 (d, J = 11.0 Hz, 1H), 4.17 (m, 1H), 4.12 (dd, J = 6.9, 6.9 Hz, 1H), 4.03 (ddd, J = 8.2, 6.4, 6.4 Hz, 1H), 3.77 (m, 1H), 3.76 (s, 3H), 3.74 (s, 3H), 3.64 (m, 1H), 3.41 (dd, J = 6.9, 1.4 Hz, 1H), 3.28 (ddd, J = 6.4, 6.4, 6.4 Hz, 1H), 1.99 (m, 1H), 1.89 (m, 1H), 1.82–1.56 (m, 6H), 1.52–1.38 (m, 4H), 1.30–1.24 (m, 4H), 0.89 (s, 9H), 0.86 (m, 12H), 0.05 (s, 3H), 0.03 (s, 3H), –0.02 (s, 3H), –0.03 (s, 3H); 13C NMR (150 MHz, CDCl3) δ 159.0 (2C), 139.5, 138.4, 131.4, 131.2, 129.4 (2C), 129.2 (2C), 128.1 (2C), 127.5 (2C), 127.1, 115.5, 113.7 (2C), 113.6 (2C), 87.5, 81.4, 81.1, 76.6, 76.1, 74.8, 74.3, 73.7, 72.6, 71.0, 55.23, 55.21, 41.1, 32.8, 31.2, 30.6, 28.6, 27.9, 27.4, 25.9 (6C), 22.8, 18.2, 18.0, 14.1, –3.9, –4.6, –4.7 (2C); HRMS (ESI) calcd for C53H84O8NaSi2 [(M + Na)+] 927.5597, found 927.5595.
Acetonide 26. To a solution of diol 25 (8.7 mg, 13 µmol) in (CH2Cl)2 (0.5 mL) at 0 °C were added PPTS (3.2 mg, 13 µmol) and 2,2-dimethoxypropane (50 µL, 0.41 mmol), and the resultant solution was stirred at room temperature for 11 h. The reaction was quenched with saturated aqueous NaHCO3 solution. The resultant mixture was extracted with EtOAc, and the organic layer was washed with H2O and brine, dried over Na2SO4, filtered, and concentrated under reduced pressure. Purification of the residue by column chromatography (silica gel, 5 to 10% EtOAc/hexanes) gave acetonide 26 (8.1 mg, 88%) as a colorless oil: [α]D23 +5.2 (c 1.00, CHCl3); IR (film) 2933, 2868, 1612, 1513, 1247, 1083, 1036, 821 cm–1; 1H NMR (600 MHz, CDCl3) δ 7.26–7.16 (m, 9H), 6.80–6.78 (m, 2H), 6.75–6.74 (m, 2H), 6.02 (ddd, J = 17.4, 10.6, 7.3 Hz, 1H), 5.36 (d, J = 17.4 Hz, 1H), 5.24 (d, J = 10.6 Hz, 1H), 4.63 (d, J = 11.0 Hz, 1H), 4.56 (d, J = 11.5 Hz, 1H), 4.48 (d, J = 11.0 Hz, 1H), 4.41 (d, J = 11.0 Hz, 1H), 4.37 (d, J = 11.0 Hz, 1H), 4.34 (d, J = 11.5 Hz, 1H), 4.31 (dd, J = 7.3, 4.1 Hz, 1H), 4.12 (m, 1H), 3.97 (ddd, J = 6.4, 6.4, 6.4 Hz, 1H), 3.73 (s, 3H), 3.70 (s, 3H), 3.61–3.57 (m, 2H), 3.34 (dd, J = 7.3, 4.1 Hz, 1H), 3.23 (ddd, J = 6.4, 6.4, 6.4 Hz, 1H), 1.96 (m, 1H), 1.87 (m, 1H), 1.82–1.38 (m, 11H), 1.34 (s, 3H), 1.28 (s, 3H), 1.28–1.20 (m, 3H), 0.83 (t, J = 7.3 Hz, 3H); 13C NMR (150 MHz, CDCl3) δ 159.0 (2C), 138.1, 134.3, 131.4, 131.1, 129.4 (2C), 129.3 (2C), 128.3 (2C), 127.9 (2C), 127.7, 117.8, 113.7 (2C), 113.6 (2C), 100.8, 82.7, 81.5, 81.2, 76.2, 76.0, 73.6, 73.3, 72.6, 72.0, 71.2, 55.24, 55.22, 41.2, 32.7, 30.7, 30.5, 28.7, 28.6, 27.9, 24.9, 24.0, 22.8, 14.1; HRMS (ESI) calcd for C44H60O8Na [(M + Na)+] 739.4180, found 739.4164.
ACKNOWLEDGEMENTS
This study was financially supported by JSPS KAKENHI Grant Number 25282228. We are grateful to Dr. Eunsang Kwon (Tohoku University) for X-ray crystallographic analysis.
SUPPORTING INFORMATION
Proofs for the stereochemical assignment of compound 14, experimental procedure for compound 15, and 1H and 13C NMR spectra for all new compounds are available.
