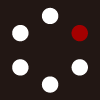
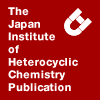
HETEROCYCLES
An International Journal for Reviews and Communications in Heterocyclic ChemistryWeb Edition ISSN: 1881-0942
Published online by The Japan Institute of Heterocyclic Chemistry
e-Journal
Full Text HTML
Received, 31st March, 2014, Accepted, 22nd April, 2014, Published online, 25th April, 2014.
DOI: 10.3987/COM-14-12994
■ Synthetic Access to Poly-Substituted 11H-Pyrido[3,2-a]carbazoles, a DNA-Intercalating Ellipticine Related Structure, and Their Antiproliferative Activity
Ming-Yu Wu, Elkhabiry Shaban, Marta Świtalska, Ning Wang, Miho Shimoda, Yusuke Mizutani, Megumi Yoshida, Zhen-Wu Mei, Hiroyuki Kawafuchi, Junzo Nokami, Joanna Wietrzyk,* Xiao-Qi Yu, and Tsutomu Inokuchi*
Division of Chemsitry and Biochemistry, Graduate School of Natural Science and Technology, Okayama University, 3-1-1 Tsushima-naka, Okayama 700-8530, Japan
Abstract
The facile procedure for the synthesis of the 11H-pyrido[3,2-a]carbazole structure involving the Fischer indole cyclization on tetrahydroquinolinones, available from enaminones and methyl 2-formyl-3-oxopropanoate, followed by the aromatization of the resulting 5,6-dihydro derivatives is described. This method allows for the introduction of substituents at C2, C6, and C8 to the scaffold by choice of the starting materials. In the biological testing, introduction of the phenyl group at C6 is significantly effective to improve the antiproliferative activity.INTRODUCTION
The natural plant alkaloids with a linearly condensed aromatic ring system show interesting antitumor activities due to their DNA intercalating ability.1 For example, ellipticine (5,11-dimethyl-6H-pyrido[4,3-b]carbazole, I, Figure 1), an alkaloid isolated from Apocyanaceae plants,2,3 exhibits significant antitumor, anti-HIV,4a and anti-malarial activities.4c Accordingly, the overlapping interaction of the 6H-pyridocarbazole aromatic rings with that of a DNA base pair was precisely demonstrated.5 Nevertheless, ellipticine I and its derivatives are mutagenic to the Salmonella typhimurium Ames tester strains, Neurospora crassa, and mammalian cells.4a,4b,6
The DNA intercalating and antitumor ability of the related structures, such as 11H-pyrido[3,2-a]-, [4,3-a]-, [3,4-a]-, and [2,3-a]-carbazoles III~VI were subsequently tested, indicating that the cytotoxicity of the 11H-pyridocarbazoles, measured on L1210 cells in vitro, is much lower than those of the 6H- and 7H-pyridocarbazole analogues I, II.7 However, a comparative study of the interaction of the pyridocarbazole analogues with DNA topoisomerase II has not been well examined. Furthermore, the 3-substituted 11H-pyrido[3,2-a]carbazole derivatives were shown to possess slight antidepressant and L-dopa-potentiating effects.8
Syntheses of ellipticine and its related pyridocarbazole analogues were mainly relied on cyclization of hydrazinoquinoline with cyclohexanone via the Fischer-Borsche indole synthesis followed by aromatization or the Skraup reaction of aminocarbazoles with acrolein.9,10 A Doebner reaction was applied to form 1-carboxy-3,5-dimethyl-7H-pyrido[2,3-c]carbazole from 3-amino-2-methylcarbazole, acetaldehyde, and pyruvic acid.11 The C ring of ellipticine core are constructd by the cyclization of o-quinodimethane intermediate, generated by thermolysis of the 2-alkyl-3-[α-(3-pyridyl)vinyl]indole.12,13 Regioselective acylation of a 3-lithio-l-(phenylsulfonyl)indole with 3,4-pyridinedicarboxylic anhydride to the corresponding quinone was demonstrated.14 A one-step procedure was developed by the Diels-Alder reaction between 1,4-dimethylpyrano[3,4-b]indol-3-one and 3,4-didehydropyridine to construct ellipticine core.15
For the synthesis of the 11H-pyridocarbazoles core, the Fischer indole cyclization of the 7-(azanaphthalenyl)hydrazines with 4-methoxycyclohexanone followed by aromatization,16–19 or the reaction of 7,8-dihydroquinoline-2,5(1H,6H)-dione with phenylhydrazine followed by aromatization,8 or the electrocyclization of the 1-(3-indolyl-2-(pyridyl)propenes under UV irradiation have been reported.20,21 However, these procedures suffer from low yields during the aromatization steps and are not feasible to supply the corresponding pyridocarbazoles with various substituents in the further structure–activity relationship (SAR) studies.
