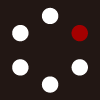
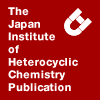
HETEROCYCLES
An International Journal for Reviews and Communications in Heterocyclic ChemistryWeb Edition ISSN: 1881-0942
Published online by The Japan Institute of Heterocyclic Chemistry
e-Journal
Full Text HTML
Received, 27th June, 2014, Accepted, 29th July, 2014, Published online, 8th August, 2014.
DOI: 10.3987/COM-14-S(K)49
■ Perylene-Based, Bis(terpyridine)-Ru(II) Complexes: Synthesis, Electrochemical and Photovoltaic Properties
Hany El-Batal, Juan Manríquez Rocha, Perla F. Méndez, Luis A. Godínez, Kai Guo, Xiaopeng Li, Xiaocun Lu, Chrys Wesdemiotis, Charles N. Moorefield, and George R. Newkome*
Departments of Polymer Science and Chemistry, The University of Akron, Akron, OH 44325-4717, U.S.A.
Abstract
Perylene-based, terpyridine-Ru(II) complexes are synthesized and their electrochemical and photoelectrochemical properties are studied; their fabrication into dye-sensitized solar cells are described (DSSCs) and their resultant photovoltaic properties are evaluated.INTRODUCTION
Perylenediimides (PDIs) have been widely studied due to their stability and attractive electronic properties.1-7 Elegant work by Würthner et al.8,9 has resulted in the construction of numerous functional supramolecular architectures using ionic, H-bonding, π-π, and metal-ligand directed self-assembly.10,11 Terpyridine-metal-terpyridine [<tpy-M2+-tpy>, where tpy = 2,2':6',2"-terpyridine] connectivity12,13 is an attractive approach to building functional materials due to the rich coordination chemistry and their incorporation of redox, photophysical, and electrochemical properties into the resulting supramolecules. As a result, terpyridine coordination has permitted the exploration of diverse materials used in construction of solar devices.14,15 Their incorporation into a dendritic shell has given rise to unimolecular micelles16-20 and bolaamphiphiles.9,21 This, along with the unique properties of perylene provided the inspiration to synthesize perylene-containing tpy ligands7 and their RuII complexes. Herein, we report the synthesis of heteroleptic, bis(terpyridinyl)perylene RuII complexes and their use in the fabrication of dye-sensitized solar cell (DSSC) devices.
RESULTS AND DISCUSSION
Synthesis began with the construction of aminoterpyridines 2 and 4,22 which were obtained from the reduction of the corresponding known nitroterpyridines23 using Pd/C and hydrazine hydrate. The reaction of perylenetetracarboxylic acid dianhydride (1) with 2 gave (51%) the linear bis(terpyridine)perylene ligand24 3 (Scheme 1), which was confirmed with 1H NMR by absorptions at 8.79 (3',5'-tpyH), 8.74 (6,6"-tpyH), 8.30 (perH), and 1.27 ppm [C(CH3)3] as well as with electrospray ionization mass spectrometry (ESI-MS) by a peak at m/z 1597.38 (calcd. [M+H]+ = 1597.64). The isomeric 525 was similarly synthesized (60%) and exhibited 1H NMR peaks at 8.76 (3',5'-tpyH), 8.70 (6,6"-tpyH), 8.30 (perH), and 1.27 ppm [C(CH3)3] as well as ESI-MS peaks at m/z 1596.71 (calcd. [M]+ = 1596.64).
The known RuIII adduct 724 was obtained (88%) from terpyridine 6 by reaction with RuCl3·nH2O (Scheme 1). Complexes 8 and 9 were subsequently generated from adduct 7 (2.2 eq.) by treatment with bisterpyridine ligands 3 and 5, respectively, in a MeOH:CHCl3 mixture and N-ethylmorpholine, as a reducing agent. After counter ion exchange to give the PF6ˉ salt, the desired complexes were isolated (78%), as dark red solids.
The 1H NMR spectrum for bis-complex 8 revealed a pair of singlets at 9.53 (3',5'-tpyHa) and 9.45 (3',5'-tpyHb) ppm [where, “a = inner” tpyHs and “b = outer” tpyHs; Scheme 1] that, as expected, were shifted downfield (Δδ = +0.65 Hz) in comparison to that of the free ligands 3 and 6. It also revealed a multiplet at 9.14 (3,3"-tpyHa and 3,3"-tpyHb) ppm, a peak at 7.80 (6,6"-tpyHa and 6,6"-tpyHb) ppm, with a significant upfield shift resulting from the ring current shielding effect upon complexation, as well as two singlets with an integration ratio of 1:6 at 2.90 (Ph-CH3) and 1.66 [C(CH3)3] ppm. In the case of the isomeric 9, the 1H NMR spectrum showed three singlets with an integration ratio of 1:1 at 9.04 (3',5'-tpyHa), 9.02 (3',5'-tpyHb), and 8.18 (perH) ppm along with two doublets at 8.66 (3,3"-tpyHa) and 8.63 (3,3"-tpyHb) ppm. The spectrum also revealed a multiplet at 7.41 (6,6"-tpyHa and 6,6"-tpyHb) ppm, in addition to two singlets at 2.57 (Ph-CH3) and 1.32 [C(CH3)3] ppm, with an integration ratio of 1:6, respectively. The structure was further confirmed by MALDI-TOF-MS peaks at m/z 2881.01 [M-PF6ˉ]1+ (Calcd. m/z = 2881.2) for 9.
