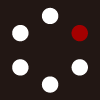
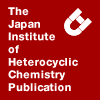
HETEROCYCLES
An International Journal for Reviews and Communications in Heterocyclic ChemistryWeb Edition ISSN: 1881-0942
Published online by The Japan Institute of Heterocyclic Chemistry
e-Journal
Full Text HTML
Received, 7th May, 2014, Accepted, 29th May, 2014, Published online, 10th June, 2014.
DOI: 10.3987/COM-14-S(K)26
■ Design and Synthesis of Conformationally Constrained Bicyclo[2.2.2]octane-Based Unusual α-Amino Acid Derivatives via the Diels–Alder Reaction
Sambasivarao Kotha* and Milind Meshram
Department of Chemistry, Indian Institute of Technology Bombay, Powai, Mumbai - 400076, India
Abstract
We report a simple synthetic approach to various conformationally constrained bicyclic α-amino acid derivatives using the Diels−Alder reaction as a key step. Moreover, we have investigated the reactivity pattern of various anthracene derivatives in relation to the Diels−Alder chemistry.INTRODUCTION
Conformationally constrained α-amino acids (AAAs) (e.g. 1‒3 and 6‒7)1–5 play a significant role in peptidomimetics. In this regard, various synthetic methods based on Strecker synthesis, Bucherer–Bergs method, and Diels–Alder (DA) strategy have been developed. Sterically constrained AAAs such as α-aminoisobutyric acid (Aib) 1 is known to be useful in the peptide design. For example, by incorporation of Aib 1 the resulting peptides adopt α-helical and/or β-sheet confirmation.1a To design peptidomimetics and peptide catalysts, racemic or meso unusual AAAs are also useful because when they are coupled with optical pure proteinogenic AAA two diastereoisomers are formed and these isomers can be separated by conventional techniques.1b
To assemble constrained phenylalanine (Phe) derivatives by the DA reaction, methyl 2-acetamidoacrylate (4) has been used as a useful synthon. In another occasion, various constrained bicyclic aliphatic AAAs2b‒c (e.g. 2a‒b) are assembled by the DA reaction of dehydroalanine derivatives. The constrained phenylalanine derivatives such as 3a–b2 were prepared by the Bucherer–Bergs reaction or Strecker synthesis. On the other hand, highly constrained bicyclic AAA derivative (e.g., 6)6a has been synthesized by the DA reaction of methyl 2-acetamidoacrylate (4) and anthracene (5). It is worth mentioning that, this strategy has found to be useful to design non-steroidal bioactive molecules.6b−i Furthermore, this methodology has been extended to 9-substituted anthracene derivatives to generate the substituted bicyclic constrained AAA derivatives 7 involving regioselective DA reaction7 (Figure 1). Therefore, systematic investigation was launched to design bicyclo[2.2.2]octane related AAA derivatives, that are useful in medicinal chemistry.
In view of our interest to generate various unusual AAA derivatives, we had generated o-xylylene (or o-quinodimethane) derivatives such as 8 and 98,9 and trapped them with methyl 2-acetamidoacrylate (4) in a DA fashion8–20 to deliver various constrained AAA derivatives. In this context, now we plan to generate a higher analog of 9, such as 10 and react it with methyl 2-acetamidoacrylate (4) to design unusual AAA derivatives (Figure 2).
To this end, here we report the reactivity pattern of anthracene derivatives 11–13 in connection with the DA chemistry. Various dienophile such as methyl 2-acetamidoacrylate (4) and dimethyl acetylenedicarboxylate (DMAD) were reacted with these anthracene derivatives. It is worth mentioning that two possible reactive sites are available in 10 during the DA reaction. The first choice involves the DA reaction of the dienophile at 9,10 position and the other option involves the dienophile reacting at outer portion of the diene. We are also keen to probe the role of the substituents in anthracene derivatives such as 11–13 during the DA reaction with methyl 2-acetamidoacrylate (4) (Figure 3).
STRATEGY
Toward the synthesis of various conformationally constrained AAA derivatives,21–27 we identified the known dibromoanthracene 12 as a key intermediate. Sultine 14, which can be generated from the dibromide 12 on thermal activation may produce the transient diene 10. Alternatively, the DA reaction of 12 with dimethyl acetylenedicarboxylate (DMAD) can deliver another key dibromide 15, which is useful to prepare various constrained AAA derivatives 17–18. Similarly, 11 on DA reaction with DMAD can also generate 19 (Figure 4).
RESULTS AND DISCUSSION
The DA reaction has been identified as a key step to design various bicyclic AAA derivatives 17–19. To this end, the known 2,3-bis(bromomethyl)anthracene (12) was chosen as a key intermediate and it was prepared by following the literature procedure.28,29
Initially, the reduction30–33 of anthracene-2,3-dicarboxylic acid did not give the diol 21. Later, the dimethyl ester 20 was treated with LiAlH4, in dry THF at low temperature (–12) to 0 ºC for 2 h to afford the desired diol 21. Formation of the diol was confirmed by the 1H and 13C NMR spectral data and further supported by mass spectral data. Here, the diol 21 was isolated (49%) first time. Next, the diol was reacted with acetic anhydride in the presence of dry pyridine to furnish a pale-yellow colored 2,3-bis(acetoxymethyl)anthracene (13) in 66% yield after crystallization with hot methanol/dichloromethane mixture. Subsequently, the diacetate 13 was subjected to bromination with 33% HBr in glacial acetic acid to afford the yellow colored 2,3-bis(bromomethyl)anthracene (12) in 91% yield after crystallization with hot methanol/dichloromethane mixture.