In this study, we developed a synthetic access to the 2-methoxycarbonyl-11H-pyrido[3,2-a]carbazoles 1 by the Fischer indole synthesis of the corresponding 2-methoxycarbonyltetrahydroquinolinones 2 with phenylhydrazines 3.22–24 The method described here would permit formation of 11H-pyrido[3,2-a]carbazole scaffold with an ester group at the C2 and additional option of substituents at the C6 and C8. Availability of poly-substituents in the pyrido[3,2-a]carbazole core could be useful for further SAR study toward prospective anticancer and antimalarial activities (Figure 2). In addition, preliminary biological testing of the some obtained compounds was performed against MV4-11 cell line (human leukemia).
RESULTS AND DISCUSSIONS
Synthesis of tetrahydroquinolinone derivatives and their biological properties as group I mGluR antagonists are claimed in the patent by Jirgensons et al.25 However, we prepared the starting 2-methoxycarbonyltetrahydroquinolinones 2 by condensation of the enaminones 5 with methyl 2-formyl-3-oxopropanoate (7), according to the method reported by us.26
Thus, 5 were formed by the reaction of the 5-substituted cyclohexane-1,3-diones 4 with ammonium acetate while heating in toluene.27,28 Their counterpart 7 was prepared by the Claisen condensation of methyl 3,3-dimethoxypropionate 6 with methyl formate using NaH in ether followed by hydrolysis with 10% HCl. The two-component [3+3] cycloannulation process for the rapid formation of the desired tetrahydroquinolinones 2 was achieved in an one-pot operation by the tosylation of 7 with TsCl-Et3N forming the corresponding 2-formyl-3-(tosyloxy)acrylate, followed by the addition of 5 in the presence of pyridine, affording 2-methoxycarbonyltetrahydroquinolones 2 in moderate yields (Scheme 1).
The Fischer indole cyclization of 2a (R1 = H) using phenylhydrazine 3a (R2 = H) was performed by heating in acetic acid with 36% HCl, affording the desired tetracyclic 6,11-dihydro-5H-pyrido[3,2-a]carbazoles 8a in 72% yield. A similar indole synthesis on 2a (R1 = H) was successfully achieved with 4-BrC6H4NHNH2 3b (R2 = Br), while the use of 4-NO2C6H4NHNH2 3d (R2 = NO2) resulted in no desired indole structure. The oxidative dehydrogenation of 8a to the desired 11H-pyrido[3,2-a]carbazole 1a was achieved by the treatment with DDQ in benzene in 74% yield. The substituent R1 affected the conversion of the DDQ oxidation; a high yield was attained with Ph group (1d, 98% yield), while a slightly lesser yield with Me group (1c, 85%) (Scheme 2).
We subsequently examined the preparation of 5,6-dimethyl-11H-pyrido[3,2-a]carbazole 11 through the 1,2-rearrangement of the gem-dimethyl group of the precursor 10 during the aromatization step.29–31 Thus, the tetrahydroquinolinone 9, derived from the dimedone in a manner described in the literature,26 was converted to the corresponding 6,6-dimethyl-6,11-dihydro-5H-pyrido[3,2-a]carbazole 10 by the Fischer indole cyclization with hydrazine 3a. The oxidation of 10 with SeO2 (2 equiv.) in MeCN-H2O afforded the desired 11 (36%) and the ketone 12 (54%) as a by-product. In all our attempts, the oxidation of 10 with DDQ, the reaction was sluggish and a hardly separable mixture of the starting 10 and the aromatized 11 was produced. The formation of 11 can be explained by the Wagner-Meerwein type rearrangement of the methyl group followed by aromatization during the oxidation process with SeO2 (Scheme 3).
In connection of search for finding biologically significant derivatives, the 11H-pyrido[3,2-a]carbazoles 1a was converted its pyridinium salt 13 by treatment with MeI in DMF on heating, quantitatively (Scheme 4).
Antiproliferative activity in vitro against MV4-11 cell line
In order to study the biological properties of 11H-pyrido[3,2-a]carbazoles, we tested antiproliferative activity of prepared compounds against human leukemia MV4-11 cells and the results are summarized in Table 1. The results of the cytotoxic activity in vitro were expressed as IC50 (in μM), the concentration of the compound that inhibits proliferation of the cells by 50% as compared to the untreated control cells. Except for 1d and 12, other compounds show no-activity against MV4-11 cells. We modified structure of 1a by introducing Br group at C8 and Me group at C6, but those groups are not effective. Introduction of the Ph group at C6 significantly improve the antiproliferative activity. The presence of two Me groups at C5 and C6 is also not effective. Some literatures reported that introduction Me group at N atom of the indole or quinoline ring can improve the biological activity of the compound,32,34 but the compound 13, pyridinium bearing the methyl group at the N4 of the quinoline ring, still showed no activity. However, 5-ketone compound 12 showed antiproliferative activity. According to SAR study, introduction of an amino group is a promising method to improve the biological activity,32,34 so in the future, we will modify the 11H-pyrido[3,2-a]carbazole core with the amino group and varying other substituents at C8 or C6.
