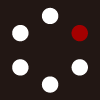
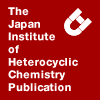
HETEROCYCLES
An International Journal for Reviews and Communications in Heterocyclic ChemistryWeb Edition ISSN: 1881-0942
Published online by The Japan Institute of Heterocyclic Chemistry
e-Journal
Full Text HTML
Received, 18th April, 2014, Accepted, 18th June, 2014, Published online, 2nd July, 2014.
DOI: 10.3987/COM-14-13007
■ 9-Alkylacridine Synthesis Using 2,2-Dimethoxypropane as Water Scavenger
George Bratulescu*
Department of Chemistry, University of Craiova, 13 A.I. Cuza, 200396 Craiova, Romania
Abstract
A rapid and high yielding green method was developed for acridine derivative synthesis in solvent free medium. The commercial reagents are easily converted into products by using of microwaves in a heterogeneous medium in presence of zinc chloride and 2,2-dimethoxypropane. Principal advantages of the procedure are short time, low energy consumption, and easy work-upStart here.) All manuscripts must be accompanied by an abstract.Acridines were first used commercially as organic dyes.1 Currently, acridines are well known for their cytotoxic activity and are used as anticancer drugs.2-5 Selected substituted acridine derivatives exhibit antiviral properties6-8 against Herpes simplex-1 and Herpes simplex-2;9 anti-inflammatory, analgesic and kinase inhibition activities;10,11 and telomerase inhibition.12-14 Certain functionalized 9-acridines show antiprion activity.15,16
Conventional methods currently used to synthesize the acridines include high-temperature condensation between carboxylic acids and diarylamines or reaction of aromatic primary amines with o-halogenobenzoic acids.17,18 Both protocols require extensive processing to obtain the products, and the yield is below expectations.19 Other routine acridine syntheses employ methods attributed to Goldberg, Friedlander, Ullman, Pfitzinger, or Bernthsen.1 Alternatively, new methods for preparation of acridines have been introduced requiring scarce, expensive, metal catalysts20,21 and multi-step cascade heterocyclizations.22
Traditionally, the Bernthsen method of acridine synthesis uses a mixture of carboxylic acid and aromatic amines, which are heated to a high temperature for 48 h.17,18 The protocol uses Lewis acids, such as zinc chloride, or Brönsted acids, such as polyphosphoric acid. These catalysts are unable to change the temperature at which reactions occur: 230 °C for 16 h (yield 18-20%) if zinc chloride is employed and 200 °C for 15 min when polyphosphoric acid is used (yield below 15%).23,24 Each of these conventional methods exhibited multiple drawbacks: high reaction temperature, long reaction times, generation of substantial amounts of waste products, intensive energy consumption, and mediocre yields.23,25,26 Recently, several unconventional protocols involving microwaves27-30 and ultrasonic irradiation31,32 were developed for acridine synthesis.
Using the Bernthsen reaction, selected 9-arylacridine derivatives were synthesized. Diphenylamine, aromatic carboxylic acids, ZnCl2, as a catalyst, and 2,2-dimethoxypropane (DMP), as a water scavenger, were employed as starting reagents. This is the first synthesis of acridines performed by the Bernthsen reaction and mediated by the water scavenger, DMP (Scheme 1).
The synthesis of 9-benzylacridine was chosen for determining optimal reaction conditions (Table 1). After 7 min (entry 5), using ZnCl2 as a catalyst without DMP, the reaction yield reached 20%. Condensation between diphenylamine and benzoic acid does not occur if the catalyst is absent from the reaction medium. A higher yield and a shorter reaction time are obtained if the reaction medium includes both the catalyst and DMP. The optimal molar ratio of reagents was determined to be —diphenylamine:phenylacetic acid:DMP equal to 1:1:2.