In contrast, complex 13 was synthesized via a Suzuki coupling of dibromide 10 (Scheme 2) with 4'-terpyridinylphenylboronic acid (11) in a solvent mixture of toluene:H2O:t-BuOH (3:3:1) at 100 °C for 3 days. The solvent was evaporated in vacuo and the residue was column chromatographed (Al2O3) eluting with CHCl3 and then recrystallized (5x) CHCl3:MeOH (1:1 v/v) to eliminate the isomeric 1,6-bis(terpyridine)-substituted perylene to afford (55 %) the pure 1,7-bis(terpyridine)perylene ligand 12, as a red solid. The 1H NMR spectrum of 12 showed the following resonances: 8.88 (3',5'-tpyH), 8.79 (6,6"-tpyH), 8.74 (3,3"-tpyH), and 8.69 ppm (perH), in addition to the ESI-MS peak at m/z 1229.64 [M+H]+ (Calcd. m/z = 1229.54). Finally, bisterpyridine 12 was reacted with 7 in refluxing EtOH/CHCl3 (2:1) solution with a catalytic amount of N-ethylmorpholine for 12 h, followed by counter ion exchange (NO3ˉ to PF6ˉ) to afford (78%) 13, as a dark red solid. The 1H NMR of bis-complex 13 exhibited three singlets at 9.35 (3',5'-tpyHa) and 9.18 (3',5'-tpyHb), and 8.69 (perH) ppm, two doublets at 8.91 (3,3"-tpyHa) and 8.83 (3,3"-tpyHb) ppm, and a multiplet at 7.54 (6,6"-tpyHa and 6,6"-tpyHb) ppm. A singlet at 2.54 ppm was assigned to the Ph-CH3 moieties. ESI-MS further confirmed 13 with peaks at m/z 519.70 [M-4PF6ˉ]4+ (Calcd. m/z = 519.65), 741.28 [M-3PF6ˉ]3+ (Calcd. 741.19). Finally, complex 14 (Figure 1) was synthesized according to the literature procedure.
ELECTROCHEMISTRY
The CV responses of complexes 8 and 9 (Figure 2A and 2B, respectively) each show two reversible waves between -2.2 and -1.4 V associated with one-electron consecutive reductions for the two pairs of inner and outer tpy moieties.26 Two additional reversible waves were also observed between -1.3 and -0.6 V that are associated with two-electron consecutive reduction processes for perylene.27 Similarly, CV responses for 14 and 13 (Figures 2C and 2D, respectively) show irreversible waves for the consecutive reduction events previously described for the tpy moieties (from -2.2 to -1.4 V) and perylene (from -1.3 to -0.6 V).
Comparison of the cathodic behavior for the isomers 8 and 9 to that of 13 and 14 (Figures 2A and 2B vs 2C and 2D) strongly suggests that the reversible electrochemical responses for tpy and perylene moieties are observed only if the π-orbitals of these two types of groups are able to communicate electronically through the peri-positions on the perylene units.27 Inspection of the oxidation processes (Figures 2A and 2B) reveals consecutive one-electron oxidations for the perylene and phenoxy moieties between 0 and +0.50 V and a one-electron oxidation for the <tpyRuIItpy> moieties between +0.50 and +0.75 V, respectively.25
Thus, the low intensities observed for these two types of oxidation could be associated with very short life-times for the perylene radical anions due to favorable e-transfer to RuIIItpy units. In contrast, a comparison between Figures 2C and 2D show irreversible overlapped signals between +0.6 and +1.4 V, possibly due to the consecutive oxidation reactions previously described for perylene and <tpyRuIItpy>.