In summary, we have described a quick access to poly-substituted 11H-pyrido[3,2-a]carbazoles 1 by the Fischer indole cyclization on 5-oxo-5,6,7,8-tetrahydroquinoline-3-carboxylate followed by aromatization. Due to high activity of the saturated C5–C6 bond, flanked by two aromatic rings of the resulting 6,11-dihydro-5H-pyrido[3,2-a]carbazole-2-carboxylate 8, the subsequent DDQ oxidation smoothly proceeded to afford the desired 1 in good yields. When this aromatization was applied to the 6,6-dimethyl derivative 10, the 1,2-rearrangement of a methyl group was induced with SeO2 to produce the corresponding 5,6-dimethylated 11H-pyrido[3,2-a]carbazole 11. Thus, we developed a new procedure to introduce an alkyl group to the C5 position. Introduction of the phenyl group at C6 is significantly effective to improve the antiproliferative activity. Further study of the derivatives available from the 11H-pyrido[3,2-a]carbazole motif in order to improve their anticancer activities is currently underway in our laboratory.
EXPERIMENTAL
General Procedures
The commercially obtained reagents were directly used without further purification. Reactions were monitored by thin layer chromatography (TLC) on silica gel plates (60 F254) and flash chromatography was performed on silica gel (230–40 mesh) using a gradient solvent system. (hexane/ ethyl acetate as the eluent unless otherwise specified). The 1H NMR and 13C NMR spectra were measured on the Varian INOVA-400 spectrometer. Melting points were determined on a J-Science RFS-10 hot stage microscope. High resolution mass spectra were obtained on a Bruker micrOTOF II-SKA spectrometer.
Preparation of methyl 6,11-dihydro-5H-pyrido[3,2-a]carbazole-2-carboxylate (8a), a general procedure (Method A for 8a and 8b): A mixture of 2a (205 mg, 1.0 mmol) and phenylhydrazine (3a, 130 mg, 1.2 mmol) in AcOH (4 mL)-36% HCl (1 mL) was heated under reflux for 5 h. After the reaction completed, a small amount of ice water was added, then the mixture was neutralized with saturated aqueous NaHCO3. Products were extracted with EtOAc (30 mL x 3), and the extracts were washed with brine, dried over Na2SO4, and concentrated on an evaporator. The crude product was used for the next step without further purification.
Fischer indole cyclization and the subsequent esterification, giving 8c, 8d, and 10 (Method B): A mixture of 2b (1.0 mmol) and 3a (1.2 mmol) were dissolved in AcOH-HCl (4:1, 5 mL) and heated for 5 h under reflux. After the reaction completed, the yellow solids were collected by filtration. The solids thus obtained were dissolved in MeOH (10 mL) and SOCl2 (238 mg, 2 mmol) was added. The mixture was stirred under reflux for 20 h. The solvents were evaporated under reduced pressure, a small amount of water was added, and neutralize to pH 8 with 1N NaOH. The solids 7c obtained by filtration was dried in vacuum overnight.
Methyl 6,11-dihydro-5H-pyrido[3,2-a]carbazole-2-carboxylate (8a): Pale yellowish solids in 72% yield, mp 239–241 °C (after LC); IR (KBr) νmax = 3225, 2947, 2889, 2839, 1937, 1898, 1821, 1728, 1601, 1551, 1481, 1441, 1440, 1362, 1317, 1294, 1244, 1119, 1051, 1009, 993, 978, 922, 910, 870, 810, 760, 748 cm-1; 1H NMR (400 MHz, CDCl3) δ 8.93 (s, 1H), 8.39 (s, 1H), 8.16 (s, 1H), 7.58 (d, J = 8.0 Hz, 1H), 7.41 (d, J = 8.4 Hz, 1H), 7.25 (t, J = 7.2 Hz, 1H), 7.16 (t, J = 7.2 Hz, 1H,), 3.98 (s, 3H), 3.34 (t, J = 8.0 Hz, 2H), 3.15(t, J = 8.0 Hz, 2H); 13C NMR (100 MHz, DMSO-d6) δ 165.9, 161.9, 147.1, 138.3, 130.9, 127.6, 126.6, 125.3, 124.5, 123.0, 119.8, 119.4, 112.5, 112.1, 52.8, 32.4, 18.9. HRMS (ESI) calcd for C17H15N2O2 (M+H)+: 279.1134, found: 279.1128.