Next, treatment of the dibromide 12 with ethyl isocyanoacetate (EICA) (23)34,35 in the presence of tetrabutylammonium hydrogen sulfate (TBAHS) as phase-transfer catalyst (PTC) and K2CO3 as a base gave the crude isonitrile derivative, which was directly subjected to hydrolysis in absolute ethanol and conc. hydrochloric acid, and the subsequent acetylation in the presence of acetic anhydride in dry MeCN at room temperature afforded the anthracene based AAA derivative 11 in 35% yield (three-steps). Alternatively, the dibromide 12 was treated with a readily available sodium hydroxymethane sulfinate (rongalite) 22 under PTC conditions using tetrabutylammonium bromide (TBAB) in DMF to generate the anthracene-based sultine 14 in 74% yield. Since sultine 14 is partially soluble in most of the organic solvents, it was further purified by crystallization with hot CCl4. The formation of the sultine was confirmed by the 1H and 13C NMR data and further supported by mass spectral data (Scheme 1).
Next, the DA reaction of the sultine 14 was attempted with methyl 2-acetamidoacrylate (4) in toluene at 75–80 °C for 24 h and subsequently in toluene reflux for 3 days. Surprisingly, the starting material was recovered and the identity of the starting material was confirmed by the 1H NMR spectral data. Later, the sultine 14 was treated with the dienophile 4 in toluene under MWI conditions at 60 W; the desired cycloaddition product was not realized. Further, treatment of the sultine 14 with the same dienophile 4 in o-dichlorobenzene under reflux conditions did not afford the desired cycloadduct.
Having the AAA derivative 11 in hand, we have attempted the DA reaction with methyl 2-acetamidoacrylate (4) in toluene reflux for 10 days. In spite of several such attempts, the starting material 11 was recovered and it was confirmed by the 1H NMR spectral data. Later, the Lewis acid mediated DA reaction by using BCl336 and Et2AlCl37 was also found to be unsuccessful. Then, treatment of the compound 11 with 4 under MWI (70 W), the desired cycloaddition product was not realized. Next, treatment of the compound 11 with another dienophile, such as DMAD (24) under toluene reflux condition for 3 days did not afford the expected cycloadduct.
Later, we chose the other anthracene derivatives, such as 2,3-bis(bromomethyl)anthracene (12) and 2,3-bis(acetoxymethyl)anthracene (13) to realize the cycloaddition reaction. To this end, the compound 12 was reacted with methyl 2-acetamidoacrylate (4) in toluene (sealed-tube) at 140 °C for 3 days and under these conditions did not deliver the desired cycloadduct. Similarly, treatment of 2,3-bis(acetoxymethyl)anthracene (13) with the compound 4 under the same reaction conditions did not afford the desired product. Surprisingly, the starting material was recovered and its identity was confirmed by the 1H NMR spectral data. Later, we have attempted ionic-liquid38 conditions to realize the DA reaction of the compound 13 with 4 and in this case also we found no desired cycloadduct formation.
Since DMAD (24) is a better dienophile39 for the DA reaction as compared to a methyl 2-acetamidoacrylate (4), the 2,3-bis(acetoxymethyl)anthracene (13) was reacted with DMAD (24) under toluene reflux conditions. As expected the desired cycloadduct 16 was obtained as a viscous liquid in 93% yield, which was purified and then identified by 1H and 13C NMR spectroscopy techniques and further supported by mass spectral data (Scheme 2). Later, treatment of the DA adduct 16 with 33% HBr in acetic acid at room temperature for 39 h gave the corresponding dibromide 15 as a white solid in 51% yield along with the monobrominated product 25 in 15% yield.
Along similar lines, 2,3-bis(bromomethyl)anthracene (12) was reacted with DMAD (24) in refluxing toluene to furnish the desired cycloadduct 15 in excellent yield 90% (Scheme 3). Next, the cycloadduct 15 was treated with rongalite 22 in the presence of TBAB as a PTC to afford the desired sultine 26 in 73% yield, and NMR data showed the presence of diasteromers. Further, bicyclic sultines 26a and 26b were rearranged to sulfone 27 (76% yield) under toluene refluxing conditions.
Later, the cycloadduct 15 was treated with ethyl isocyanoacetate (23) in the presence of K2CO3 and PTC in refluxing MeCN conditions gave the coupling product 28. The coupling product was not purified and it was directly hydrolyzed in conc. hydrochloric acid/absolute ethanol mixture to furnish the desired amino ester. Subsequent acetylation of the amino ester with acetic anhydride in MeCN at room temperature gave the expected acylated product 19 (13%, three-steps, overall yield). TLC indicates a mixture of diasteromers of 19. However, we have isolated a single diastereomer by preparative TLC and identified by spectral data. Alternatively, the compound 15 was reacted with diethyl acetamidomalonate (DEAM) 29 in the presence of K2CO3 as a base in MeCN refluxing condition to furnish the desired Tic derivative 18 in 76% yield (Scheme 4).
Having the sultine 26 in the hand, we attempted the synthesis of the tetralin-based AAA derivative 17 via the cycloaddition reaction. To this end, the bicyclic sultine 26 was reacted with methyl 2-acetamidoacrylate (4) in refluxing toluene for 3 days to furnish the tetralin-based AAA derivative 17 in 25% yield as a mixture of diastereomers. Here, the rearranged sulfone 27 was also isolated in 30% yield (Scheme 5).