Reaction did not occur when conventional heating in an oil bath was used for several minutes in the presence of ZnCl2. After 12 h at a temperature of 220 °C, the yield was 21% (entry 2). When the reaction medium contained DMP, the yield of 9-benzylacridine was 36% (entry 1) by conventional heating. An excess of phenylacetic acid (entry 7) or an excess of diphenylamine (entry 8) do not increase the yield. The acid absorbs microwaves better than amine in the reaction medium (entry 7) and the yield is better. Reducing the amount of catalyst and scavenger decreases the yield (entry 10). Increasing the irradiation time, when the ratio of reactants is optimal, does not improves the yield (entry 9). The optimal values determined for the synthesis of 9-benzylacridine were used as the starting point for the synthesis of a series of 9-substituted acridine derivatives. The addition of DMP improved the yields of acridine derivatives by this synthetic route (Table 2). Moreover, temperature and reaction time decreased. Consumption of the amine and carboxylic acid reagents was optimal. The experimental protocol developed in this research was more flexible and the products were more easily recovered from the reaction medium. Reduction in the amount of organic wastes produced was an advantage of this protocol. Additionally, cumbersome processes of separation and purification of acridine derivatives were no longer required.
The consumption of water molecules by DMP shifts the reaction equilibrium toward the product, i.e., the acridine derivatives. Furthermore, DMP and its decomposition products—formed by reaction with water, acetone, and methanol—are highly polar substances and easily absorb microwaves in the reaction medium, thereby increasing the yield of the synthesis.
In conclusion, an efficient method for synthesis of 9-substituded acridine derivatives involving DMP and microwaves was developed. The reaction was performed gently, rapidly, and with high yield. The products were more easily separated from the reaction medium as compared to other methods for synthesis of acridines using microwaves or ultrasound irradiation.27-32 Pure products were obtained by recrystallization of the reaction mixture. The harsh conditions of conventional acridine derivative syntheses were avoided by using this method. Furthermore, energy consumption was substantially reduced compared to all other synthetic routes.33-36
EXPERIMENTAL
All reagents, diphenylamine, DMP, carboxylic acids, and zinc chloride are commercial compounds. Synthesis occurred in a Microsynth microwave reactor. The synthesized products were identified by elemental analysis, 1H-NMR, IR, and 13C-NMR. 1H-NMR and 13C-NMR spectra were measured on BRUKER ARX instrument at 300 MHz and 100 MHz, respectively. CDCl3 was used as solvent and tetramethylsilane as internal standard. The infrared spectra were recorded using an Alpha Bruker Optics spectrometer. Microanalyses were carried out on a Carlo Erba CHN analyzer, model 1106. The melting points were determined on a Gallenkamp digital melting point apparatus.
General method for the synthesis of acridine derivatives (1-10)
In a Pyrex beaker was introduced, diphenylamine (0.01 mol), DMP (0.02 mol), ZnCl2 (0.02 mol) and carboxylic acid (0.01 mol). The resulting combination was stirred and irradiated at 100 °C for required time. The residue generated by the reaction was recrystallized from EtOH–toluene to isolate the acridine products (Table 2).
9-Methylacridine (1).33 Yield: 84%; mp 118-119 °C;. Anal. Calcd for: C14H11N: C, 87.04; H, 5.69; N, 7.25. Found C, 87.03; H, 5.64; N, 7.21; IR: 3021w, 1518m, 1436m, 1414s, 755vs cm-1. 1H-NMR: δ=8.19 (d, J=8.6, 2H, H-4,5), 8.16 (d, J=8.8, 2H, H-1,8), 7.72 (dd, J=8.6, J=7.8, 2H, H-3,6), 7.49 (dd, J=8.8, J=7.8, 2H, H-2,7), 3.02 (s, 3H, CH3); 13C-NMR: δ=13.5, 124.4, 125.3, 125.5, 129.6, 130.2, 142, 148.4.
9-Benzylacridine (2).23
a) Microwave heating.