Methyl 8-bromo-6,11-dihydro-5H-pyrido[3,2-a]carbazole-2-carboxylate (8b): Pale yellowish solids in 74% yield, mp 293–295 °C (after LC); IR (KBr) νmax = 3229, 2953, 2841, 1886, 1822, 1726, 1616, 1601, 1550, 1487, 1443, 1397, 1360, 1312, 1296, 1248, 1220, 1128, 1041, 1013, 990, 972, 934, 968, 812, 764 cm-1; 1H NMR (400 MHz, DMSO-d6) δ 12.00 (br s, 1H), 8.79 (s, 1H), 8.48 (s, 1H), 7.72 (s, 1H), 7.35 (d, J = 8.4 Hz, 1H), 7.23 (d, J = 8.4 Hz, 1H), 3.91 (s, 3H), 3.21 (t, J = 8.0 Hz, 2H), 3.03 (t, J = 8.0, 2H); 13C NMR (100 MHz, DMSO-d6) δ 165.3, 161.6, 147.1, 136.5, 131.9, 128.0, 127.6, 124.9, 124.3, 124.0, 121.2, 113.5, 111.8, 111.6, 52.3, 31.7, 18.2. HRMS calcd for C17H13BrN2O2: 356.0160, found: 356.0201.
Methyl 6-methyl-6,11-dihydro-5H-pyrido[3,2-a]carbazole-2-carboxylate (8c): yellow solids in 91% yield of the two steps, mp 163–165 °C (after LC); IR (KBr) νmax = 3555, 3142, 2953, 2482, 2012, 1892, 1730, 1713, 1605, 1553, 1479, 1441, 1412, 1364, 1323, 1308, 1290, 1277, 1233, 1136, 1076, 1049, 117, 980, 922, 882, 812, 764, 743 cm-1; 1H NMR (400 MHz, DMSO-d6) δ 11.80 (s, 1H), 8.81 (d, J = 1.6 Hz, 1H), 8.56 (d, J = 1.6 Hz, 1H), 7.61 (d, J = 7.6 Hz, 1H), 7.40 (d, J = 8.0 Hz, 1H), 7.15 (d, J = 7.6 Hz, 1H), 7.03 (d, J = 7.6 Hz, 1H), 3.92 (s, 3H), 3.46–3.35 (m, 2H), 3.03 (dd, J = 16.4, 7.2 Hz, 1H), 1.24 (d, J = 6.0 Hz, 3H); 13C NMR (100 MHz, DMSO-d6) δ 165.7, 160.7, 146.4, 138.5, 129.6, 128.1, 126.0, 125.1, 124.9, 123.1, 119.9, 119.7, 118.0, 112.2, 52.9, 26.2, 21.4. HRMS calcd for C18H16N2O2: 292.1212, found: 292.1182.
Methyl 6-phenyl-6,11-dihydro-5H-pyrido[3,2-a]carbazole-2-carboxylate (8d): yellow solids in 95% yield of the two steps, mp 233–235 °C (after LC); IR (KBr) νmax = 3096, 2499, 2002, 1890, 174, 1630, 1564, 1497, 1454, 1439, 1417, 1352, 1325, 1292, 1215, 1126, 109, 985920, 895, 814, 743 cm-1; 1H NMR (400 MHz, DMSO-d6) δ 12.05 (s, 1H), 8.77 (d, J = 13.6 Hz, 2H), 7.42 (d, J = 8.4 Hz, 1H), 7.24–7.19 (m, 5H), 7.13 (t, J = 8.0 Hz, 1H), 6.99 (d, J = 8.0 Hz, 1H), 6.88 (t, J = 7.6 Hz, 1H), 4.70 (t, J = 7.2 Hz, 1H), 3.95 (s, 3H), 3.71 (dd, J = 16.8, 7.6 Hz, 1H), 3.45 (dd, J = 16.8, 6.4 Hz, 1H). HRMS calcd for C23H18N2O2: 354.1368, found: 354.1369.