It is interesting to note that the sultine derivative 14 containing anthracene ring did not undergo DA reaction with methyl 2-acetamidoacrylate (4). However, the conjugation present in 14 is destroyed by formation of the DA adduct 26, and therefore 26 undergo DA reaction with 4.
Herein, we have successfully synthesized conformationally constrained bicyclic AAA derivatives such as tetralin-based AAA derivative 17 (11 steps), 1,2,3,4-tetrahydroisoquinoline-3-carboxylic acid (Tic) based AAA derivative 18 (10 steps), and indan-based AAA derivative 19 (12 steps) using the DA reaction as a key step. The tetracyclic compound 11, which contains an additional five membered ring on anthracene system does not behave like anthracene in the DA sequence. The sultine 14 containing anthracene unit does not participate in the DA reaction with methyl 2-acetamidoacrylate (4) and dimethyl acetylenedicarboxylate (24) even under forcing reaction conditions. However, the compound 26 undergo the DA reaction with 4.
EXPERIMENTAL
Melting points were recorded on Labhosp or Veego melting point apparatus and are uncorrected. Proton Nuclear Magnetic Resonance (1H NMR) spectra were generally recorded on Varian VXR (400 MHz) or Bruker (400 MHz), spectrometers. Carbon Nuclear Magnetic Resonance (13C NMR) spectra were recorded on Varian VXR (75 MHz) or Bruker (100 MHz) spectrometer. NMR samples were generally made in chloroform-d solvent and chemical shifts were reported in δ scale using tetramethylsilane (TMS) as the internal standard. Analytical thin-layer chromatography (TLC) was performed on glass plates coated with Acme’s silica gel G or GF 254 (containing 13% calcium sulfate as a binder).
All the reactions were monitored by employing TLC technique using appropriate solvent system for development. Transfer of moisture sensitive materials were carried out by using standard syringe-septum techniques and the reactions were maintained under nitrogen atmosphere until the work up. Dry CCl4 and CH2Cl2, were obtained by distillation over P2O5. Magnesium sulfate/sodium sulfate was dried in an oven at 130 °C for one day. All the solvent extracts were washed successively with water, brine (saturated sodium chloride solution) and dried over anhydrous magnesium sulfate/ sodium sulfate and concentrated at reduced pressure on a Buchi R-114 rotary evaporator. Yields reported are isolated yields of the products. Ethyl isocyanoacetate was prepared by following the literature procedure34,35 and methyl 2-acetamidoacrylate and diethyl acetamidomalanate were purchased from Aldrich Chemical Co., inc. Yields refer to the chromatographically isolated yield. All the commercial grade reagents were used without further purification.
Reduction with lithium aluminum hydride (LiAlH4)28,29
To a suspension of LiAlH4 (764 mg, 20.10 mmol) in THF (30 mL) at 0 ºC was added the diester 20 (1.18 g, 4.02 mmol) under N2 with stirring. Then, the reaction mixture was kept at –12 ºC to 0 ºC for 3 h. Later, the reaction mixture was quenched at 0 ºC by adding saturated solution of Na2SO4 (3 mL) in a dropwise manner, stirred for 15 min, the mixture was filtered on a glass wool and the filtrate was evaporated at reduced pressure at 40–50 ºC to obtain the product 21 (469 mg, 49%) as a yellow colored solid.
mp 247–249 ºC; IR (Neat) 3428, 3054, 2987, 1604, 1421, 1265, 740 cm-1; 1H NMR (400 MHz, CD3SOCD3) δ 8.50 (s, 2H, ArH), 8.06-8.04 (m, 2H, ArH), 8.02 (s, 2H, ArH), 7.48-7.46 (m, 2H, ArH), 5.36 (bs, 2H, OH), 4.73 (s, 4H, OCH2); 13C NMR (100 MHz, DMSO-d6) δ 138.2, 131.2, 130.6, 128.0, 125.4, 125.2, 124.7, 60 8; HRMS (Q-ToF MS ES+): m/z: (M+H)+ Calcd for C16H15O2: 239.1077. Found: 239.1072.
Synthesis of anthracene sultine (14)
This experiment was conducted by following the earlier procedure.25
In a two neck round bottom flask was added rongalite 22 (383 mg, 2.4 mmol) in DMF (6 mL). Later, to an obtained suspension was added dibromo compound 12 (90 mg, 0.24 mmol) and TBAB (37 mg, 0.1 mmol) at 0 ºC and the resulting reaction mixture was stirred (at 0 ºC for 3 h) and then at room temperature for 3 h. At the conclusion of the reaction (TLC monitoring), the reaction mixture was diluted with H2O (10 mL) and extracted with EtOAc. The organic layer was washed with H2O (2 × 10 mL), brine (2 × 10 mL), dried over anhydrous Na2SO4. Evaporation of the organic layer under reduced pressure afforded the crude product. Later, the compound was purified by crystallization using hot CCl4 to give a yellow colored solid 14 (49.2 mg, 74%).