In a 25 mL Pyrex beaker was introduced, diphenylamine (1.69 g; 0.01 mol), DMP (2.08 g; 0.02 mol), ZnCl2 (2.72g; 0.02 mol) and phenylacetic acid (1.36 g; 0.01 mol). The resulting mixture was irradiated at 100 °C for 6 min. After this time, the reaction mixture was cooled to room temperature. The residue generated by the reaction was recrystallized from 100 mL EtOH–toluene (1:2) to obtain 9-benzylacridine. The progress of the reactions was monitored by TLC using Merck silica gel 60 F254 plates (eluent, MeOH:benzene=1:1). The plates were illuminated under UV (254 nm) and evaluated in iodine vapors. (Rf=0.48). Yield: 80%; mp 170-171 °C; Anal. Calcd for C20H15N: C, 89.21; H, 5.57; N, 5.20. Found C, 89.19; H, 5.55; N, 5.12; IR: 3039w, 1511s, 1451m, 1410s, 770vs cm-1. 1H-NMR: δ=8.17 (d, J=8.7, 2H, H-4,5), 8.14 (d, J=8.9, 2H, H-1,8), 7.74 (dd, J=8.7, J=7.5, 2H, H-3,6), 7.50 (dd, J=8.9, J=7.7, 2H, H-2,6), 7.30 (m, 2H, H-m,m'), 7.25 (m, 1H, H-p), 7.19 (m, 2H, H-o,o'), 3.84 (s, 2H, CH2); 13C-NMR: δ=38.2, 123.6, 125.3, 125.5, 126.3, 128.2, 129.1, 129.7, 137.7, 130.1, 141.3, 147.4.
b) Conventional thermal heating.
In a 25 mL Pyrex beaker was introduced, diphenylamine (1.69 g; 0.01 mol), DMP (2.08 g; 0.02 mol), ZnCl2 (2.72g; 0.02 mol) and phenylacetic acid (2.04 g; 0.015 mol). The reaction mixture was heated in an oil bath at 220 ºC for 12 h. The residue generated by the reaction was recrystallized from 100 mL EtOH–toluene (1:2) to obtain 9-benzylacridine. After this time, the reaction mixture was cooled to room temperature. The progress of the reactions was monitored by TLC using Merck silica gel 60 F254 plates (eluent, MeOH:benzene =1:1). The plates were illuminated under UV (254 nm) and evaluated in iodine vapors. (Rf=0.48). Yield: 36%; mp 170-171 °C; Anal. Calcd for C20H15N: C, 89.21; H, 5.57; N, 5.20. Found C, 89.19; H, 5.55; N, 5.12; IR: 3039w, 1511s, 1451m, 1410s, 770vs cm-1. 1H-NMR: δ=8.17 (d, J=8.7, 2H, H-4,5), 8.14 (d, J=8.9, 2H, H-1,8), 7.74 (dd, J=8.7, J=7.5, 2H, H-3,6), 7.50 (dd, J=8.9, J=7.7, 2H, H-2,6), 7.30 (m, 2H, H-m,m'), 7.25 (m, 1H, H-p), 7.19 (m, 2H, H-o,o'), 3.84 (s, 2H, CH2); 13C-NMR: δ=38.2, 123.6, 125.3, 125.5, 126.3, 128.2, 129.1, 129.7, 137.7, 130.1, 141.3, 147.4.
9-(4-Methylbenzyl)acridine (3).34 Yield: 79%; mp 147-148 °C; Anal. Calcd for C21H17N: C, 89.04; H, 6.00; N, 4.94. Found C, 88.99; H, 5.93; N, 4.90; IR: 3053w, 1503s, 1465m, 1407s, 761vs cm-1. 1H-NMR: δ=8.16 (d, J=8.2, 2H, H-4,5), 7.93 (d, J=8.4, 2H, H-1,8), 7.70 (dd, J=8.2, J=6.9, 2H, H-3,6), 7.53 (dd, J=8.4, J=6.9, 2H, H-2,7), 7.19 (d, J=8.1, 2H, H-m,m'), 7.08 (d, J=8.1, 2H, H-o,o'), 3.81 (s, 2H, CH2), 2.23 (s, 3H, CH3); 13C-NMR: δ=21.4, 38.3, 124.3, 126.1, 126.5, 128.8, 129.1, 129.6, 130.2, 133.4, 134.9, 142.3, 147.6.
