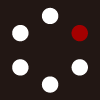
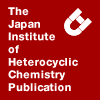
HETEROCYCLES
An International Journal for Reviews and Communications in Heterocyclic ChemistryWeb Edition ISSN: 1881-0942
Published online by The Japan Institute of Heterocyclic Chemistry
e-Journal
Full Text HTML
Received, 26th May, 2014, Accepted, 9th June, 2014, Published online, 11th June, 2014.
DOI: 10.3987/COM-14-S(K)33
■ Bis(diarylethenyl)thiophene, -bithiophene, and -terthiophene: A New Series of Violene-Cyanine Hybrid-Type Electron Donors
Yusuke Ishigaki, Hidetoshi Kawai, Ryo Katoono, Kenshu Fujiwara, and Takanori Suzuki*
Division of Chemistry, Graduate School of Science, Hokkaido University, Kita 10 Nishi 8, Kita-ku, Sapporo, Hokkaido 060-0810, Japan
Abstract
The title fluorescent (oligo)thiophenes 1-3 were designed as new violene/cyanine-hybrid-type electron donors, which have two 4-methoxyphenyl groups each on the two cyanine parts. Voltammetric analyses showed that they all undergo reversible two-stage one-electron oxidation. The intramolecular short S(thiophene) -- Cipso(Ar) contacts were commonly present in their X-ray structures, and the coplanarily extended geometries are suitable for π-delocalization. Electrochemical oxidation of 1-3 caused continuous changes in their UV-Vis-NIR and fluorescence spectra although the oxidized species are too labile to be isolated.Tetraarylethylenes 41,2 and their vinylogues such as 1,1,4,4-tetraarylbutadienes 52 are important components of violene/cyanine-hybrid-type2,3 electrochromic systems4 (Scheme 1), the characteristics of which can be altered by changing the Ar2C end-groups (cyanine-parts). π-Extension of the violene-part is another method for modifying the properties, but simple vinylogues5 are less attractive due to their instability against oxygen and proton. Thus, there is a growing interest on the compounds, to which cyclic π-systems are inserted as the extended violene-part.6
Based on the above consideration and our recent success in constructing methyleneacridan-type redox systems with the (oligo)thiophene skeleton,7 the title violene/cyanine-hybrids were newly designed, in which one (1), two (2), or three (3) thiophene-2,5-diyl units are inserted between the two Ar2C=CH end-groups (Scheme 2). This report describes their preparation, X-ray structures, redox properties, and perturbation of the number of thiophene units on the physical/spectral properties of 1 - 3. Preliminary studies on their electrochromic behavior were also conducted.
By the Wittig-Horner reaction of 2,5-diformylthiophene with (4-MeOC6H4)2CHP(=O)(OMe)2/BuLi in THF, the new donor 1 was obtained in 92% yield. Similarly, 2 and 3 were prepared from diformylbithiophene and diformylterthiophene in respective yields of 96% and 91%.8 They were isolated as stable yellow-orange crystalline materials (Scheme 3).
The X-ray analyses9 on 1-3 revealed that the violene π−system consisting of thiophene unit(s) and the ethenyl groups is nearly planar, in which two neighboring thiophenes in 2,3 adopt either an anti or syn arrangement (Figure 1). For all of the diarylethenyl units in 2,3, while one of the 4-MeOC6H4 groups is more or less coplanar with the violene part [dihedral angle θ2: 19.4(1)-48.8(1)º], the other is rather perpendicular to the ethenyl group [θ1: 73.8(1) - 88.2(3)º] and shows a short interatomic contact [d: 2.94(1) - 3.12(1) Å] between the thiophene sulfur atom (S) and the ipso carbon (Cipso) of the aryl group (sum of the van der Waals radii: 3.50 Å) (Table 1). The observed short contacts are highly likely to be associated with electronic interaction between σ* (C-S) and π (Cipso), resulting in conformational stability for the coplanarily extended geometries, which are suitable for electronic delocalization over the extended π-systems.