Methyl 6,6-dimethyl-6,11-dihydro-5H-pyrido[3,2-a]carbazole-2-carboxylate (10): Pale yellowish solids in 74% yield, mp 217–220 °C (after LC); IR (KBr) νmax = 3433, 3096, 2949, 2498, 2021, 1904, 1744, 1630, 1560, 1499, 1456, 1416, 1325, 1304, 1267, 1223, 1194, 1136, 1119, 1096, 1034, 1013, 980, 924, 882, 814, 760 cm-1; 1H NMR (400 MHz, DMSO-d6) δ 11.86 (s, 1H), 8.83 (t, J = 2.0 Hz, 1H), 8.61 (d, J = 2.0 Hz, 1H), 7.77 (d, J = 8.4 Hz, 1H), 7.41 (d, J = 8.0 Hz, 1H), 7.16 (t, J = 7.6 Hz, 1H), 7.03 (t, J = 7.6 Hz, 1H), 3.94 (s, 3H), 3.13 (s, 2H), 1.43 (s, 6H); 13C NMR (100 MHz, DMSO-d6) δ 164.4, 158.4, 142.9, 138.4, 129.7, 127.9, 125.7, 125.6, 124.7, 123.0, 121.5, 120.5, 119.6, 112.0, 52.7, 45.9, 32.9, 28.4(2C). HRMS calcd for C19H18N2O2: 306.1368, found: 306.1319.
Preparation of methyl 11H-pyrido[3,2-a]carbazole-2-carboxylate (1a), a general procedure: A mixture of 8a (140 mg, 0.5 mmol) and DDQ (227 mg, 1.0 mmol) was dissolved in benzene (10 mL) and then heated at reflux for 4 h under the monitoring of TLC (hexane:THP = 2:1). After the reactions completed, the reaction was quenched with saturated aqueous NaHCO3 (30 mL) and extracted with EtOAc (30 mL x 3). The organic phase was collected and washed with saturated aqueous NaHCO3 and brine to give 1a.
Methyl 11H-pyrido[3,2-a]carbazole-2-carboxylate (1a): Pale yellowish solids in 74% yield, mp 272–275 °C (after LC); IR (KBr) νmax = 3185, 2949, 1865, 1788, 1732, 1614, 1597, 1564, 1524, 1497, 1456, 1439, 1412, 1366, 1329, 1290, 1254, 1233, 1206, 1125, 1053, 1011, 991, 926, 854, 831, 810, 748 cm-1; 1H NMR (400 MHz, CDCl3) δ 9.47 (s, 1H), 9.25 (s, 1H), 9.20 (br s, 1H), 8.49 (d, J = 8.8 Hz, 1H), 8.17 (d, J = 8.8 Hz, 1H), 7.99 (d, J = 8.8 Hz, 1H), 7.65 (d, J = 8.4 Hz, 1H), 7.52 (t, J = 7.6 Hz, 1H), 7.38 (t, J = 7.6 Hz, 1H), 4.06 (s, 3H); 13C NMR (100 MHz, DMSO-d6) δ 165.9, 149.1, 148.3, 139.7, 135.6, 133.2, 126.3, 125.8, 122.9, 121.8, 120.6, 120.3, 120.1, 118.7, 115.4, 112.1, 52.9. HRMS calcd for C17H12N2O2: 276.0899, found: 276.0853.
Methyl 8-bromo-11H-pyrido[3,2-a]carbazole-2-carboxylate (1b): Pale yellowish solids in 81% yield, mp 307–310 °C (after LC); IR (KBr) νmax = 3163, 2953, 2214, 1881, 1794, 1724, 1614, 1593, 1564, 1522, 1479, 1445, 1408, 1366, 1333, 1292, 1273, 1258, 1229, 1206, 1115, 1044, 984, 926, 856, 831, 814, 764, 737 cm-1; 1H NMR (400 MHz, DMSO-d6) δ 12.87 (s, 1H), 9.55 (d, J = 2.0 Hz, 1H), 9.31 (d, J = 2.4 Hz, 1H), 8.62 (d, J = 8.8 Hz, 1H), 8.48 (d, J = 1.6 Hz, 1H), 7.79 (d, J = 8.8 Hz, 1H), 7.63 (d, J = 8.4 Hz, 1H), 7.56 (dd, J = 8.8, 2.0 Hz, 1H), 3.99 (s, 3H); 13C NMR (100 MHz, DMSO-d6) δ 165.3, 148.8, 148.1, 137.9, 135.7, 132.8, 127.7, 126.0, 124.3, 122.7, 121.4, 120.0, 117.3, 114.9, 113.6, 112.0, 52.4. HRMS calcd for C17H11BrN2O2: 354.0004, found: 353.9977.
