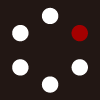
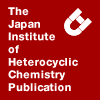
HETEROCYCLES
An International Journal for Reviews and Communications in Heterocyclic ChemistryWeb Edition ISSN: 1881-0942
Published online by The Japan Institute of Heterocyclic Chemistry
e-Journal
Full Text HTML
Received, 17th June, 2014, Accepted, 29th July, 2014, Published online, 31st July, 2014.
■ Efficient Installation and Elaboration of C4-C6 fused Furan Moiety in the Total Synthesis of Teucrium Clerodane Diterpenoids
Yen-Ku Wu, I-Chia Chen, Jia-Liang Zhu,* and Hsing-Jang Liu*
Department of Chemistry, National Dong Hwa University, 1, Sec. 2, Hsueh Rd., Shou-Feng, Hualien 974, Taiwan, R.O.C.
Abstract
We previously reported the total synthesis of several Teucrium clerodane diterpenoids including teucvin (1), 12-epi-teucvin (2), montanin A (3) and teuscorolide (4) in a unified Diels-Alder approach. A full account for the tactical installation of the C4-C6 furan ring of 3 from an α,β-unsaturated lactone moiety, and the elaboration of the resulting furan unit into an α,β-unsaturated γ-hydroxyl lactone for preparing 4 is discussed herein. In addition, the transformation of 3 and its 12-epimer into 1 and 2 by the air-mediated furan oxidation reaction is described.INTRODUCTION
Clerodane diterpenoids1 are a large family of natural products with over one thousand members having been isolated in the past decades. They have attracted considerable attention from synthetic community2 owing to their challenging structural complexity and interesting biological properties.2d,3 It has been known that the most abundant source of clerodanes including some 19-nor variants is the plants of genus Teucrium (family Lamiaceae),4 and the isolated individuals usually contain a decalin core bearing a C-9 (clerodane numbering) spiro γ-lactone ring with a furyl appendage (Figure 1). As compared with other classes of clerodane products, much less attention has been paid to the synthesis of Teucrium clerodanes in spite of their intriguing structural features and useful bioactivities2b,5 This fact may arise from the lack of efficient routes for reaching the compact and densely functionalized tricyclo-spiro ring system in correct steric sense. Among few documented examples, Lee and co-worker recently reported the first total synthesis of (−)-teucvidin,6 by employing a Michael/Conia-ene cascade cyclization reaction to establish the cis-decalin framework. In addition, Ley et al. described an approach for assembly of the spiro γ-lactone clerodane skeleton in a modified Jung's Diels-Alder strategy,7 but without achieving any natural targets.
Back to 2003,8 we reported the first total synthesis of (±)-teucvin (1), an amoebicidal agent,4a and its naturally occurring 12-epimer 2 as an application of our long-time pursued Diels-Alder strategy within α-activated cycloalkenone systems.9 In the sequence, a completely regio-, stereo- and facial selective Diels-Alder reaction between an α-formyl cyclohexenone and trans-2,4-pentadien-1-ol was utilized to assembly the decalin core with establishing two essential contiguous stereocenters (C-9 and C-10) (Scheme 1). It turned out that the hemiacetal ring concomitantly formed during the addition, after being protected in the acetate form, could ensure a stereo-controlled installation of the C-17 methyl group in the subsequent 1,4-addition reaction. Treatment of the resulting adduct with aqueous NaOH caused the acetyl deprotection and the selective hydrolysis of the primary ester at C-9. After this, the hydrogenation followed by the consecutive construction of the fused α,β-unsaturated lactone and the spiro γ-lactone completed the total synthesis of 1 and 2.
In an alternative route, the 1,4-addition adduct, by reacting with sodium ethoxide, was converted to a keto alcohol with maintaining both ester groups at C-9 (Scheme 2). The resulting compound was then transferred into lactone 5 in three steps. With 5 in hand, we initially tried to selectively reduce the primary ester into an aldehyde for installing the requisite spiro lactone in 1 and 2. However, the α,β-unsaturated lactone was found to be easily reduced, in producing a fused C4-C6 furan ring after in situ elimination. This finding has resulted in the synthesis of another natural target, montanin A (3),4b as we reported in the subsequent communication.10 Additionally, by taking the advantage of the liberty of the furan under the chromium oxidation conditions, a straightforward synthesis of teuscorolide (4)4d from 3 was achieved.10 Herein, we wish to disclose a full account of these elegant elaborations, as well as directly transferring 3 and its 12-epimer into 1 and 2 via an air-mediated furan oxidation reaction.