PHOTOELECTROCHEMISTRY
As predicted by their electrochemical responses, a comparison between Figures 3A and 3B, indicates that the output power obtained from DSSC assembled with 14 or 13 are higher than for 8 or 9. This behaviour is reflected by the photovoltaic parameters compiled in Table 1; whereas, the global photovoltaic efficiencies (η) follow the same tendencies. Despite the well-known photophysical and electrochemical properties of both <tpyRuIItpy> and perylene, the observed low efficiencies of the DSSC can be attributed to the presence of the two strong electron-withdrawing imide groups leading to a non-directional transfer7 of the generated photoelectrons. As well, for all of the dyes studied, there is an absence of anchoring groups (i.e., CO2H) that are known to promote an efficient injection of photo-generated electrons into TiO2 photoanodes.29
Photo-stability for the sensitizers was studied by means of open-circuit-potential transients (ocp vs. time plots) obtained for all the DSSC upon repetitive illumination-dark (ON-OFF) cycles. Figures 4-i and 4-ii show the ocp transients obtained for 8 and 9 (Figures 4-iA and 4-iB) and for 14 and 13 (Figures 4-iiA and 4-iiB), respectively.
These results indicate that the ocp for the DSSC containing 8 (Figure 4-iA) or 14 (Figure 4-iiA) is constant under illumination, as function of time; however, the ocp for photocells sensitized by 9 (Figure 4-iB) and 13 (Figure 4-iiB) is rapidly deactivated under the same condition. These last results indicate that the oxidized states for the dyes 9 and 13 are not efficiently reduced by I¯ ions contained in the cell electrolyte.
To support the results shown in Figures 4-iA and 4-iiA, steady-state-photocurrent spectra (IPCE vs. wavelength plots, Figure 5) were obtained and compared for the DSSCs containing the dyes. The photocurrents subsequently obtained from photocells constructed with 8 and 14 predominately resulted from injection by perylene at 470, 500, and 550 nm, respectively.30 Finally, the spectra shown in Figure 5 demonstrates that <tpyRuIItpy> units contained in 8 and 14 have no direct contribution to the photocurrent generation, thereby suggesting that only perylene groups can establish a successful electronic connection with Ti bonding orbitals in the TiO2 photoanodes.
In summary, dyes 8, 9, 13, and 14 were synthesized, their structures were confirmed using 1H and 13C NMR, COSY, and ESI-MS. As well, their electrochemical, photoelectrochemical, and photovoltaic properties were studied. These complexes were successfully implemented in the assembly of DSSCs for which global conversion efficiencies were obtained. Photovoltaic results demonstrated that the perylene moieties of these dyes were responsible for the photoactivated electron injection into the TiO2 collectors. As well, the <tpyRuIItpy> complexes were simultaneously deactivated by the presence of the perylene radical cations newly formed by photoactivation, thus inhibiting charge injection into the TiO2.
EXPERIMENTAL
Starting materials were commercially purchased and used without further purification. Thin layer chromatography (TLC) was conducted on flexible sheets (Baker-flex) percolated with Al2O3 (IB-F) or SiO2 (IB2-F) and visualized by UV light. Column chromatography was conducted using basic Al2O3, Brockman Activity I (60-325 mesh) or SiO2 (60-200 mesh) from Fisher Scientific. Melting points were determined on an Electrothermal 9100 heater. 1H and 13C NMR spectra were recorded on either a Varian Mercury 300 or a Varian NMRS 500 spectrometer. Mass spectra were obtained on a Synapt HDMS quadrupole/time-of flight (Q/TOF) mass spectrometer (Waters Corp., Milford, MA); the sprayed solution was prepared by dissolving 1 mg of sample in 1 mL of a MeCN/MeOH (1:1) solvent mixture.
Preparation of the 8-, 9-, 13-, and 14-Sensitized Solar Cells. Nanoparticulate TiO2 electrodes (523-roughness factor, η, and 5 µm thick, d, estimated according to measurements of the electroactive area, Ae, and perfilometry, respectively) were obtained by electrophoretic deposition of TiO2 nanoparticles (P25 Degussa, 21 nm diameter), as previously reported. Cathodes were obtained, as follows: 8 µL/cm2 of a 10 mM H2PtCl6⋅6H2O (Strem Chemicals, 99.9%-Pt) solution in Me2CHOH (J.T. Baker, 99.97%) was homogeneously spread on the surface of optically transparent electrodes (OTE) based on indium-doped tin oxide (TEC15, Hartford Glass Co., USA). The cathodes were subsequently dried at 25 °C and finally annealed in air at 380 °C for 30 min. TiO2 electrodes were dyed by immersion in 0.40 mM solutions of (a) 8 and 9 both in MeOH (J. T. Baker, HPLC grade), (b) 14 in MeOH/CHCl3 (3:1) or (c) 13 in MeOH/CHCl3 (5:1), at 25 °C for 6 days. DSSCs were assembled in a sandwich arrangement using a 160 µm-thick o-ring (6 mm i.d.), home-made from a commercial transparency sheet (CG3700 3M, typically used as projecting transparencies) in order to avoid premature short circuit. A solution of 0.3 M LiI (Aldrich, 99.9%) + 0.015 M I2 (J.T. Baker, ACS reagent) in propylene carbonate (Aldrich, 99.7%) was employed as the electrolytic medium in the photovoltaic cells. An effective geometric area of 0.28 cm2 was illuminated for all the photoelectrochemical experiments.