According to the theoretical calculation (B3LYP/6-31G*) for 1-3, the geometry around the diarylethenyl units are similar to that found in the crystal (Table 1),10 which supports the notion described above. In the solid-state geometry of 1, slight deformation of the violene π−system is caused by steric repulsion between the two inner aryl groups, although the above-mentioned structural motif suitable for σ* (C-S) and π (Cipso) interaction still exists, which suggests that the S -- Cipso contacts are attractive in nature. The electron donating group on the benzene nucleus is not necessary for this interaction since 2,5-bis(2,2-diphenylethenyl)thiophene without methoxy substituents also adopts a geometry similar to 1.11
According to the voltammetric analyses of 1-3, they undergo reversible two-stage one-electron oxidation (Figure 2) as in tetrakis(4-methoxyphenyl)ethylene 4 (Scheme 1, n = 0, Ar = 4-MeOC6H4)1 and many other violene/cyanine-hybrids. The first (E1ox) and second (E2ox) oxidation potentials of 1 are +0.86 and +1.00 V vs SCE in CH2Cl2, respectively. Nearly identical values were obtained for bithiophene 2 (E1ox +0.84 V, E2ox +0.99 V) and terthiophene 3 (E1ox +0.84 V, E2ox +0.95 V). These behaviors are in accord with the theoretical calculation, which predicts that the HOMO levels for 1-3 are nearly the same (Figure 3).
Comparisons of the UV-Vis spectra of 1-3 show a considerable redshift for the first band with an increase in the number of thiophene units in the violene part [λmax/nm (log ε): 406 (4.59) for 1, 435 (4.72) for 2, and 453 (4.76) for 3, respectively, in CH2Cl2] (Figure 4). If we consider the large MO coefficients on the thiophene rings in LUMOs of 1-3 estimated by the DFT calculations (Figure 3), the observed redshift can be accounted for by a lowering of the LUMO level with an increase in the number of thiophene units in the violene part, which reflects the electron-accepting properties of longer oligothiophenes.12
These electron donors 1-3 are also fluorescent. Consistent with the absorption spectra, the fluorescence (FL) maximum of terthiophene 3 occurs at the longest wavelength [λem: 527 nm (Φf: 0.14) in CH2Cl2] among 1-3. The FL maxima for 1 [518 (0.01)] and 2 [518 (0.09)] are the same, and the larger Stokes shift and lower quantum efficiency for 1 can be accounted for by supposing larger conformational flexibility of the violene π−system due to the steric repulsion between the two inner aryl groups, which would consume the excitation energy through the motion of the subunits in the excited state.
A drastic change in color from yellow to green was observed upon the electrochemical oxidation of 1 in CH2Cl2. At the expense of the absorption at 406 nm, new bands gradually appeared at 714 and 1072 nm in the UV-Vis-NIR spectrum (Figure 5a). The presence of isosbestic points at 360 and 470 nm indicates the clean conversion of 1 to the oxidized species,13 although attempts to isolate the labile cationic state as a salt have thus far failed. When the same electrolysis was followed by FL spectroscopy, a continuous decrease in intensity was also observed (Figure 5b), suggesting that the oxidized state is non-fluorescent.
Similar color change accompanied by decrease in FL intensity14 was observed upon the electrochemical oxidation of 2,3, where the newly appearing band at 714 in 1 was redshifted to 766 and 816 in the cases of 2 and 3, respectively. When the electrode polarity was reversed, these bands in the long-wavelength region disappeared, but electrochemical reduction did not completely reproduce the full intensity of the starting UV-Vis spectrum. To achieve reversibility of the electrochromic behavior, the oxidized state should be more stabilized, namely, by replacing the CAr2+ unit with one with a greater pKR+ value.
The present results showed that bis(diarylethenyl)-type electron donors with an (oligo)thiophene unit could be a feasible and easily-accessible platform for constructing violene/cyanine-hybrid-type electron donors. The number of thiophene units can be used as a variable to modify and tune the spectral properites (UV-Vis-NIR, FL) as well as the LUMO-level without changing the HOMO-level. Thus, with a proper molecular design stabilizig the cationic states, it would be possible to develop a novel dual electrochromic systems, by which not only UV-Vis-NIR but also FL spectral changes are reversibly induced. Studies along these lines are now in progress.