References
1. For a recent review, see: J. Kobayashi, J. Antibiot., 2008, 61, 271 and references cited therein. CrossRef
2. M. Ishibashi, N. Yamaguchi, T. Sasaki, and J. Kobayashi, J. Chem. Soc., Chem. Commun., 1994, 1455. CrossRef
3. Y. Takahashi, T. Kubota, M. Imachi, M. R. Wälchli, and J. Kobayashi, J. Antibiot., 2013, 66, 277. CrossRef
4. I. Bauer, L. Maranda, K. A. Young, and Y. Shimizu, J. Am. Chem. Soc., 1995, 60, 1084.
5. For recent total synthesis of amphidinolides, see: G. Valot, C. S. Regens, D. P. O’Malley, E. Godineau, H. Takikawa, and A. Fürstner, Angew. Chem. Int. Ed., 2013, 52, 9534; CrossRef S. Mahapatra and R. G. Carter, J. Am. Chem. Soc., 2013, 135, 10792; CrossRef M.-H. Hwang, S.-J. Han, and D.-H. Lee, Org. Lett., 2013, 15, 3381; CrossRef I. Volchkov and D. Lee, J. Am. Chem. Soc., 2013, 135, 5324; CrossRef L. Lu, W. Zhang, S. Nam, D. A. Horne, R. Jove, and R. G. Carter, J. Org. Chem., 2013, 78, 2213; CrossRef A. Hara, R. Morimoto, Y. Iwasaki, T. Saitoh, Y. Ishikawa, and S. Nishiyama, Angew. Chem. Int. Ed., 2012, 51, 9877; CrossRef S. Mahapatra and R. G. Carter, Angew. Chem. Int. Ed., 2012, 51, 7948; CrossRef A. Fürstner, Isr. J. Chem., 2011, 51, 329 and references cited therein. CrossRef
6. K. C. Nicolaou, W. E. Brenzovich, P. G. Bulger, and T. M. Francis, Org. Biomol. Chem., 2006, 4, 2119; CrossRef K. C. Nicolaou, P. G. Bulger, and W. E. Brenzovich, Org. Biomol. Chem., 2006, 4, 2158. CrossRef
7. B. M. Trost and J. Ley, Org. Lett., 2012, 14, 5632. CrossRef
8. G. Scheid, E. Ruijter, M. Konarzycka-Bessler, U. T. Bornscheuer, and L. A. Wessjohann, Tetrahedron: Asymmetry, 2004, 15, 2861. CrossRef
9. D. A. Evans, J. Bartroli, and T. L. Shih, J. Am. Chem. Soc., 1981, 103, 2127. CrossRef
10. A. Basha, M. Lipton, and S. M. Weinreb, Tetrahedron Lett., 1977, 18, 4171. CrossRef
11. The carbon numbering of compounds in this paper corresponds to that of amphidinolide N.
12. X. Lu, H. S. Byun, and R. Bittman, J. Org. Chem., 2004, 69, 5433. CrossRef
13. V. N. Odinokov, R. R. Vakhidov, R. N. Shakhmaev, and V. V. Zorin, Chem. Nat. Compd., 1998, 34, 186. CrossRef
14. G. E. Keck, K. H. Tarbet, and L. S. Geraci, J. Am. Chem. Soc., 1993, 115, 8467. CrossRef
15. I. Ohtani, T. Kusumi, Y. Kashman, and H. Kakisawa, J. Am. Chem. Soc., 1991, 113, 4092. CrossRef
16. H. C. Kolb, M. S. VanNieuwenhze, and K. B. Sharpless, Chem. Rev., 1994, 94, 2483. CrossRef
17. For closely related cyclizations, see: J. A. Marshall and J. J. Sabatini, Org. Lett., 2005, 7, 4819; CrossRef D. K. Mohapatra, P. Dasari, H. Rahaman, and R. Pal, Tetrahedron Lett., 2009, 50, 6276; CrossRef J. S. Yadav, K. Ramesh, U. V. S. Reddy, B. V. S. Reddy, and A. A. K. A. Ghamdi, Tetrahedron Lett., 2011, 52, 2943. CrossRef
18. The synthesis of the enantiomers of compounds 14 and 16 has previously been reported in the literature.6.
19. An added proof for the stereochemical assignment of compound 14 is provided in the Supporting Information.
20. Crystalline triol 15 was obtained as a minor byproduct under unoptimized conditions. For experimental procedure for compound 15, see the Supporting Information.
21. CCDC 1012729 contains the supplementary crystallographic data for this paper. These data can be obtained free of charge from the Cambridge Crystallographic Data Centre via: www.ccdc.cam.ac.uk/data_request/cif.
22. S. J. Connon and S. Blechert, Angew. Chem. Int. Ed., 2003, 42, 1900. CrossRef
23. M. Scholl, S. Ding, C. W. Lee, and R. H. Grubbs, Org. Lett., 1999, 1, 953. CrossRef
24. T. Katsuki and K. B. Sharpless, J. Am. Chem. Soc., 1980, 102, 5974; CrossRef R. M. Hanson and K. B. Sharpless, J. Org. Chem., 1986, 51, 1922. CrossRef
25. M. A. Blanchette, W. Choy, J. T. Davis, A. P. Essenfeld, S. Masamune, W. R. Roush, and T. Sakai, Tetrahedron Lett., 1984, 25, 2183. CrossRef
26. W. S. Mahoney, D. M. Brestensky, and J. M. Stryker, J. Am. Chem. Soc., 1988, 110, 291; CrossRef B. H. Lipshutz, J. Keith, P. Papa, and R. Vivian, Tetrahedron Lett., 1998, 39, 4627. CrossRef
27. T. Takahashi, M. Miyazawa, and J. Tsuji, Tetrahedron Lett., 1985, 26, 5139. CrossRef
28. T. Oishi and T. Nakata, Acc. Chem. Res., 1984, 17, 338. CrossRef
29. S. D. Rychnovsky and D. J. Skalitzky, Tetrahedron Lett., 1990, 31, 945. CrossRef
30. D. A. Evans, D. L. Rieger, and J. R. Gage, Tetrahedron Lett., 1990, 31, 7099. CrossRef