Electrochemistry. Cyclic voltammetry (CV) experiments were performed at 25 °C and 25 mV/s by means of a BAS Epsilon potentiostat-galvanostat. A glassy carbon working-disc-electrode (1.5 mm-diameter) was immersed in a typical three-electrode cell containing DMF (99.8 %, J.T. Baker) + 0.1M Bu4NPF6 (Fluka, 98%) for testing complexes 8 and 9. Electrolytic mixtures of MeOH/CHCl3 (5:1) + 0.1M Bu4NPF6 and MeOH/CHCl3 (3:1) + 0.1M Bu4NPF6 were used for obtaining the CV responses for samples 13 and 14, respectively. Experimental setup was completed when silver and platinum wires were inserted in the electrochemical cell as pseudo- and counter-electrodes, respectively. All solutions were purged with ultra-pure nitrogen (Praxair, 99.999%) for 15 min. prior to conducting the experiments. Potentials were referenced to the ferrocinium/ferrocene redox scale (denoted as Fc+ | Fc). PDIs are stable, electron-deficient molecules, which are difficult to oxidize and easy to reduce. Scheme SI-A shows the one electron-reversible oxidation (as revealed by CV) and Scheme S1-B shows the reduction process reported for PDI.31
Photoelectrochemistry. Discharge curves were obtained for the DSSCs utilizing a variable resistance of 52 kΩ upon polychromatic illumination emitted by a MR16 50W 12V lamp (GE) equipped with an EXN dichroic reflector to diffuse the light. Power of the light source was 9.21 mW/cm2 (ca. 0.1 Sun). Steady-state photocurrent spectra were obtained upon monochromatic illumination (400 - 650 nm) using a 6257 100W Xe lamp coupled to a 77250 monochromator, both purchased from Thermo-Oriel and calibrated by means of a 17043 Eppley Thermopile. Incident-photon-to-current efficiencies (IPCE) were estimated according to Eq. 1, where λ, jph,λ and I0,λ denote incident photon wavelength (in nm) photocurrent density (in µA/cm2), and incident photon flux (in W/cm2), respectively. The constant 0.82 corresponds to the effective transmittance of the incident photon flux through the OTE. All these experiments were conducted on an IM6 BAS-Zahner potentiostat-galvanostat in order to measure open-circuit-potentials or steady-state-photocurrents.
General procedure for the synthesis of terpyridine-perylene ligands 3 and 4. A mixture of 1,6,7,12-tetra(p-t-butylphenoxy)perylene-3,4:9,10-tetracarboxylic acid bis(anhydride) 1 (500 mg, 0.5 mmol), 4- or 3-aminophenylterpyridine 2 or 4 (1.31 g, 4.06 mmol) in quinoline (50 mL) was stirred at 220 ºC under argon for 3 days. The mixture was cooled to 25 ºC, precipitated with AcOH (50 mL), filtered, and washed with water, and then MeOH. The product was column chromatographed (Al2O3) eluting with a mixture of CHCl3/hexane (2:1).
N,N'-Bis[4-([2,2':6',2"]terpyridin-4'-yl)phenyl]-1,6,7,12-tetra(p-t-butylphenol)perylene-3,4:9,10-tetracarboxylic acid bisanhydride (3): red powder, 51%; mp > 350 ºC; 1H NMR (300 MHz, CDCl3) δ 8.79 (s, 4H, 3',5'-tpyH), 8.74 (d, 4H, J = 4.2 Hz, 6,6"-tpyH), 8.68 (d, 4H, J = 7.8 Hz, 3,3"-tpyH), 8.30 (s, 4H, perH), 8.06 (d, 4H, J = 8.4 Hz, PhH), 7.89 (dd, 4H, J1 = J2 = 7.8 Hz, 4,4"-tpyH), 7.43 (d, 4H, J = 8.4 Hz, PhH), 7.36 (dd, J1 = 7.8 Hz, J2 = 4.2 Hz 4H, 5,5"-tpyH), 7.25 (d, 8H, J = 9.0 Hz, ArH), 6.88 (d, 8H, J = 9.0 Hz, ArH), 1.27 (s, 36 H, CH3); 13C NMR δ 163.75, 156.40, 156.28, 153.07, 149.68, 149.37, 147.70, 139.19, 137.09, 136.21, 133.41, 129.44, 128.46, 126.93, 124.06, 122.79, 121.61, 121.08, 120.51, 120.05, 119.60, 119.27, 105.23, 34.59, 31.65; ESI-MS: m/z 1597.38 (calcd. [M+H]+ = 1597.64).