ACKNOWLEDGEMENTS
This work was supported by Grant-in-Aid for Scientific Research on Innovative Areas: "Organic Synthesis Based on Reaction Integration" (No. 2105) from MEXT, Japan. T.S. thanks JSPS Grant-in-Aid for Challenging Exploratory Research on "Maximum Function on Minimum Skeleton (MFMS)" (No. 25620050). Y.I. thanks JSPS research fellowship for young scientists (22-648). This work was also supported by the Cooperative Research Program of “Network Joint Research Center for Materials and Devices”. We are grateful to Prof. Takanori Fukushima at Chemical Resources Laboratory, Tokyo Institute of Technology.
References
1. R. Rathore, S. V. Lindeman, A. S. Kumar, and J. K. Kochi, J. Am. Chem. Soc., 1998, 120, 6931; CrossRef T. Suzuki, H. Shiohara, M. Monobe, T. Sakimura, S. Tanaka, Y. Yamashita, and T. Miyashi, Angew. Chem., Int. Ed. Engl., 1992, 31, 455. CrossRef
2. S. Hünig, M. Kemmer, H. Wenner, F. Barbosa, G. Gescheidt, I. F. Perepichka, P. Bäuerle, A. Emge, and K. Peters, Chem. Eur. J., 2000, 6, 2618. CrossRef
3. S. Hünig, M. Kemmer, H. Wenner, I. F. Perepichka, P. Bäuerle, A. Emge, and G. Gescheidt, Chem. Eur. J., 1999, 5, 1969. CrossRef
4. P. M. S. Monk, R. J. Mortimer, and D. R. Rosseinsky, Electrochromism and Electrochromic Devices, Cambridge Univ. Press, Cambridge, 2007; CrossRef L. Beverina, G. A. Pagani, and M. Sassi, Chem. Commun., 2014, 50, 5413. CrossRef
5. P. Simon, M. Landl, and M. Breza, Dyes and Pigments, 1999, 43, 227. CrossRef
6. S. Ito, H. Inabe, N. Morita, and A. Tajiri, Eur. J. Org. Chem., 2004, 1774; CrossRef S. Ito, T. Iida, J. Kawakami, T. Okujima, and N. Morita, Eur. J. Org. Chem., 2009, 5355; CrossRef Y. Sakano, R. Katoono, K. Fujiwara, and T. Suzuki, Chem. Lett., 2014, 43, 1143. CrossRef
7. T. Suzuki, Y. Hoshiyama, K. Wada, Y. Ishigaki, Y. Miura, H. Kawai, R. Katoono, K. Fujiwara, and T. Fukushima, Chem. Lett., 2013, 42, 1004. CrossRef
8. Typical procedure: To a solution of dimethyl bis(4-methoxyphenyl)methylphosphonate (840 mg, 2.50 mmol) in 10 mL of dry THF was added BuLi in hexane (1.65 M, 1.50 mL, 2.48 mmol) dropwise over 5 min at –78 °C under Ar, and the mixture was stirred for 2 h. To the solution was added 2,5-thiophenedicarboxaldehyde (145 mg, 1.03 mmol) at –78 °C, and the mixture was gradually warmed up to 23 °C and stirred for 14 h. After diluted with water, the whole mixture was extracted with CH2Cl2. The combined organic layers were washed with water and brine, and dried over anhydrous Na2SO4. After filtration, solvent was concentrated under reduced pressure. The residue was purified by column chromatography on silica gel (toluene/hexane = 3) to give 1 (535 mg) as a yellow solid in 92% yield. Data for 1: mp 135-137 °C; 1H NMR (300 MHz, CDCl3): δ/ppm 7.17 (4H, brd, J = 9.0 Hz), 7.06 (4H, brd, J = 8.8 Hz), 6.92 (2H, s), 6.89 (4H, brd, J = 8.8 Hz), 6.79 (4H, brd, J = 9.0 Hz), 6.56 (2H, s), 3.90 (6H, s), 3.78 (6H, s); 13C NMR (100 MHz, CDCl3): δ/ppm 159.32, 159.02, 141.57, 139.16, 135.23, 131.61, 131.36, 128.09, 127.71, 119.22, 114.38, 113.56, 55.28, 55.13; IR (KBr): ν/cm−1 3000, 2962, 2932, 2903, 2834, 1606, 1571, 1510, 1460, 1438, 1416, 1376, 1294, 1247, 1173, 1109, 1033, 884, 830, 808, 791, 738, 590, 569, 545, 520; LR-MS (FD): m/z (%) 562 (15), 561 (41), 560 (M+, bp).