Rf = 0.36 (silica gel, 10% EtOAc-petroleum ether); mp 250–252 ºC; IR (Neat) 3049, 2923, 1657, 1325, 1264, 747 cm-1; 1H NMR (400 MHz, CDCl3) δ 8.43 (s, 1H, ArH), δ 8.41 (s, 1H, ArH), δ 8.03-8.00 (m, 2H, ArH), δ 7.92 (s, 1H, ArH), δ 7.91 (s, 1H ArH), δ 7.52-7.48 (m, 2H, ArH), δ 5.58 (½ ABq, 1H, J = 13.1 Hz), δ 5.17 (½ ABq, 1H, J = 13.1 Hz), δ 4.74 (½ ABq, 1H, J = 15.3 Hz), δ 3.83 (½ ABq, 1H, J = 15.3 Hz); 13C NMR (100 MHz, CDCl3) δ 132.3, 132.2, 131.4, 131.2, 129.0, 128.3, 126.6, 126.4, 126.1, 125.5, 124.5, 64.5, 59.1; HRMS (Q-ToF MS ES+): m/z: (M+H)+ Calcd for C16H13O2S: 269.0638. Found: 269.0636.
Synthesis of AAA derivative (11)
This experiment was conducted by following the earlier procedure.29
In a two neck round bottom flask were added the dibromide 12 (150 mg, 0.41 mmol), tetrabutylammonium hydrogen sulfate (55.5 mg, 0.16 mmol) and K2CO3 (1.13 g, 8.19 mmol) in MeCN (15 mL). Later, the resulting reaction mixture was treated with ethyl isocyanoacetate (23) (0.5 mL, 4.11 mmol). Then the reaction mixture was stirred at 75–80 °C under N2 for 22.5 h, then cooled to room temperature, and filtered through a sintered glass funnel. The solid was washed with CH2Cl2 and the filtrate was evaporated at reduce pressure to give the crude product, which was directly subjected to hydrolysis without further purification.
The crude product in CH2Cl2 (12 mL), was reacted with dry EtOH (25 mL) in the presence of conc. HCl (1 mL). The reaction mixture was stirred at room temperature for 3 h and then diluted with CH2Cl2 (50 mL), H2O (75 mL), followed by the addition of NaHCO3 to make it alkaline. Later, the CH2Cl2 layer was washed with H2O (2 × 30 mL), dried (Na2SO4), filtered, and evaporated at reduced pressure gave the crude product was directly subjected to acetylation.
The crude product in dry MeCN (12 mL) was treated with Ac2O (0.51 mL, 5.44 mmol) at room temperature for 24 h. Evaporation of the solvent under reduced pressure to give the crude product and it was further purified by column chromatography (50% EtOAc/petroleum ether mixture) to give the pure product 11 (49.8 mg, 35%-three-steps) as a white solid.
Rf = 0.49 (silica gel, 50% EtOAc-petroleum ether); IR (Neat) 3054, 2926, 1712, 1605, 1422, 1156, 748 cm-1; 1H NMR (400 MHz, CDCl3) δ 8.32 (s, 2H, ArH), 7.97-7.95 (m, 2H, ArH), 7.78 (s, 2H, ArH), 7.45-7.42 (m, 2H, ArH), 6.05 (s, 1H, NH), 4.27 (q, 2H, J = 7.0 Hz, OCH2CH3), 3.78 and 3.47 (d, 2H, J = 16.5 Hz, ArCH2 and d, 2H, J = 16.5 Hz, ArCH2), 1.92, (s, 3H, COCH3), 1.27 (t, 3H, J = 7.0 Hz, OCH2CH3); 13C NMR (100 MHz, CDCl3) δ 172.7 (C=O amide), 170.3 (C=O ester), 139.0, 131.6, 128.1, 125.7, 125.3, 122.8, 66.4, 61.8, 42.7, 23.2, 14.2; HRMS (Q-ToF MS ES+): m/z: (M+H)+ Calcd for C22H22NO3: 348.1600. Found: 348.1590.
The DA reaction of diacetate (13) with DMAD (24)
In a 50 mL round bottom flask was added 2,3-bis(acetoxymethyl)anthracene (13) (30 mg, 0.09 mmol) was reacted with dimethyl acetylenedicarboxylate (DMAD) (24) (1.5 equiv) in toluene (3 mL). The reaction mixture was refluxed for 3 days (TLC monitoring). Evaporation of the solvent gave the crude product, which was further purified by column chromatography. Elution of the column with 30% EtOAc/petroleum ether mixture gave the desired product 16 (40.2 mg, 93%) as a viscous semi solid.
Rf = 0.37 (silica gel, 30% EtOAc-petroleum ether); 1H NMR (400 MHz, CDCl3) δ 7.44 (s, 2H, ArH), 7.39-7.37 (m, 2H, ArH), 7.04-7.02 (m, 2H, ArH), 5.49 (s, 2H, ArCH), 5.10 (s, 4H, OCH2), 3.79 (s, 6H, OCH3), 2.06 (s, 6H, COCH3); 13C NMR (100 MHz, CDCl3) δ 170.8, 165.8 (C=O ester). 146.9, 144.6, 143.3, 131.9, 125.7, 125.6, 124.1, 63.5, 52.6, 52.1, 21.0; HRMS (Q-ToF MS ES+): m/z: (M+Na)+ Calcd for C26H24O8Na: 487.1369. Found: 487.1363.