N,N'-Bis[3-([2,2':6',2"]terpyridin-4'-yl)phenyl]-1,6,7,12-tetra(p-t-butylphenol)perylene-3,4:9,10-tetracarboxylic acid bisanhydride (5): red powder, 60%; mp > 350 ºC; 1H NMR (500 MHz, CDCl3) δ 8.76 (s, 4H, 3',5'-tpyH), 8.70 (d, 4H, J = 4.7 Hz, 6,6"-tpyH), 8.66 (d, 4H, J = 7.8 Hz, 3,3"-tpyH), 8.3 (s, 4H, perH), 8.02 (d, 2H, J = 7.1 Hz, PhH), 7.86 (dd, 4H, J1 = J2 = 7.8 Hz, 4,4"-tpyH), 7.83 (s, 2H, PhH), 7.67 (dd, 2H, J1 = J2 = 7.1 Hz, PhH), 7.37 (d, 2H, J = 7.1 Hz, PhH), 7.33 (dd, J1 = 7.8 Hz, J2 = 4.7 Hz, 4H, 5,5"-tpyH), 7.25 (d, 8H, J = 9.1 Hz, ArH), 6.89 (d, 8H, J = 9.1 Hz, ArH), 1.27 (s, 36 H, CH3); 13C NMR δ 163.54, 156.16, 156.03, 152.90, 149.21, 149.11, 147.39, 139.92, 136.77, 136.05, 133.18, 129.85, 129.18, 126.67, 123.78, 122.56, 121.30, 119.37, 118.91, 105.02, 34.35, 31.42; ESI-MS: m/z 1596.71 (calcd. [M]+ = 1596.64).
[Ru(6)Cl3] (7). 4'-(4-Methylphenyl)[2,2':6',2"]terpyridine (6; 200 mg, 890 µmol) was treated with RuCl3·3H2O (251.7 mg, 1.21 mmol) in EtOH (20 mL), then the suspension was refluxed for 12 h. After the mixture was cooled, the resultant dark brown solid was filtered, washed with cold EtOH, and dried in vacuo to give 7: 290 mg (88%). This material was used without further purification.
General procedure for the synthesis of complexes 8, 9, and 13: Bis(terpyridine) ligand (3, 5 or 12) (40 mg, 31 µmol) was added to a suspension of RuIIItpy 7 (25 mg, 69 µmol) in a mixed solvent (20 mL) of EtOH/CHCl3 (2:1), then N-ethylmorpholine (500 µL) was added. The mixture was refluxed for 12 h. After cooling, the resulting deep red solution was filtered (Celite), solvent was removed in vacuo and the residue was column chromatographed (SiO2), eluting with H2O: KNO3:MeCN (1:1:35). The counter ion was exchanged by adding NH4PF6, and then the product was filtered, washed with water and dried in vacuo.
[(3)Ru2(6)2][PF6]4 (8): red powder, 50 mg (78%), mp > 350 °C; 1H NMR (500 MHz, CD3OD) δ: 9.53 (s, 4H, 3',5'-tpyH), 9.45 (s, 4H, 3',5'-tpyH), 9.14 (m, 8H, 3,3"-tpyH), 8.71 (d, 4H, J = 8.0 Hz, PhH), 8.67 (s, 4H, perH), 8.45 (d, 4H, J = 8.0 Hz, PhH), 8.36 (m, 8H, 4,4"-tpyH), 8.02 (d, 4H, J = 8.0 Hz, PhH), 7.90 (d, 4H, J = 8.0 Hz, PhH), 7.80 (m, 8H, 6,6"-tpyH), 7.65 (m, 16H, ArH, 5,5"-tpyH), 7.31 (d, 8H, J = 8.0 Hz, ArH), 2.90 (s, 6H, CH3), 1.66 (s, 36H, CH3); 13C NMR δ: 163.56, 157.86, 157.80, 155.01, 151.63, 149.36, 147.99, 147.45, 141.14, 138.30, 138.25, 137.17, 136.80, 133.14, 133.03, 130.19, 129.88, 127.82, 127.70, 126.46, 124.72, 124.63, 121.54, 121.04, 121.01, 120.99, 120.08, 120.05, 119.69, 119.15, 34.04, 30.92, 29.31; ESI-MS (m/z): 2881.1 [M - PF6ˉ]1+ (Calcd. m/z = 2881.5), 863.5 [M - 3PF6ˉ)]3+ (Calcd. m/z = 863.6), 611.4 [M - 4PF6ˉ)]4+ (calcd. m/z = 611.5).