RESULTS AND DISCUSSION
A few semi-syntheses of 3 from other Teucrium clerodanes have been previously reported.11 In these cases, the assembly of the C4-C6 furan ring was a pillar but none of them was accomplished from a lactone. When 5 was subjected to the reduction with Dibal-H in THF at -40 ºC,12 the reaction offered three products 6-8 in varying yields depending on the amount of the employed agent. From the results outlined in Scheme 3, it can be seen that the lactone was reduced prior to the ester groups due to the least congested nature of the carbonyl group (reactivity: lactone>primary ester>tertiary ester). In the event, after the C=O double bond was attacked by the hydride from the less hindered side, the resulting aluminum complex would immediately undergo a β-elimination to yield the furan (Scheme 4).
Of three products (6, 7 and 8), compound 7 was recognized as a competent intermediate to 3 because of conceivable ease of introducing the furyl-appended spiro lactone. To this end, we intended to first oxidize the hydroxyl group of 7 into an aldehyde. However, this expected oxidation turned out to be highly formidable. We first attempted Dess-Martin, Swern13 and N-iodosuccinimide (NIS)14 oxidative conditions on 7 (Table 1, entries 1-3), but these reactions merely gave the poor formation of the desired intermediate 9 (entry 1) or complex mixtures (entries 2 and 3). When pyridinium chlorochromate (PCC) or pyridinium dichromate (PDC) was employed, an unexpected furan ring-opening was encountered to afford keto aldehyde 10 in 10 to 95% yields (entries 4-6). The results in entry 4 suggest that this ring-opening process should be inevitable even with using molar equivalent of chromium oxidant. After considerable experiments, we found that 9 could be obtained in high yields utilizing Fetizon's reagent15 in refluxing benzene (entry 7). Also, use of RuCl2(PPh3)316 as the oxidant offered 9 in comparable yields (entry 8). Treatment of 9 with 3-furyllithium led to a mixture of diastereomers 11 and 11' (ca. 1:1). These easily separated alcohols were individually subjected to lithium hydride-induced intramolecular transesterification furnishing (±)-montanin A (3) and its 12-epimer (3'),17 respectively (Scheme 5).
The opening of the furan ring by chromium oxidants is probably resulted from the cycloaddition between the furan diene moiety and the Cr(VI)=O double bond as we proposed before.10 On the other hand, the almost quantitative formation of 10 under the conditions of PDC (4 equiv)/CH2Cl2/rt (Table 1, entry 6) provoked our interest to elaborate 3 into montanin B, a natural product isolated from the same source as 3.4b When 3 was exposed to the same reaction conditions, the desired keto aldehyde 12 was produced in good yield (Scheme 6). In this reaction, the C-12 furyl appendage was not affected, and the chemoselective ring-opening is presumably attributed to the strong tendency to release the rigidity of the fused tricycle and/or the favorable electron-rich nature of the C4-C6 furan to attend the cycloaddition with chromate. To our surprise, reduction of 12 by NaBH4 did not afford montanin B, but led back to 3 as a result of recyclization and dehydration. Moreover, the use of the more powerful LiAlH4 (4 equiv) in THF also afforded 3 exclusively (80%). Thus, it can be concluded that once the aldehyde was reduced, the resulting hydroxyl group would spontaneously participate in an intramolecular cyclization with the C-6 keto moiety before further reduction of the ketone can occur.
Failing to the reduction, we then turned our attention to oxidizing the aldehyde into a carboxylic acid. This operation is for constructing a γ-hydroxyl α,β-unsaturated lactone through the incorporation with the C-6 keto group, to potentially allow the elaboration of 3 into teuscorolide (4). The use of PDC in DMF (Corey-Schmidt Method),18 a well-known procedure for directly transferring primary alcohols into acids, seemed to be a choice for our purpose. Diester 6 in lieu of 3 was first selected as the model substrate to simplify our analysis of possible products. We were pleased to find that under the action of PDC in DMF, the anticipated furan ring-opening, the aldehyde oxidation and the lactonization could be realized in one-pot to afford compound 13 as a single diasteromer (Scheme 7). The stereochemistry of the γ position of the lactone was established by the X-ray analysis (Figure 2). Notably, the reaction conduced in CH2Cl2 only yielded keto aldehyde 14, thus validating the key role that DMF played on the oxidation. Furthermore, the dehydration of 13 with p-toluenesulfonic acid in refluxing benzene led to the generation of compound 15 possessing the diene structural motif found in 4 (Scheme 8).