Conversion of diacetate (16) to the dibromide (15)
To a solution of the diacetate 16 (39 mg, 0.08 mmol) in CH2Cl2 (10 mL) was added a 33% HBr in acetic acid (0.5 mL). The solution was stirred at room temperature for 39 h (TLC monitoring). The reaction mixture was quenched with H2O (10 mL), extracted with CH2Cl2 (3 × 10). The organic layer was washed with H2O (15 mL), dried over anhydrous Na2SO4. Evaporation of the solvent at reduced pressure gave the crude product, which was purified by column chromatography. Elution of the column with 30% EtOAc/petroleum ether mixture afforded the desired product 15 (21 mg, 51%) as a white solid along with the mono-brominated product 25 (6 mg, 15%).
Compound 15
Rf = 0.70 (silica gel, 30% EtOAc-petroleum ether); mp 180–182 °C; IR (Neat) 3054, 2999, 2951, 1717, 1464, 1435, 1267, 737 cm-1; 1H NMR (400 MHz, CDCl3) δ 7.39-7.38 (m, 2H, ArH), 7.37 (s, 2H, ArH), 7.04-7.02 (m, 2H, ArH), 5.46 (s, 2H, ArCH), 4.56 (s, 4H, 2 × CH2Br), 3.78 (s, 6H, 2 × OCH3); 13C NMR (100 MHz, CDCl3) δ 165.7 (C=O ester), 146.7, 145.1, 143.0, 133.9, 126.4, 125.9, 124.1, 52.6, 52.0, 30.0.
HRMS (Q-ToF MS ES+): m/z: (M+H)+ Calcd for C22H19Br2O4: 504.9650. Found: 504.9650.
Compound 25
Rf = 0.58 (silica gel, 30% EtOAc-petroleum ether); mp 160–162 °C; IR (Neat) 3056, 2953, 1735, 1637, 1436, 1223, 1229, 738 cm-1; 1H NMR (400 MHz, CDCl3) δ 7.40 (s, 1H, ArH), 7.40 (s, 1H, ArH), 7.39-7.35 (m, 2H, ArH), 7.05-7.01 (m, 2H, ArH), 5.48 (s, 1H, ArCH), 5.47 (s, 1H, ArCH), 5.16 (s, 2H, OCH2), 4.50 (s, 2H, CH2Br), 3.78 (s, 6H, 2 × OCH3), 2.07 (s, 3H COCH3); 13C NMR (100 MHz, CDCl3) δ 170.8 (OAc), 165.8 (C=O ester), 146.8, 146.8, 144.9, 144.8, 143.2, 133.8, 132.0, 126.1, 126.0, 125.8, 124.1, 63.2, 52.6, 52.1, 52.1, 30.5, 21.1; HRMS (Q-ToF MS ES+): m/z: (M+H)+ Calcd for C24H22BrO6: 485.0598. Found: 485.0600.
Synthesis of dibromide (15) via the DA reaction
In a sealed-tube were added 2,3-bis(bromomethyl)anthracene (12) (280 mg, 0.76 mmol) in dry toluene (3 mL) along with DMAD 24 (2.5 equiv). The reaction mixture was heated for 37 h (TLC monitoring). Evaporation of the solvent gave the crude product, which was further purified by column chromatography. Elution with 30% EtOAc/petroleum ether mixture afforded the desired product 15 as a white solid (350.7 mg, 90%). The compound formed showed identical 1H NMR data as obtained in earlier experiment.
Synthesis of bicyclic sultine (26)
To a suspension of rongalite 22 (152 mg, 0.98 mmol) in DMF (6 mL) was added dibromo compound 15 (50 mg, 0.24 mmol) and TBAB (31 mg, 0.096 mmol) at 0 ºC and the resulting suspension was stirred at 0 ºC for 3 h and at room temperature for additional 3 h. The reaction mixture was diluted with H2O (10 mL) (TLC monitoring) and extracted with CH2Cl2. The combined organic layer was washed with H2O (2 × 10 mL), dried over anhydrous Na2SO4. Evaporation of the organic layer under reduced pressure gave the crude product, which was purified by column chromatography. Elution of the column with 50% EtOAc/petroleum ether mixture afforded the product 26 (mixture of isomers) (31 mg, 76%) as a white semi solid.
Rf = 0.33 (silica gel, 30% EtOAc-petroleum ether); IR (Neat) 3056, 2986, 1718, 1436, 1218, 739 cm-1; 1H NMR (400 MHz, CDCl3) Isomers: δ 7.39-736 (m, 2H, ArH), 7.28 (s, 1H, ArH), 7.24 (s, 1H, ArH), 7.06-7.02 (m, 2H, ArH), 5.48 (s, 1H, ArCH), 5.48 (s, 1H, ArCH), 5.21 (dd, 1H, J1 = 12.1, J2 = 12.5 Hz), 4.89 (dd, 1H, J1 = 9.2, J2 = 9.1 Hz), 4.33 (dd, 1H, J1 = 8.9, J2 = 8.8 Hz), 3.78 (s, 6H, 2 × OCH3), 3.46 (dd, 1H, J1 = 2.8, J2 = 2.7 Hz); 13C NMR (100 MHz, CDCl3) Isomers: δ 165.8, 165.8, 165.7, 165.7 (C=O ester), 146.9, 146.8, 144.6, 144.5, 143.9, 143.9, 143.3, 143.2, 143.1, 143.1, 131.3, 131.0, 125.9, 125.9, 125.3, 125.2, 124.1, 124.0, 123.6, 123.2, 121.5, 121.4, 63.2, 63.2, 57.2, 56.8, 52.6, 52.1; HRMS (Q-ToF MS ES+): m/z: (M+H)+ Calcd for C22H19O6S: 411.0896. Found: 411.0902.