[(5)Ru2(6)2][PF6]4 (9): red powder, 50 mg (78%), mp > 350 °C; 1H NMR (500 MHz, CD3CN) δ 9.04 (s, 4H, 3',5'-tpyH), 9.02 (s, 4H, 3',5'-tpyH), 8.66 (d, 4H, J = 8.0 Hz, 3,3"-tpyH), 8.63 (d, 4H, J = 8.0 Hz, 3,3"-tpyH), 8.32 (d, 2H, J = 7.5 Hz, PhH), 8.18 (s, 4H, perH), 8.14 (m, 8H, PhH), 7.92 (m, 8H, 4,4"-tpyH), 7.64 (d, 2H, J = 7.5 Hz, PhH), 7.58 (d, 4H, J = 8.0 Hz, PhH), 7.41 (m, 8H, 6,6"-tpyH), 7.36 (d, 8H, J = 7.5 Hz, ArH), 7.16 (m, 8H, 5,5"-tpyH), 6.96 (d, 8H, J = 7.5 Hz, ArH), 2.57 (s, 6H, CH3), 1.32 (s, 36H, CH3); 13C NMR δ: 163.50, 157.80, 157.73, 156.05, 155.26, 154.95, 152.61, 151.57, 149.29, 147.38, 141.08, 138.18, 137.10 136.73, 133.07, 132.6, 130.13, 129.81, 128.42, 127.75, 127.67, 127.19, 126.42, 124.57, 122.12, 12.47, 120.97, 120.92, 120.01, 119.62, 119.08, 33.97, 30.93, 29.24; MALDI-TOF-MS (m/z): 2881.0 [M - PF6ˉ]1+ (calcd. m/z = 2881.2).
1,6/1,7-Dibromo-3,4,9,10-perylenetetracarboxylic dianhydride.31 A mixture of 3,4,9,10-perylene- tetracarboxylic dianhydride (4 g, 10 mmol) and H2SO4 (60 mL) was stirred at 55 ºC for 18 h. Next I2 (80 mg, 300 µmol) was added and the mixture was stirred for 5 h at 55 ºC. Br2 (3.5 g, 20 mmol) was added, the reaction mixture was stirred for 48 h at 85 ºC, followed by cooling to 0 ºC. Excess Br2 was removed using air stream in the hood. The product was precipitated with cold water (30 mL), filtered, washed with water, EtOH and then dried in vacuo to afford (89%) an orange powder: 5 g. The product was used in the next step without further purification.
N,N'-Bis(n-octyl)-1,6/1,7-dibromo-3,4,9,10-perylenetetracarboxydiimide32,33 (10). To a mixture of 1,6/1,7-dibromo-3,4,9,10-perylenetetracarboxylic dianhydride (2.5 g, 4.5 mmol) and n-octylamine (5.8 g, 44.8 mmol), was added a mixture of 2-BuOH:H2O (80 mL, 1:1). The reaction mixture was stirred under argon at 65 ºC for 12 h, cooled to 25 ºC, precipitated with MeOH (150 mL), and filtered. The product was dried in vacuo and column chromatographed (SiO2) eluting with CHCl3 to give 10, as an orange powder: 5 g (89 %); 1H NMR (500 MHz, CDCl3) δ 9.43 (d, 4H, J = 8.0 Hz, perH ), 8.87 (s, 4H, perH), 8.65 (d, 4H, J = 8.0 Hz, perH), 4.20 (m, 8H, CH2), 1.75 (m, 8H, CH2), 1.44-1.26 (m, 48H, CH2), 0.89 (t, 12H, CH3); 13C NMR δ 163.39, 163.01, 162.51, 162.14, 138.27, 138.15, 133.32, 133.08, 132.93, 132.52, 130.16, 130.02, 129.36, 128.64, 128.27, 127.88, 127.08, 123.54, 123.35, 122.92, 122.61, 121.79, 120.97, 41.16, 41.04, 40.93, 32.04, 29.54, 29.43, 28.30, 27.38, 27.33, 27.29, 22.86, 14.31; MALDI-TOF-MS: m/z 770.20 (calcd. M+ = 770.13).