From the corresponding dialdehydes, new donors 2 and 3 were similarly prepared. Data for 2: mp 209-211 °C; 1H NMR (300 MHz, CDCl3): δ/ppm 7.23 (4H, brd, J = 9.0 Hz), 7.16 (4H, brd, J = 8.8 Hz), 7.04 (2H, s), 6.98 (4H, brd, J = 8.8 Hz), 6.82 (4H, brd, J = 9.0 Hz), 6.71 (4H, s), 3.89 (6H, s), 3.80 (6H, s); 13C NMR (100 MHz, CDCl3): δ/ppm 159.67, 159.14, 140.91, 139.26, 137.11, 134.89, 131.48, 129.04, 128.04, 122.79, 119.09, 114.68, 113.66, 55.30; IR (KBr): ν/cm−1 3003, 2953, 2931, 2908, 2835, 1604, 1571, 1509, 1460, 1439, 1418, 1409, 1367, 1285, 1248, 1178, 1171, 1106, 1031, 1011, 902, 877, 841, 835, 817, 786, 573, 537, 525; LR-MS (FD): m/z (%) 645 (8), 644 (21), 643 (45), 642 (M+, bp). Data for 3: m.p. 143-146 °C; 1H NMR (300 MHz, CDCl3): δ/ppm 7.24 (4H, brd, J = 8.9 Hz), 7.18 (4H, brd, J = 8.7 Hz), 7.07 (2H, s), 7.00 (4H, brd, J = 8.7 Hz), 6.88 (2H, d, J = 3.8 Hz), 6.82 (4H, brd, J = 8.9 Hz), 6.79 (2H, d, J = 3.8 Hz), 6.78 (2H, s), 3.89 (6H, s), 3.80 (6H, s); 13C NMR (100 MHz, CDCl3): δ/ppm 159.73, 159.17, 141.14, 139.48, 136.61, 136.17, 134.82, 131.50, 131.38, 129.21, 128.07, 123.96, 122.84, 119.06, 114.73, 113.66, 55.33, 55.30; IR (KBr): ν/cm−1 3063, 2994, 2954, 2929, 2903, 2833, 1605, 1571, 1509, 1462, 1439, 1416, 1373, 1286, 1245, 1178, 1173, 1107, 1062, 1032, 836, 831, 799, 786, 584; LR-MS (FD): m/z (%) 727 (12), 726 (38), 725 (60), 724 (M+, bp).
9. Crystal data of 1: MF C36H32O4S, FW 560.71, monoclinic C2/c, a = 29.71(3), b = 12.58(1), c = 19.55(2) Å, β = 124.702(5)°, V = 6007.4(8) Å3, ρ = (Z = 8) 1.240 g cm-3, T = 153 K, 6280 independent reflections (Rint = 0.075), R = 11.2% (1148 data with F > 2σF), CCDC 800033. Due to the small size of crystal available, quality of the the structural analysis is not high but just enough for comparing the structural features to those of 2,3. Crystal data of 2: MF C40H34O4S2, FW 642.83, monoclinic P21/c, a = 15.799(5), b = 9.795(3), c = 20.756(7) Å, β = 96.229(1)°, V = 3193.0(1) Å3, ρ = (Z = 4) 1.337 g cm-3, T = 153 K, 7199 independent reflections (Rint = 0.023), R = 3.2% (4955 data with F > 2σF), CCDC 800035. Crystal data of 3: MF C44H36O4S3, FW 724.95, orthorhombic P212121, a = 9.741(3), b = 15.251(4), c = 24.139(7) Å, V = 3585.9(1) Å3, ρ = (Z = 4) 1.343 g cm-3, T = 153 K, 4510 independent reflections (Rint = 0.074), R = 5.9% (1824 data with F > 2σF), CCDC 800037.