With the two-step procedure on our model system assured, we turned to the preparation of teuscorolide (4) from 3. In the event, the critical Corey-Schmidt oxidation of 3 with PDC in DMF proceeded with complete chemoselectivity giving a single diastereomer 1619 (Scheme 9). Upon exposure to the dehydrating conditions in Scheme 8, 16 was cleanly transformed to teuscorolide (4). Meanwhile, the same sequence was readily translated to the synthesis of 12-epi-teuscorolide (4')17 from 3' (3'→16'19→4').
The auto-oxidation of furan by molecular oxygen is a well-known approach for preparing unsaturated carbonyl derivatives.20 In light of this, we also accomplished the direct conversion of montanin A (3) and its 12-epimer (3') into teucvin (1) and 12-epi-teucvin (2). The reaction was carried out by stirring 1 or 2 under balloon pressure of air in CHCl3 at rt for 3 days, to lead to the generation of 14b or 2 in 82% or 76% yield (Scheme 10). According to literature reports,20 we envisioned that the reaction should be initiated by the cycloaddition of molecular oxygen with the diene of the furan to give a peroxide intermediate. Following this, a radical-mediated process involving the homolysis of the C-O bond, the radical abstraction from CHCl321 followed by the formation of C=O bond is assumed to produce the unsaturated lactone. The reaction proceeded with the complete diastereoselectivity regarding the stereochemistry at C-6, and again, the electron-rich nature of the C4-C6 fused furan may account for the observed chemoselectivity.
CONCLUSION
The furan-based transformations are quite useful in organic synthesis, and this realization has been well demonstrated by the results from our total synthetic studies on Teucrium clerodanes. In which, the efficient construction of the fused C4-C6 furan via the reductive elimination of the α,β-unsaturated lactone has led to the total synthesis of montanin A and 12-epi-montanin A. In addition, the extremely liberal character of the very furan ring under the Corey-Schmidt and air oxidative conditions has allowed the straightforward synthesis of teuscorolide, 12-epi-teuscorolide, teucvin and 12-epi-teucvin from montanin A and 12-epi-montanin A. As such, a unified synthetic route to 19-nor clerodane diterpenoids has been established.
EXPERIMENTAL
Unless otherwise stated, all of the reagents were obtained from commercial suppliers and used without further purification. Tetrahydrofuran (THF) was distilled from sodium-benzophenone, and benzene and dimethyl formamide (DMF) were distilled from calcium hydride before use. TLC analysis was carried out on Merck 25 DCAlufolien Kieselgel 60F254 aluminum-backed plates visualized by using UV light, or by means of an ethanolic solution of vanillin (5%) with sulphuric acid (5%). All of the products were purified by flash chromatography using Merck Art.9385 Kiesegel 60 silica gel (230-400 mesh). NMR spectra (1H, 13C) were recorded on a Brücker 400 spectrometer using deuteriochloroform (CDCl3) as solvent. Chemical shifts measurements are reported in delta (δ) units. Splitting patterns are described as singlet (s), doublet (d), triplet (t), quartet (q) or multiplet (m). Coupling constants (J) are reported in Hertz (Hz). Infrared (IR) spectra were recorded on an IR-FT JASCO 410 spectrophotometer (neat) and resonances are reported in wave numbers (cm-1). High resolution mass spectra (HRMS) were determined by using a A. E. I. model MS-50 mass spectrometer in fast atom bombardment (FAB) mode. Synthesis and spectral data for 3, 4, 5, 6, 7, 9, 10, 11, 11' and 16 are found in the electronic supplementary information accompanying with reference 10 in different designated numbers.