9-(4-Nitrobenzyl)acridine (4).35 Yield: 92%; mp 256-257 °C; Anal. Calcd for C20H14N2O2: C, 76.43; H, 4.45; N, 8.91. Found C, 76.41; H, 4.41; N, 8.86; IR: 3037w, 1526vs (NO2as), 1459m, 1402s, 1378vs (NO2s), 732vs cm-1. 1H-NMR: δ=8.12 (d, J=8.6, 2H, H-4,5), 8.09 (d, J=9, 2H, H-m,m'), 7.98 (d, J=8.8, 2H, H-1,8), 7.73 (dd, J=8.6, J=7.7, 2H, H-3,6), 7.60 (dd, J=8.8, J=7.7, 2H, H-2,7), 7.33 (d, J=9.1, 2H, H-o,o'), 3.89 (s, 2H, CH2); 13C-NMR: δ=37.2, 123.2, 124.1, 126.2, 126.8, 128.7, 129.3, 130.2, 141.2, 145.3, 146.4, 147.6.
9-(Naphthalen-1-ylmethyl)acridine (5).34 Yield: 71%; mp 185-186 °C; Anal. Calcd for C23H15N: C, 90.28; H, 5.32; N, 4.38. Found: C, 90.16; H, 5.27; N, 4.32; IR: 3031w, 1500vs, 1470m, 1410m, 773s cm-1. 1H-NMR: δ=8.15 (d, J=8.7, 2H, H-4,5), 7.26-7.91 (m, 11H, C10H7, H-2,3,6,7), 7.96 (d, J=8.9, 2H, H-4,5), 5.51 (s, 2H, CH2); 13C-NMR: δ=35.8, 118.2, 119.1, 124.2, 125.2, 126.1, 126.3, 127.2, 128.2, 128.3, 128.5, 129.6, 131.4, 132.7, 133.5, 134.1, 141.8, 145.7.
9-Ethylacridine (6).33 Yield: 83%; mp 113-114 °C; Anal. Calcd for C15H13N: C, 86.95; H, 6.28; N, 6.76. Found: C, 86.92; H, 6.24; N, 6.76; IR: 3021w, 1524s, 1440m, 1399m, 758vs cm-1. 1H-NMR: δ=8.19 (d, J=8.7, 2H, H-4,5), 7.88 (d, J=8.8, 2H, H-1,8), 7.70 (dd, J=8.7, J=7.3, 2H, H-3,6), 7.44 (dd, J=8.8, J=7.3, 2H, H-2,7), 2.62 (q, J=7.8, 2H, CH2), 1.24 (t, J=7.8, 3H, CH3); 13C-NMR: δ=14.29, 28.16, 121.8, 123.7, 126.4, 128.8, 129.2, 141.3, 147.2.
9-Propylacridine (7).33 Yield: 79%; mp 124-125 °C; Anal. Calcd for C16H15N: C, 86.87; H, 6.78; N, 6.33. Found: C, 86.77; H, 7.20; N, 5.94; IR: 3016w, 1518m, 1448s, 1358m, 752vs cm-1. 1H-NMR: δ=8.19 (d, J=8.6, 2H, H-4,5), 7.86 (d, J=8.8, 2H, H-1,8), 7.72 (dd, J=8.6, J=7.5, 2H, H-3,6), 7.41 (dd, J=8.8, J=7.5, 2H, H-2,7), 2.60 (q, J=7.7, 2H, CH2), 1.64 (m, 2H, CH2), 1.03 (t, J=7.9, 3H, CH3); 13C-NMR: δ= 13.6, 24.8, 33.9, 121.7, 123.6, 126.2, 128.4, 130.1, 140.8, 148.2.
9-(3-Phenylpropyl)acridine (8).33 Yield: 80%; mp 102-103 °C; Anal. Calcd for C22H19N: C, 88.88; H, 6.39; N, 4.71. Found: C, 88.87; H, 6.37; N, 4.69; IR: 3042w, 1540s, 1455m, 1423s, 758vs cm-1. 1H-NMR: δ=8.18 (d, J=8.5, 2H, H-4,5), 8.10 (d, J=8.7, 2H, H-1,8), 7.69 (dd, J=8.5, J=7.2, H-3,6), 7.60 (dd, J=8.7, J=7.2, 2H, H-2,7), 7.18-7.44 (m, 5H, C6H5), 2.64 (t, J=7.9, 2H, CH2), 2.58 (t, J=7.6, 2H, CH2), 1.91 (m, 2H, CH2); 13C-NMR: δ=29.3, 31.6, 35.1, 124.2, 126.1, 126.5, 126.9, 128.1, 128.6, 129.1, 129.7, 141.4, 142.3, 147.2.