10. According to the molecular mechanics calculation under the force field of Amber*, the observed geometry was never obtained as a stable conformer.
11. M. Zeller, A. D. Hunter, R. Hoefnagels, H. J. Geise, and F. Blockhuys, Acta Cryst., 2005, E61, o4183.
12. N. Camaioni, G. Ridolfi, V. Fattori, L. Favaretto, and G. Barbarella, J. Mater. Chem., 2005, 15, 2220; CrossRef A. Wakamiya, D. Yamazaki, T. Nishinaga, T. Kitagawa, and K. Komatsu, J. Org. Chem., 2003, 68, 8305. CrossRef
13. By considering large separation between E1ox and E2ox, the oxidized species was most probable to be the corresponding cation radical.
14. A. P. de Silva, H. Q. N. Gunaratne, and C. P. McCoy, Nature, 1993, 364, 42; CrossRef A. P. de Silva, H. Q. N. Gunaratne, and C. P. McCoy, J. Am. Chem. Soc., 1997, 119, 7891; CrossRef M. Inouye, K. Hashimoto, and K. Isagawa, J. Am. Chem. Soc., 1994, 116, 5517; CrossRef M. Irie, Chem. Rev., 2000, 100, 1685; CrossRef H. Li, J. O. Jeppesen, E. Levillain, and J. Becher, Chem. Commun., 2003, 846; CrossRef T. Suzuki, A. Migita, H. Higuchi, H. Kawai, K. Fujiwara, and T. Tsuji, Tetrahedron Lett., 2003, 44, 6837; CrossRef H. Higuchi, K. Ichioka, H. Kawai, K. Fujiwara, M. Ohkita, T. Tsuji, and T. Suzuki, Tetrahedron Lett., 2004, 45, 3027; CrossRef T. Suzuki, S. Tanaka, H. Higuchi, H. Kawai, and K. Fujiwara, Tetrahedron Lett., 2004, 45, 8563; CrossRef M. Dias, P. Hudhomme, E. Levillain, L. Perrin, Y. Sahin, F.-X. Sauvage, and C. Wartelle, Electrochem. Commun., 2004, 6, 325; CrossRef H. Röhr, C. Trieflinger, K. Rurack, and J. Daub, Chem. Eur. J., 2006, 12, 689; CrossRef R. O. Al-Kaysi, J. L. Bourdelande, I. Gallardo, G. Guirado, and J. Hernando, Chem. Eur. J., 2007, 13, 7066; CrossRef F. Miomandre, R. Meallet-Renault, J.-J. Vachon, R. B. Pansu, and P. Audebert, Chem. Commun., 2008, 1913; T. Suzuki, Y. Ishigaki, T. Iwai, H. Kawai, K. Fujiwara, H. Ikeda, Y. Kano, and K. Mizuno, Chem. Eur. J., 2009, 15, 9434; CrossRef T. Suzuki, Y. Tokimizu, Y. Sakano, R. Katoono, K. Fujiwara, S. Naoe, N. Fujii, and H. Ohno, Chem. Lett., 2013, 42, 1001; CrossRef T. Suzuki, Y. Kuroda, K. Wada, Y. Sakano, R. Katoono, K. Fujiwara, F. Kakiuchi, and T. Fukushima, Chem. Lett., 2014, 43, 887; CrossRef T. Suzuki, Y. Sakano, Y. Tokimizu, Y. Miura, R. Katoono, K. Fujiwara, N. Yoshioka, N. Fujii, and H. Ohno, Chem. Asian J., 2014, 9, 1841. CrossRef