(6R*,7R*,8S*)-7-(Hydroxymethyl)-7-(hydroxyethyl)-6-methyl-3-oxatricyclo[6.3.1.04,12]dodec-1,4-diene (8)
To a solution of compound 5 (232 mg, 0.66 mmol) in THF (5 mL) at −40 °C under an atmosphere of nitrogen was added Dibal-H (1.0 M in hexane, 3.3 mmol, 3.3 mL) slowly. The resulting solution was stirred at −40 °C for 25 min, quenched with aqueous 6N HCl carefully to reach pH = 2, and the mixture was allowed to warm to room temperature. The aqueous layer was extracted with Et2O (2 x 10 mL). The combined organic extracts were washed with water (2 x 10 mL) and brine (30 mL), dried over anhydrous magnesium sulfate, filtered and concentrated. The crude residue was purified with flash chromatography on silica gel (EtOAc:hexanes = 1:10) to provide readily separable compounds 7 (93mg, 48%) and 8 (73 mg, 44%).
8: IR 3387 cm-1. 1H NMR (CDCl3, 400 MHz): δ 7.00 (s, 1H), 3.83 (t, J = 7.4 Hz, 2H), 3.50 (s, 2H), 2.62 (m, 2H), 2.51 (br d, J = 12.2 Hz, 1H), 2.40-2.29 (m, 2H), 2.05-1.95 (m, 2H), 1.88 (m, 4H), 1.25-1.21 (m, 2H), 1.12 (d, J = 6.9 Hz, 3H). 13C-NMR (CDCl3, 100 MHz): δ 146.8 (C), 135.9 (CH), 120.7 (C), 119.4 (C), 62.7 (CH2), 59.0 (CH2), 42.1 (C), 39.6 (CH), 37.1 (CH), 34.2 (CH2), 30.1 (CH2), 25.1 (CH2), 24.4 (CH2), 19.4 (CH2), 16.7 (CH3). HRMS (EI) calcd. for C15H23O3 [M+1]+: 251.1647, found: 251.1655.
Generation of 9 using RuCl2(PPh3)3 (Table 1, entry 8): To a solution of 7 (48 mg, 0.16 mmol) in benzene (8 mL) at room temperature under nitrogen was added RuCl2(PPh3)3 (90 mg, 0.24 mmol). The resulting mixture was stirred for 4 days, filtered with celite, and the solvent was removed from the filtrate to provide the crude product. The crude residue was purified with flash chromatography on silica gel (EtOAc:hexanes = 1:10) to provide compound 9 (40.5 mg) in 85% yield.
12-epi-Montanin A (3'): To a solution of compound 11' (12 mg, 0.03 mmol) in THF (5 mL) at room temperature under nitrogen was added quickly lithium hydride (0.3 mg, 0.04 mmol). The resulting mixture was heated to reflux for 2 h, and allowed to cool to room temperature. The reaction was quenched with water (3 mL) and the aqueous layer was separated and extracted with Et2O (2 x 3 mL). The combined organic layers were washed with brine (6 mL), dried over anhydrous magnesium sulfate and the solvents were removed. The crude product thus obtained was placed onto flash chromatography column and eluted (EtOAc:hexane = 1:15), giving 3' (9 mg, 86%) as a colorless liquid.
IR (film) 1762 cm-1. 1H NMR (CDCl3, 400 MHz): δ 7.45 (m, 1H), 7.42 (m, 1H), 7.05 (s, 1H), 6.41-6.38 (m, 1H), 5.36 (t, J = 8.6 Hz, 1H), 2.78-2.54 (m, 5H), 2.49-2.20 (m, 4H), 2.07-1.90 (m, 3H), 1.27 (d, J = 8.8 Hz, 3H). 13C NMR (CDCl3, 100 MHz): δ 175.5 (C), 147.9 (C), 144.3 (CH), 144.1 (CH), 139.6 (CH), 136.2 (CH), 125.5 (C), 119.7 (C), 117.0 (C), 108.1 (CH), 71.6 (CH), 50.7 (C), 39.7 (CH2), 39.1 (CH), 30.0 (CH3), 25.6 (CH2), 23.9 (CH2), 19.1 (CH2), 17.7 (CH3). HRMS (EI) calcd. for C19H20O4: 312.1362, found: 312.1357.