Methyl 6-methyl-11H-pyrido[3,2-a]carbazole-2-carboxylate (1c): Pale yellowish solids in 85% yield, mp 245–248 °C (after LC); IR (KBr) νmax = 3381, 3061, 2955, 1879, 1690, 1620, 1564, 1524, 1460, 1439, 1402, 1366, 1336, 1310, 1269, 1240, 1209, 1159, 1134, 1090, 1036, 988, 928, 856, 812, 772 cm-1; 1H NMR (400 MHz, DMSO-d6) δ 12.71 (s, 1H), 9.49 (d, J = 1.2 Hz, 1H), 9.23 (d, J = 1.2 Hz, 1H), 8.20 (d, J = 8.0 Hz, 1H), 7.68 (d, J = 8.0 Hz, 1H), 7.55 (s, 1H), 7.47 (t, J =7.6 Hz, 1H), 7.29 (t, J =7.6 Hz, 1H), 3.97 (s, 3H), 2.94 (s, 3H); 13C NMR (100 MHz, DMSO-d6) δ 165.9, 148.9, 148.4, 139.7, 139.5, 135.4, 132.7, 125.3, 123.2, 122.3, 120.9, 120.3, 120.1, 118.0, 113.7, 112.1, 52.7, 21.7. HRMS calcd for C18H14N2O2: 290.1055, found: 290.1040.
Methyl 6-phenyl-11H-pyrido[3,2-a]carbazole-2-carboxylate (1d): Pale yellowish solids in 98% yield, mp 263–266 °C (after LC); IR (KBr) νmax = 3377, 3057, 2953, 2361, 1723, 1694, 1620, 1559, 1528, 1495, 1454, 1362, 1323, 1310, 1275, 1238, 1198, 1123, 1103, 995, 934, 866, 812, 773, 745 cm-1; 1H NMR (400 MHz, DMSO-d6) δ 12.98 (s, 1H), 9.66 (d, J = 2.0 Hz, 1H), 9.32 (d, J = 2.0 Hz, 1H), 7.70–7.59 (m, 7H), 7.41 (t, J = 7.6 Hz, 1H), 731 (d, J = 8.0 Hz, 1H), 7.04 (t, J =8.0 Hz, 1H), 4.00 (s, 3H); 13C NMR (100 MHz, DMSO-d6) δ 165.9, 148.9, 148.6, 142.6, 140.1, 140.0, 136.1, 133.1, 129.2, 129.1, 128.8, 125.6, 122.4, 121.7, 121.6, 120.4, 120.0, 116.6, 114.5, 112.3, 52.9. HRMS calcd for C23H16N2O2 (M+H)+: 352.1212, found: 352.1248.
Oxidation of 10 with SeO2 and rearrangement to form 11. To a solution of 10 (153 mg, 0.5 mmol) dissolved in MeCN:H2O (3:1, 40 mL), heated to reflux, was added SeO2 (277 mg, 2.5 mmol) portion by portion (five times every one hour) and the reaction was continued for 12 h, as monitored by TLC (hexane:THP = 2:1). After the reaction finished, MeCN was removed under vacuum. The residue was extracted with EtOAc (50 mL x 3), and the organic layer was collected and washed with saturated aqueous NaHCO3 and brine to give 11 and 12 which were purified by flash column chromatography with the gradient of hexane: EtOAc = 10:1 to 1:1 v/v.
Methyl 5,6-dimethyl-11H-pyrido[3,2-a]carbazole-2-carboxylate (11): Pale yellowish solids in 36% yield, mp 294–296 °C (after LC); IR (KBr) νmax = 3362, 3051, 2953, 2922, 2854, 1871, 1703, 1609, 1566, 1508, 1449, 1418, 1373, 1350, 1327, 1302, 1265, 1229, 1134, 1109, 1086, 1049, 1028, 941, 873, 810 cm-1; 1H NMR (400 MHz, DMSO-d6) δ 12.57 (s, 1H), 9.47 (d, J = 2.0 Hz, 1H), 9.28 (d, J = 2.0 Hz, 1H), 8.27 (d, J = 8.0 Hz, 1H), 7.66 (d, J = 8.0 Hz, 1H), 7.45 (t, J = 7.2 Hz, 1H), 7.27 (t, J = 7.2 Hz, 1H), 3.98 (s, 3H), 2.90 (s, 3H), 2.75 (s, 3H); 13C NMR (100 MHz, DMSO-d6) δ 166.1, 147.5, 147.4, 139.8, 137.0, 133.7, 132.9, 125.1, 124.3, 123.3, 122.6, 120.4, 120.0, 118.1, 113.8, 112.0, 52.7, 18.2, 13.4. HRMS calcd for C19H16N2O2: 304.1212, found: 304.1263.