N,N'-Bis(n-octyl)-1,7-di[4-([2,2':6',2"]terpyridin-4'-yl)phenyl]-3,4,9,10-perylenetetracarboxydiimide(12). To a mixture of 10 (250 mg, 500 μmol) and 4'-(4-boronatophenyl)[2,2':6',2'']terpyridine 11 (485 mg, 1.3 mmol) in toluene: t-BuOH:H2O (2:1:1; 80 mL), was added K2CO3 (3.5 g, 27.4 mmol). The system was freeze-pump-thawed three times and backfilled with argon; then Pd(PPh3)2Cl2 (16 mg) was added. After refluxing for 72 h under argon, the mixture was cooled to 25 °C, solvent was removed in vacuo and the residue was dissolved in CHCl3 and filtered. After concentration in vacuo, the residue was purified by column chromatography (Al2O3) eluting with CHCl3, followed by recrystallization from CHCl3/MeOH (5x) to give (31%) 15, as a red solid: mp > 300 °C; 1H NMR (500 MHz, CDCl3) δ 8.88 (s, 4H, 3',5'-tpyH), 8.79 (d, 4H, J = 4.5 Hz, 6,6"-tpyH), 8.74 (d, 4H, J = 8.0 Hz, 3,3"-tpyH), 8.69 (s, 2H, perH), 8.23 (d, 2H, J = 8.0 Hz, perH) , 8.11 (d, 4H, J = 8.0 Hz, PhH), 7.95 (m, 6H, perH, 4,4"-tpyH), 7.76 (d, 4H, J = 8.0 Hz, PhH), 7.42 (dd, J1 = 8.0 Hz, J2 = 4.5 Hz, 4H, 5,5"-tpyH), 4.20 (t, 4H, J = 8 Hz, CH2), 1.43-1.27 (m, 23H, CH2), 0.87 (m, 6H, CH3); 13C NMR δ 163.55, 163.42, 140.44, 135.30, 134.90, 133.36, 132.88, 130.60, 130.12, 129.86, 129.46, 129.42, 127.98, 124.71, 122.66, 122.34, 40.81, 31.99, 29.51, 29.36, 28.32, 27.29, 22.79, 14.23; ESI-MS: m/z 1229.64 (calcd. [M+H]+ = 1229.54).
[(10)Ru2(6)2][PF6]4 (13): red powder, (78%), mp > 350 °C; 1H NMR (500 MHz, CD3OD): δ 9.35 (s, 4H, 3',5'-tpyH), 9.18 (s, 4H, 3',5'-tpyH), 8.91 (d, 4H, J = 8.0 Hz, 3,3"-tpyH), 8.83 (d, 4H, J = 8.0 Hz, 3,3"-tpyH), 8.69 (s, 2H, perH), 8.49 (d, 4H, J = 8.0 Hz, PhH), 8.26 (d, 2H, J = 8.0 Hz, perH), 8.13 (d, 4H, J = 8.0 Hz, PhH), 8.02-7.96 (m, 14H, PhH, perH, 4,4"-tpyH), 7.54 (m, 8H, 6,6"-tpyH), 7.51 (d, 4H, J = 8.0 Hz, PhH), 7.30 (m, 8H, 5,5"-tpyH), 4.23 (t, 4H, CH2), 2.54 (s, 6H, CH3), 1.77 (m, 8H, CH2), 1.46-1.25 (m, 23H, CH2), 0.86 (m, 6H, CH3); 13C NMR δ 162.72, 157.84, 157.75, 155.14, 155.12, 154.84, 151.62, 151.35, 148.88, 140.62, 137.94, 137.87, 137.78, 137.77, 137.74, 133.09, 127.42, 127.36, 126.75, 126.68, 124.64, 124.31, 121.37, 121.35, 120.76, 31.24, 28.71, 28.60, 28.54, 27.44, 26.53, 21.96, 20.18, 13.03; ESI-MS (m/z): 519.70 [M-4PF6ˉ]4+ (calcd. m/z = 519.65), 741.20 [M-3PF6ˉ]3+ (calcd. m/z = 741.19).
ACKNOWLEDGEMENTS
The authors gratefully thank the National Science Foundation (GRN CHE-1151991; CW CHE-1308307) and the Ohio Board of Regents. The Mexican Council for Science and Technology is also gratefully acknowledged for its financial support (CONACyT; 106000). P. F. M. gratefully acknowledges CONACyT for her Ph. D. scholarship (104180).