Synthesis of bicyclic sulphone (27)
In a 50 mL round bottom flask was added sultine 26 (20 mg) in toluene (3 mL) and then the reaction mixture was refluxed for 24 h (TLC monitoring). Evaporation of the solvent gave the crude product, which was further purified by column chromatography. Elution of the column with 40% EtOAc/petroleum ether mixture afforded the desired product 27 (15 mg, 73%) as a white semisolid.
Rf = 0.71 (silica gel, 40% EtOAc-petroleum ether-double run); IR (thin film) 3055, 2986, 2953, 1719, 1437, 1320, 1216, 738 cm-1; 1H NMR (400 MHz, CDCl3) δ 7.39-7.37 (m, 2H, ArH), 7.32 (s, 2H, ArH), 7.06-7.04 (m, 2H, ArH), 5.48 (s, 2H, ArCH), 4.29 (d, J = 15.7 Hz, 2H), 4.23 (d, J = 15.6 Hz, 2H) 3.79 (s, 6H, 2 × OCH3); 13C NMR (100 MHz, CDCl3) δ 165.7 (C=O ester). 146.6, 145.1, 142.9, 128.3, 126.0, 124.1, 121.7, 56.9, 52.7, 52.2; HRMS (Q-ToF MS ES+): m/z: (M+H)+ Calcd for C22H19O6S: 411.0892. Found: 411.0902.
Synthesis of indan-based AAA derivative (19)
To a suspension of the dibromide 15 (100 mg, 0.19 mmol), tetrabutylammonium hydrogen sulfate (32 mg, 0.095 mmol) and K2CO3 (262 mg, 1.9 mmol) in MeCN (15 mL) was added ethyl isocyanoacetate (23) (10 equiv). The reaction mixture was stirred under N2 at 75–80 °C for 15 h, then cooled to room temperature, and filtered through sintered glass funnel. The solid was washed with CH2Cl2 and the filtrate was evaporated in vacuo to afford the desired isonitrile product 28. The crude product obtained was directly subjected to hydrolysis without further purification.
The crude isonitrile derivative 28 was hydrolysed in absolute EtOH (15 mL) in the presence of conc. HCl (1 mL). The reaction mixture was stirred at room temperature for 3 h and then diluted with CH2Cl2 (50 mL), H2O (75 mL), and the mixture was made alkaline by slow addition of NaHCO3 with stirring. The separated CH2Cl2 layer was washed with H2O (2 × 25 mL), dried over Na2SO4, filtered, and evaporated under reduced pressure to give the crude amino product. The product obtained, which was directly subjected to acetylation. The crude product in dry MeCN (15 mL) was reacted with Ac2O (1.5 mL,) at room temperature for 24 h. Evaporation of the solvent gave the crude product, which was purified by column chromatography. Elution of the column with 50% EtOAc/petroleum ether mixture gave pure product 19 (12 mg, 13%, three-steps) as a pale yellow semi solid.
Rf = 0.31 (silica gel, 50% EtOAc-petroleum ether); IR (Neat) 3055, 2985, 2929, 1731, 1682, 1437, 1218, 1061, 740 cm-1; 1H NMR (400 MHz, CDCl3) δ 7.38-7.34 (m, 2H, ArH), 7.21 (s, 2H, ArH), 7.04-6.99 (m, 2H, ArH), 5.97 (s, 1H, NH), 5.42 (s, 2H, ArCH), 4.21 (q, 2H, J = 7.0 Hz, OCH2CH3), 3.78 (s, 6H, 2 × OCH3), 3.55 and 3.10 (d, 2H, J = 16.5 Hz, ArCH2, and d, 2H, J = 16.6 Hz, ArCH2), 1.83 (s, 3H, COCH3), 1.25 (t, 3H, J = 7.1 Hz, OCH2CH3); 13C NMR (100 MHz, CDCl3) δ 172.7 (C=O amide), 170.2, 166.0, (C=O ester), 147.2, 144.0, 143.5, 137.0, 125.6, 123.9, 120.5, 66.3, 61.8, 52.6, 52.5, 43.3, 29.8, 23.3, 14.2; HRMS (Q-ToF MS ES+): m/z: (M+H)+ Calcd for C28H28NO7: 490.1854. Found: 490.1866.
Synthesis of Tic-based AAA derivative (18)
To a suspension of the dibromide 15 (60 mg, 0.12 mmol) and K2CO3 (82 mg, 0.59 mmol) in MeCN (15 mL) was added diethyl acetamidomalonate (DEAM) 29 (26 mg, 0.12). The reaction mixture was stirred under N2 at 75–80 °C for 15 h, then cooled to room temperature, and filtered through sintered glass. The solid was washed with CH2Cl2 and the filtrate was evaporated under reduced pressure. The crude product obtained was purified by column chromatography. Elution of the column with 50% EtOAc/petroleum ether mixture gave the pure product 18 (51 mg, 76%) as a white semi solid.