Methyl 6,6-dimethyl-5-oxo-6,11-dihydro-5H-pyrido[3,2-a]carbazole-2-carboxylate (12): yellow solid in 54% yield, mp 265–268 °C (after LC); IR (KBr) νmax = 3364, 3281, 2959, 1732, 1711, 1688, 1603, 1551, 1439, 1408, 1366, 1325, 1302, 1261, 1248, 1134, 1092, 1005, 924, 878, 810, 750 cm-1; 1H NMR (400 MHz, DMSO-d6) δ 12.06 (s, 1H), 9.04 (d, J = 2.0 Hz, 1H), 8.93 (d, J = 2.0 Hz, 1H), 7.79 (d, J = 8.0 Hz, 1H), 7.47 (d, J = 8.0 Hz, 1H), 7.21 (d, J = 7.6 Hz, 1H), 7.06 (t, J = 7.6 Hz, 1H), 3.98 (s, 3H), 1.59 (s, 6H); 13C NMR (100 MHz, DMSO-d6) δ 201.3, 165.1, 148.4, 146.1, 139.0, 131.2, 130.3, 129.2, 125.9, 125.4, 123.8, 120.7, 120.3, 120.2, 112.8, 53.3, 46.3, 26.9. HRMS calcd for C19H16N2O3: 320.1161, found: 320.1141.
Synthesis of 2-(methoxycarbonyl)-4-methyl-11H-pyrido[3,2-a]carbazol-4-ium iodide (13). A solution of 1a (40 mg, 0.145 mmol) and MeI (82.2 mg, 0.58 mmol) in DMF (1 mL) was stirred at 60 °C for 9 h. Removal of the volatile under reduced pressure, the solids were washed with EtOAc and hexane several times to obtain the gray solids, 51 mg, 84% yield; mp >350 °C; IR (KBr) νmax = 3451, 3078, 1721, 1632, 1601, 1547, 1503, 1458, 1435, 1393, 1368, 1333, 1294, 1273, 1252, 1221, 1165, 1126, 1105, 991, 937, 922, 797, 754 cm-1; 1H NMR (400 MHz, DMSO-d6) δ 13.16 (s, 1H), 9.95 (s, 1H), 9.74(s, 1H), 8.95 (d, J = 8.8 Hz, 1H), 8.30 (d, J = 7.6 Hz, 1H), 8.00 (d, J = 9.2 Hz, 1H), 7.68 (d, J = 9.4 Hz, 1H), 7.56 (t, J = 7.2 Hz, 1H), 7.38 (t, J = 7.2 Hz, 1H), 4.68 (s, 3H), 4.10 (s, 1H); 13C NMR (100 MHz, DMSO-d6) δ 162.6, 147.5, 140.5, 139.5, 137.6, 135.1, 131.6, 122.2, 121.3, 121.1, 121.1, 120.9, 116.3, 112.4, 108.4, 53.4, 46.2. HRMS calcd for C18H15IN2O2: 418.0184, found: 418.0217.
Anti-proliferative activity screening test
Anti-proliferative assays in vitro were performed in the same manner as described in our previous papers.32,33
ACKNOWLEDGEMENT
We are grateful to Okayama University for its support by Promotion of Graduate Course Students to E.S. and to the Advanced Science Research Center for the NMR experiments, EA by Mr. M. Kobayashi and Ms. M. Kosaka. This study was partially supported by the Adaptable and Seamless Technology Transfer Program of JST.
Supporting Information
Experimental procedure and spectral data including 1H NMR and 13C NMR spectra of 8a–8d, 10, 1a–1d, 11, 12 and 13 are provided.
References
1. N. J. Rahier, C. J. Thomas, and S. M. Hecht, in Anticancer Agents from Natural Products, 2nd ed. by G. M. Cragg, D. G. I. Kingston, and D. J. Newman, CRC press, Boca Raton, FL, 2012, pp. 5–25.
2. Review: C. M. Miller and F. O. McCarthy, RSC Advances, 2012, 2, 8883.
3. S. Goodwin, A. F. Smith, and E. C. Horning, J. Am. Chem. Soc., 1959, 81, 1903. CrossRef
4. a) M. Stiborová, C. A. Bieler, M. Wiessler, and E. Frei, Biochem. Pharmacol., 2001, 62, 1675; CrossRef b) M. Stiborova, J. Poljakova, E. Martinkova, L. Booek-Dohalska, T. Eckschlager, R. Kizek, and E. Frei, Interdiscip. Toxicol., 2011, 4, 98; CrossRef c) A. M. Pohlit, L. F. Rocha e Silva, M. C. Henrique, A. Montoia, R. C. N. Amorim, S. M. Nunomura, and V. F. Andrade-Neto, Phytomedicine, 2012, 19, 1049. CrossRef
5. S. C. Jain, K. K. Bhandary, and H. M. Sobell, J. Mol. Bio., 1979, 135, 813. CrossRef
6. J. Y. Kim, S. G. Lee, J.-Y. Chung, Y.-J. Kim, J.-E. Park, H. Koh, M. S. Han, Y. C. Park, Y. H. Yoo, and J.-M. Kim, Toxicology, 2011, 289, 91. CrossRef
7. E. Lescot, G. Muzard, J. Markovits, J. Belleney, B. P. Roques, and J. B. Le Pecq, J. Med. Chem., 1986, 29, 1731. CrossRef
8. A. K. Shanazarov, V. G. Granik, N. I. Andreeva, S. M. Golovina, and M. D. Mashkovskii, Khim.-Farmatsevticheskii Zhurnal, 1989, 23, 1197.