9-(3-Hydroxyropropyl)acridine (9).36 Yield: 76%; mp 224-225 °C; Anal. Calcd for C16H15NO: C, 81.01; H, 6.32; N, 5.90. Found C, 80.93; H, 6.31; N, 5.87; IR: 3147s, 1504s, 1467m, 1411s, 768s; 1H-NMR: δ=8.60 (d, J=8.8, 2H, H-4,5), 8.20 (d, J=8.8, 2H, H-1,8), 8.02 (dd, J=8.8, J=7.7, 2H, H-3,6), 7.77 (dd, J=8.8, J=7.7, 2H, H-2,7), 7.51 (br, s, 1H, OH), 3.89 (t, J=6.1, CH2O), 3.78 (t, J=6.2, 2H, CH2), 2.05 (m, 2H, CH2); 13C-NMR: δ=26.7, 35.7, 61.9, 121.6, 126.1, 127.5, 129.1, 137.9, 141.1, 164.2.
9-Pentylacridine (10).37 Yield: 72%; mp 69-70 °C; Anal. Calcd for C18H19N: C, 86.74; H, 7.63; N, 5.62. Found: C, 86.70; H, 7.59; N, 5.58; IR: 3032s, 1527m, 1432s, 1394s, 790vs. 1H-NMR: δ=8.23 (d, J=7.5, 2H, H-4,5), 8.12 (d, J=7.6, 2H, H-1,8), 7.75 (dd, J=7.5, J=6.8, 2H, H-3,6), 7.54 (dd, J=7.6, J=6.8, 2H, H-2,7), 3.60 (t, J=7.6, 2H, CH2), 1.82 (m, 2H, CH2), 1.55 (m, 2H, CH2), 1.43 (m, 2H, CH2), 0.93 (t, J=7.2, 3H, CH3); 13C-NMR: δ=14.1, 22.6, 27.9, 31.2, 32.3, 124.4, 125.1, 125.8, 130, 130.7, 147.7, 149.2.
References
1. A. Albert, " The Acridines" , St. Martin’s Press, New York, 1966, pp. 403-504.
2. O. Sedlacek, M. Hruby, M. Studenovsky, D. Vetvicka, J. Svoboda, D. Kankova, J. Kovar, and K. Ulbrich, Bioorg. Med. Chem., 2012, 20, 4056. CrossRef
3. S. M. Sondhi, M. Johar, N. Singhal, S. G. Dastidar, R. Shukla, and R. Raghubir, Monatsh. Chem., 2000, 131, 511. CrossRef
4. S. A. Gamage, J. A. Spicer, G. W. Rewcastle, and W. A. Denny, Tetrahedron Lett., 1997, 38, 699. CrossRef
5. G. J. Finlaya and B. C. Baguley, Cancer Chemoth. Pharm., 2000, 45, 417. CrossRef
6. O. I. El-Sabbagh and H. M. Rady, Eur. J. Med. Chem., 2009, 44, 3680. CrossRef
7. S. A. Lyakhov, Y. I.Suveyzdis, L. A. Litvinova, S. A. Andronati, S. L. Rybalko, and S. T. Dyadyun, Pharmazie, 2000, 55, 733.