Rf = 0.46 (silica gel, 40% EtOAc-petroleum ether); IR (Neat) 3058, 2984, 2954, 1732, 1682, 1436, 1394, 1232, 1060, 737 cm-1; 1H NMR (400 MHz, CDCl3) δ 7.37-7.34 (m, 2H, ArH), 7.18 (s, 2H, ArH), 7.04-7.00 (m, 2H, ArH), 5.43 (s, 1H, ArCH), 5.42 (s, 1H, ArCH), 4.61 (d, 2H, J = 4.6 Hz, ArCH2), 4.15 (q, 2H, J = 7.1 Hz, OCH2CH3), 4.04 (q, 2H, J = 7.2 Hz, OCH2CH3), 3.78 (s, 3H, OCH3), 3.77 (s, 3H, OCH3), 3.36 (d, 2H, J = 4.9 Hz, ArCH2), 2.24 (s, 3H, COCH3), 1.12 (t, 3H, J = 7.1 Hz, OCH2CH3), 0.94 (t, 3H, J = 7.2 Hz, OCH2CH3); 13C NMR (100 MHz, CDCl3) δ 171.0 (C=O amide), 167.9, 165.9, 165.8 (C=O ester), 147.1, 146.6, 143.7, 143.4, 129.8, 129.6, 125.7, 123.9, 123.5, 121,9, 68.0, 62.1, 62.0, 52.6, 52.2, 52.1, 47.9, 37.3, 22.5, 13.9, 13.7; HRMS (Q-ToF MS ES+): m/z: (M+H)+ Calcd for C31H32NO9: 562.2087. Found: 562.2077.
Synthesis of tetralin-based AAA derivative (17)
To a solution of bicyclic sultine 26 (29 mg, 0.07 mmol) in toluene (3 mL) was added methyl 2-acetamidoacrylate (4) (15 mg, 0.07 mmol). The resulting reaction mixture was refluxed for 3 days (TLC monitoring). Evaporation of the solvent gave the crude mixture, which was further purified by column chromatography. Elution of the column with 50% EtOAc/petroleum ether mixture afforded the corresponding sulphone 26 (8.7 mg, 30% yield) and further elution with 70% mixture of EtOAc and petroleum ether afforded the desired product 17 (semi solid, 8.4 mg, 25%) as mixture of isomers.
Rf = 0.28 (silica gel, 40% EtOAc-petroleum ether); IR (Neat) 3273, 3054, 2986, 2953, 1735, 1684, 1437, 1220, 1060, 739 cm-1; 1H NMR (400 MHz, CDCl3) Isomers: δ 7.39-7.35 (m, 2 × 2H, ArH), 7.14 (s, 2 × 1H, ArH), 7.07 (s, 2 × 1H, ArH), 7.05-7.01 (m, 2 × 2H, ArH), 5.63, 5.52 (s, 2 × 1H, 2NH), 5.41, 5.40, (s, 2 × 2H, bridge head, 2 × CH), 3.79, 3.79 (s, 2 × 3H, 2 × OCH3), 3.78, 3.78 (s, 2 × 3H, 2 × OCH3), 3.75, 3.74 (s, 2 × 3H, 2 × OCH3), 3.17 (t, 2 × 1H, J = 16.9 Hz, CH2), 2.88-2.81 (m, 2 × 1H, CH2), 2.79-2.60 (m, 2 × 2H, CH2), 2.58-2.51 (m, 2 × 1H, CH2), 2.05-2.01 (m, 2 × 1H, CH2), 1.95, 1.85 (s, 2 × 3H, 2 × COCH3); 13C NMR (100 MHz, CDCl3) Isomers: δ 174.0 (NHCO), 170.3, 170.2 (2 × CO2Me), 166.2, 166.1, 166.0, 166.0, 147.2, 147.0, 146.9, 143.9, 143.6, 143.6, 142.4, 142.3, 141.9, 141.8, 132.1, 132.1, 128.7, 125.7, 125.7, 125.0, 124.5, 124.5, 123.9, 57.8, 57.8, 52.8, 52.7, 52.6, 52.6, 52.0, 52.0, 38.1, 38.1, 27.6, 27.6, 25.2, 23.2, 23.2; HRMS (Q-ToF MS ES+): m/z: (M+H)+ Calcd for C28H28NO7: 490.1869. Found: 490.1866.
ACKNOWLEDGEMENTS
We thank CSIR and Department of Science and Technology (DST), New Delhi for the financial support. SK thanks DST for the award of a J. C. Bose fellowship. MM thanks CSIR for the award of a research fellowship.
References
1. a) P. Balaram, Curr. Opin. Struct. Biol., 1992, 2, 845; CrossRef b) C. T. Mbofana and S. J. Miller, J. Am. Chem. Soc., 2014, 136, 3285. CrossRef
2. a) T. Abellan, B. Manccheno, C. Najera, and J. M. Sansano, Tetrahedron, 2001, 57, 6627; CrossRef b) M. A. Blaskovich, Handbook on Synthesis of Amino Acids, Oxford University Press, 2010; c) K.-H. Park and M. J. Kurt, Tetrahedron, 2002, 58, 8629. CrossRef
3. S. E. Gibson, N. Guillo, and M. J. Yozer, Tetrahedron, 1999, 55, 585. CrossRef
4. G. L. Grunewald, S. H. Kuttab, M. A. Pleiss, and J. B. Mangold, J. Med. Chem., 1980, 23, 754. CrossRef
5. K. Kitahonoki and Y. Takano, Tetrahedron Lett., 1963, 4, 1597. CrossRef
6. a) S. Kotha, A. K. Ghosh, and M. Behera, Indian J. Chem., 2002, 41B, 2330; b) T. G. M. Dhar, H.-Y. Xiao, and B. Yang, U. S. Patent 8,013,004, September 6, 2011; c) B. V. Yang, U. S. Patent 7,326,728, February 5, 2008; d) D. S. Weinstein, J. Sheppeck, and J. L. Gilmore, U. S. Patent 7,605,264, October 20, 2009; e) J. Duan, B. Jiang, J. Sheppeck, and J. L. Gilmore, U. S. Patent 7,569,689, August 4, 2009; f) T. G. M. Dhar, H.-Y. Xiao, and B. Yang, U. S. Patent 7,642,273, January 5, 2010; g) D. S. Weinstein, J. L. Gilmore, J. Sheppeck, B. V. Yang, S.-H. Kim, and W. Vaccaro, U. S. Patent 7,253,283, August 7, 2007; h) B. V. Yang, U. S. Patent 7,273,881, September 25, 2007; i) D. S. Weinstein, J. L. Gilmore, J. Sheppeck, B. V. Yang, S.-H. Kim, and W. Vaccaro, U. S. Patent 7,544,808, June 9, 2009.