9. G. Alunni-Bistocchi, P. Orvietani, O. Mauffret, S. El Antri, A. Deroussent, P. C. Jacquignon, S. Fermandjian, A. Riccia, and E. Lescot, J. Chem. Soc., Perkin Trans. 1, 1992, 2935. CrossRef
10. J. Bergman and R. Carlsson, Tetrahedron Lett., 1978, 4051. CrossRef
11. L. K. Dalton, S. Demerac, and T. Teitei, Aust. J. Chem., 1969, 22, 185. CrossRef
12. S. Kano, E. Sugino, S. Shibuya, and S. Hibino, J. Org. Chem., 1981, 46, 2979. CrossRef
13. M. Sainsbury, B. Webb, and R. Schinazi, J. Chem. Soc., Perkin Trans. 1, 1975, 289. CrossRef
14. M. G. Saulnier and G. W. Gribble, J. Org. Chem., 1983, 48, 2690. CrossRef
15. C. May and C. J. Moody, J. Chem. Soc., Chem. Commun., 1984, 926. CrossRef
16. T. R. Govindachari and V. Sudarsanam, Ind. J. Chem., 1971, 9, 402.
17. G. R. Clemo and D. G. I. Felton, J. Chem. Soc., 1951, 671. CrossRef
18. M. Marshall and R. H. F. Manske, Can. J. Chem., 1952, 30, 711. CrossRef
19. M. G. Ferlin, O. Gia, and L. Dalla Via, ChemMedChem, 2011, 6, 1872. CrossRef
20. D. Cohylakis, G. Hignett, K. V. Lichman, and J. A. Joule, J. Chem. Soc., Perkin Trans. 1, 1974, 1518. CrossRef
21. a) C. Minot, P. Roland-Gosselin, and C. Thal, Tetrahedron, 1980, 36, 1209; CrossRef b) C. Dieng, C. Thal, H. P. Husson, and P. Potier, J. Heterocycl. Chem., 1975, 12, 455. CrossRef
22. Fischer indole synthesis, reviews: A. Gupta, B. Kamble, N. M. Joghee, and C. M. J. Nanjan, Curr. Org. Synth., 2012, 9, 377. CrossRef
23. D. L. Hughes, Org. Prep. Proc. Internat., 1993, 25, 607. CrossRef
24. M. Martin, L. J. Dorn, and J. M. Cook, Heterocycles, 1993, 36, 157. CrossRef
25. A. Jirgensons, C. G. R. Parsons, I. Jaunzeme, I. Kalvinsh, M. Henrich, M. Vanejevs, T. Weil, V. Kauss, and W. Danysz, US 20050197361 A1 20050908; PCT Int. Appl. (2005), WO 2005082856 A2 20050909.
26. S. Torii, T. Inokuchi, and M. Kubota, Synthesis, 1986, 400. CrossRef
27. Y. Huang and R. W. Hartmann, Synth. Commun., 1998, 28, 1197. CrossRef
28. P. L. Jackson, C. D. Hanson, A. K. Farrell, R. J. Butcher, J. P. Stables, N. D. Eddington, and K. R. Scott, Eur. J. Med. Chem., 2012, 51, 42. CrossRef
29. H. E. Zimmerman and P. H. Lamers. J. Org. Chem., 1989, 54, 5788. CrossRef
30. Y.-H. Lai, J. Chem. Soc., Perkin Trans. 2, 1986, 1667. CrossRef
31. J. J. Eisch, C. A. Kovacs, and P. Chobe, J. Org. Chem., 1989, 54, 1275. CrossRef
32. L. Wang, M. Świtalska, Z.-W. Mei, W.-J. Lu, Y. Takahara, X. W. Feng, I. E. El-Sayed, J. Wietrzyk, and T. Inokuchi, Bioorg. Med. Chem., 2012, 20, 4820. CrossRef
33. W.-J. Lu, M. Świtalska, L. Wang, M. Yonezawa, I. El-Sayed, J. Wietrzyk, and T. Inokuchi, Med. Chem. Res., 2013, 22, 4492. CrossRef
34. N. Wang, M. Świtalska, M.-Y. Wu, K. Imai, T. A. Ngoc, C.-Q. Pang, L. Wang, J. Wietrzyk, and T. Inokuchi, Eur. J. Med. Chem., 2014, 69, 294.