References
1. A. Herrmann and K. Müllen, Chem. Lett., 2006, 35, 978. CrossRef
2. C. Huang, S. Barlow, and S. R. Marder, J. Org. Chem., 2011, 76, 2386. CrossRef
3. H. Langhals and T. Pust, Eur. J. Org. Chem., 2010, 3140. CrossRef
4. F. Schlosser, V. Stepanenko, and F. Würthner, Chem. Commun., 2010, 46, 8350. CrossRef
5. M. R. Wasielewski, J. Org. Chem., 2006, 71, 5051. CrossRef
6. A. Herrmann, T. Weil, V. Sinigersky, U.-M. Wiesler, T. Vosch, J. Hofkens, F. C. De Schryver, and K. Müllen, Chem. Eur. J., 2001, 7, 4844. CrossRef
7. C. Li and H. Wonneberger, Adv. Mater., 2012, 24, 613. CrossRef
8. F. Würthner and K. Meerholz, Chem. Eur. J., 2010, 16, 9366. CrossRef
9. D. Görl, X. Zhang, and F. Würthner, Angew. Chem. Int. Ed., 2012, 51, 6328. CrossRef
10. Y. Tidhar, H. Weissmen, S. G. Wolf, A. Gulino, and B. Rybtchinski, Chem. Eur. J., 2011, 17, 6068. CrossRef
11. V. Stepanenko and F. Würthner, Small, 2008, 4, 2158. CrossRef
12. U. S. Schubert, H. Hofmeier, and G. R. Newkome, Modern Terpyridine Chemistry; Wiley-VCH: Weinheim, 2006. CrossRef
13. U. S. Schubert, A. Winter, and G. R. Newkome, Terpyridine-Based Materials - For Catalytic, Optoelectronic, and Life Science Applications; Wiley-VCH: Weinheim, 2011. CrossRef
14. T. J. Cho, C. D. Shreiner, S.-H. Hwang, C. N. Moorefield, B. Courneya, L. A. Godínez, J. Manríquez, K.-U. Jeong, S. Z. D. Cheng, and G. R. Newkome, Chem. Commun., 2007, 4456. CrossRef
15. C. Houarner, E. Blart, P. Buvat, and F. Odobel, Photochem. Photobiol. Sci., 2005, 4, 200. CrossRef
16. S. You, Q. Cai, K. Müllen, W. Yang, and M. Yin, Chem. Commun., 2014, 50, 823. CrossRef
17. T. Heek, F. Würthner, and R. Haag, Chem. Eur. J., 2013, 19, 10911. CrossRef
18. J. Zhou, J. Zhang, Y. Lai, Z. Zhou, Y. Zhao, H. Wang, and Z. Wang, New J. Chem., 2013, 37, 2983. CrossRef
19. S. K. Yang and S. C. Zimmerman, Adv. Funct. Mater., 2012, 22, 3023. CrossRef
20. V. Percec, S. D. Hudson, M. Peterca, P. Leowanawat, E. Aqad, R. Graf, H. W. Spiess, X. Zeng, G. Ungar, and P. A. Heiney, J. Am. Chem. Soc., 2011, 133, 18479. CrossRef
21. M. Franceschin, C. Bombelli, S. Borioni, G. Bozzuto, S. Eleuteri, G. Mancini, A. Molinari, and A. Bianco, New J. Chem., 2013, 37, 2166. CrossRef
22. W. Goodall, K. Wild, K. J. Arm, and J. A. G. Williams, J. Chem. Soc., Perkin Trans. 2, 2002, 1669. CrossRef
23. R.-A. Fallahpour, Eur. J. Inorg. Chem., 1998, 1205. CrossRef
24. G. R. Newkome, T. J. Cho, C. N. Moorefield, R. Cush, P. S. Russo, L. A. Godínez, M. J. Saunders, and P. Mohapatra, Chem. Eur. J., 2002, 8, 2946. CrossRef
25. C. Li, Z. Liu, J. Schoneboom, F. Eickemeyer, N. G. Pschirer, P. Erk, A. Herrmann, and K. Müllen, J. Mater. Chem., 2009, 19, 5405. CrossRef
26. F. Fabregat-Santiago, J. Bisquert, G. Garcia-Belmonte, G. Boschloo, and A. Hagfeldt, Sol. Engery Mater. Sol. Cells, 2005, 87, 117. CrossRef
27. S. K. Lee, Y. Zu, A. Herrmann, Y. Geerts, K. Müllen, and A. J. Bard, J. Am. Chem. Soc., 1999, 121, 3513. CrossRef
28. R. Dobrawa and F. Würthner, Chem. Commun., 2002, 1878.
29. J. R. Durrant, S. A. Haque, and E. Palomares, Chem. Commun., 2006, 3279. CrossRef
30. Y. Ie, T. Uto, A. Saeki, S. Seki, S. Tagawa, and Y. Aso, Synth. Met., 2009, 159, 797. CrossRef
31. Q. Zhao, S. Zhang, Y. Liu, J. Mei, S. Chen, P. Lu, A. Qin, Y. Ma, J. Z. Sun, and B. Z. Tang, J. Mater. Chem., 2012, 22, 7387. CrossRef
32. L. Fan, Y. Xu, and H. Tian, Tetrahedron Lett., 2005, 46, 4443. CrossRef
33. M. Queste, C. Cadiou, B. Pagoaga, L. Giraudet, and N. Hoffmann, New J. Chem., 2010, 34, 2537. CrossRef