7. B. V. Yang and L. M. Doweyko, Tetrahedron Lett., 2005, 46, 2857. CrossRef
8. S. Kotha, G. Thota, and A. K. Ghosh, Bioorg. Med. Chem. Lett., 2000, 10, 1755. CrossRef
9. S. Kotha and A. K. Ghosh, Tetrahedron, 2004, 60, 10833. CrossRef
10. M. D. Hoey and D. C. Dittmer, J. Org. Chem., 1991, 56, 1947. CrossRef
11. W. F. Jarvis, M. D. Hoey, A. L. Finocchio, and D. C. Dittmer, J. Org. Chem., 1988, 53, 5750. CrossRef
12. R. L. Funk and K. P. C. Vollhardt, Chem. Soc. Rev., 1980, 9, 41. CrossRef
13. O. Diels and K. Alder, Justus Liebigs Ann. Chem., 1928, 460, 98. CrossRef
14. O. Diels and K. Alder, Justus Liebigs Ann. Chem., 1931, 486, 191. CrossRef
15. W. Carruthers, Cycloaddition Reactions in Organic Synthesis, Tetrahedron Organic Chemistry Series Vol. 8, Pergamon Press, 1990.
16. F. Fringuelli and A. Taticchi, the Diels–Alder Reaction, Selected Practical Methods, John Wiley & Sons, Ltd, England, 2002.
17. J. C. C. Atherton and S. Jones, Tetrahedron, 2003, 59, 9039. CrossRef
18. A. Anantanarayan and H. Hart, J. Org. Chem., 1991 56, 991. CrossRef
19. C.-C. Chi, I-F. Pai, and W.-S. Chung, Tetrahedron, 2004, 60, 10869. CrossRef
20. A. Satrijo, S. E. Kooi, and T. M. Swager, Macromolecules, 2007, 40, 8833. CrossRef
21. S. Kotha, E. Brahmachary, and N. Sreenivasachary, Tetrahedron Lett., 1998, 39, 4095. CrossRef
22. S. Kotha and E. Brahmachary, J. Org. Chem., 2000, 65, 1359. CrossRef
23. S. Kotha, Acc. Chem. Res., 2003, 36, 342. CrossRef
24. S. Kotha, N. Sreenivasachary, and E. Brahmachary, Eur. J. Org. Chem., 2001, 787. CrossRef
25. S. Kotha and A. K. Ghosh, Synthesis, 2004, 558. CrossRef
26. A. K. Mailyan, I. M. Krylov, C. Bruneau, P. H. Dixneuf, and S. N. Osipov, Eur. J. Org. Chem., 2013, 5353. CrossRef
27. S. Kotha and E. Brahmachary, Bioorg. Med. Chem. Lett., 1997, 7, 2719. CrossRef
28. J. L. Hallman and R. A. Bartsch, J. Org. Chem., 1991, 56, 6243. CrossRef
29. J.-F. Lohier, K. Wight, C. Peggion, F. Formaggio, C. Toniolo, M. Wakselman, and J.-P. Mazaleyrat, Tetrahedron, 2006, 62, 6203. CrossRef
30. J. V. B. Kanth and M. Periasamy, J. Org. Chem., 1991, 56, 5964. CrossRef
31. T. Fujisawa, T. Mori, and T. Sato, Chem. Lett., 1983, 835. CrossRef
32. N. M. Yoon, C. S. Pak, H. C. Brown, S. Krishnamurthy, and T. P. Stocky, J. Org. Chem., 1973, 38, 2786. CrossRef
33. N. M. Yoon and Y. S. Gyoung, J. Org. Chem., 1985, 50, 2443. CrossRef
34. G. D. Haytman and L. M. Weinstock, Org. Synth., Coll. Vol. VI, 1988, 620.
35. S. Kotha and S. Halder, Synlett, 2010, 337. CrossRef
36. P. K. Amancha, Y.-C. Lai, I.-C. Chen, H.-J. Liu, and J.-L. Zhu, Tetrahedron, 2010, 66, 871.
37. W. R. Roush, A. I. Ko, and H. R. Gillis, J. Org. Chem., 1980, 45, 4267. CrossRef
38. C. P. Benard, Z. Geng, M. A. Heuft, K. VanCrey, and A. G. Fallis, J. Org. Chem., 2007, 72, 7229. CrossRef
39. C. G. Neochoritis, T. Zarganes-Tzitzikas, and J. Stephanidou-Stephanatou, Synthesis, 2014, 46, 537. CrossRef