8. A. Bishnoi, R. Saxena, S. Singh, A. Rani, M. N. Joshi, and S. K. Bajpai, Lett. Drug. Des. Discov., 2009, 6, 252. CrossRef
9. J. R. Goodell, A. V. Ougolkov, H. Hiasa, H. Kaur, R. Remmel, D. D. Billadeau, and D. M. Ferguson, J. Med. Chem., 2008, 51, 179. CrossRef
10. S. M. Sondhi, N. Singh, A. M. Lahoti, K. Bajaj, A. Kumar, O. Lozach, and L. Meijer, Bioorg. Med. Chem., 2005, 13, 4291. CrossRef
11. S. M. Sondhi, G. Bhattacharjee, R. K. Jameel, R. Shukla, R. Raghubir, O. Lozach, and L.Meijer, Cent. Eur. J. Chem., 2004, 2, 1. CrossRef
12. S. Sparapani, S. M. Haider, F. Doria, M. Gunaratnam, and S. Neidle, J. Am. Chem. Soc., 2010, 132, 12263. CrossRef
13. M. Laronze-Cochard, Y. M. Kim, B. Brassart, J. F. Riou, J. Y. Laronze, and J. Sapi, Eur. J. Med. Chem., 2009, 44, 3880. CrossRef
14. M. Gunaratnam, O. Greciano, C. Martins, A. P. Reszka, C. M. Schultes, H. Morjani, J. F. Riou, and S. Neidle, Biochem. Pharm., 2007, 74, 679. CrossRef
15. H. T. N. Thi, C. Y. Lee, K. Teruya, W. Y. Ong, K. Doh-Ura, M. L. Go, Bioorg. Med. Chem., 2008, 16, 6737. CrossRef
16. S. Dollinger, S. Lober, R. Klingenstein, C. Korth, and P. Gmeiner, J. Med. Chem., 2006, 49, 6591. CrossRef
17. A. Bernthsen, Ann., 1878, 192, 1.
18. A. Bernthsen, Ann., 1884, 224, 1.
19. B. C. Sekhar, J. Heterocycl. Chem., 2004, 41, 807. CrossRef
20. P. Belmont, J. C. Andrez, and C. S. M. Allan, Tetrahedron Lett., 2004, 45, 2783. CrossRef
21. R. Csuk, A. Barthel, and C. Raschke, Tetrahedron, 2004, 60, 5737. CrossRef
22. A. Fraleoni-Morgera and P. Zanirato, ARKIVOC, 2006, xii, 111. CrossRef
23. F. D. Popp, J. Org. Chem., 1962, 27, 2658. CrossRef
24. J. Joseph, E. Kuruvilla, A. T. Achuthan, D. Ramaiah, and G. B. Schuster, Bioconjugate Chem., 2004, 15, 1230. CrossRef
25. N. G. Kozlov, L. I. Basalaeva, S. I. Firgang, and A. S. Shashkov, Russ. J. Org. Chem., 2004, 40, 518. CrossRef
26. M. D. Mosher, T. D. Morris, S. Westerbuhr, and C. Foster, Heterocycl. Commun., 1996, 6, 521.
27. A. I. S.Almeida, V. L. M. Silva, A. M. S. Silva, D.C.G.A Pinto, and J. A. S. Cavaleiro, Synlett, 2012, 23, 889. CrossRef
28. S.-L. Wang, G. Zhang, D. Jie, B. Jiang, X.-H. Wang, and S.-J. Tu Comb, Chem. High. T. Scr., 2012, 15, 400.
29. J. A. Seijas, M. P. Vázquez-Tato, M. M. Martínez, and J. Rodríguez-Parga, Green Chem., 2002, 4, 390. CrossRef
30. S. Tu, T. Li, Y. Zhang, F. Shi, J. Xu, Q. Wang, and J. Zhang, J. Heterocycl. Chem., 2007, 44, 83. CrossRef
31. H. Zang, Y. Zhang, Y. Mo, and B. Cheng, Synth. Commun., 2011, 41, 3207. CrossRef
32. V. V. Dabholkar and M. M. Wadkar, Indian J. Heterocycl. Chem., 2009, 18, 303. CrossRef
33. Z. Zeng and S. C. Zimmerman, Tetrahedron Lett., 1988, 29, 5123. CrossRef
34. E. Veverkova, M. Noskova, and S. Toma, Synth. Commun., 2002, 32, 729. CrossRef
35. S. Das, and A. J. Thakur, Green Chem. Lett. Rev., 2011, 4, 131. CrossRef
36. K. Okuma, N. Matsunaga, and S. Ozaki, Fukuoka Daigaku Rigaku Shuho, 2011, 41, 23.
37. Ng. Ph. Buu-Hoï, J. Chem. Soc., 1950, 1146