(1R*,4’R*,5S*,6R*,7R*,10R*)-1-Formyl-7-methylbicyclo[4.4.0]-decan-9-one-6-spiro-1’-[4’-(3-furyl)-3’-oxacyclopetan-2’-one] (12): To a solution of 3 (15 mg, 0.048 mmol) in CH2Cl2 (4 mL) at room temperature was added PDC (73 mg, 0.19 mmol) under a nitrogen atmosphere. The resulting mixture was stirred for 4 h, filtered with celite, and the solvent was removed from the filtrate to provide the crude product. Purification with flash chromatography on silica gel (EtOAc:hexane = 1:5) gave the compound 12 (13 mg, 80%) as a colorless oil.
IR 1760 cm-1. 1H NMR (CDCl3, 400 MHz): δ 9.63 (s, 1H), 7.46 (d, J = 0.6 Hz, 1H), 7.44 (d, J = 1.7 Hz, 1H), 6.39 (d, J = 0.9 Hz, 1H), 5.47 (t, J = 8.6 Hz, 1H), 3.18 (dd, J = 15.9, 13.1 Hz, 1H), 2.78-2.70 (m, 2H), 2.67 (br, 1H), 2.60-2.48 (m, 4H), 2.30-2.12 (m, 4H), 1.09 (d, J = 6.6 Hz, 3H). 13C NMR (CDCl3, 100 MHz): δ 200.3 (C), 192.2 (CH), 176.1 (C), 147.8 (C), 146.0 (C), 144.3 (CH), 139.6 (CH), 124.7 (C), 107.9 (C), 72.3 (CH), 52.8 (C), 46.8 (CH), 45.6 (CH2), 39.2 (CH2), 37.3 (CH), 25.1 (CH2), 23.3 (CH2), 20.3 (CH2), 17.6 (CH3). HRMS (FAB) calcd. for C19H21O5 [M+1]+: 329.1389, found: 329.1389.
(4S*,6R*,7R*,8S*)-7-Carbethoxy-7-(carbethoxymethyl)-6-methyl-4-hydroxy-3-oxatricyclo[6.3.1.04,12]dodec-1-en-2-one (13): To a solution of compound 6 (37 mg, 0.11 mmol) in DMF (2 mL) at room temperature was added PDC (165 mg, 0.44 mmol) under a nitrogen atmosphere. The resulting mixture was stirred for 4 h, filtered with celite, and the solvent was removed from the filtrate to provide the crude product. Purification with flash chromatography on silica gel (EtOAc:hexane = 1:2) gave the compound 13 (23 mg, 58%) as a white powder, which was recrystallized from hexane to yield a crystalline solid suitable for X-ray analysis.
IR 3462, 1729 cm-1. 1H NMR (CDCl3, 400 MHz): δ 4.08 (q, J = 7.0 Hz, 2H), 4.00 (q, J = 7.1 Hz, 2H), 3.18 (t, J = 7.7 Hz, 1H), 2.92 (d, J = 14.9 Hz, 1H), 2.60 (d, J = 14.9 Hz, 1H), 2.50-1.80 (m, 7H), 1.46 (qt, J = 10.3, 0.2 Hz, 1H), 1.20 (t, J = 7.1 Hz, 3H), 1.11 (t, J = 7.1 Hz, 3H), 1.11-0.92 (m, 2H), 0.89 (d, J = 6.9 Hz, 3H). 13C NMR (CDCl3, 100 MHz): δ 171.4 (C), 171.3 (CH), 171.2 (C), 162.7 (C), 126.5 (C), 102.3 (C), 60.9 (CH2), 60.7 (CH2), 55.2 (C), 40.7 (CH2), 36.1 (CH2), 35.2 (CH), 33.2 (CH), 23.7 (CH2), 21.5 (CH2), 19.3 (CH2), 16.1 (CH3), 14.0 (CH3), 13.9 (CH3). HRMS (FAB) calcd. for C19H27O7 [M+1]+: 367.1757, found: 367.1757.
(4R*,5R*,6S*)-5-Carbethoxy-5-(carbethoxymethyl)-10-formyl-4-methylbicyclo[4.4.0]dec-10-en-2-one(14): To a solution of compound 6 (38 mg, 0.11 mmol) in CH2Cl2 (8 mL) at room temperature was added PDC (124 mg, 0.33 mmol) under a nitrogen atmosphere. The resulting mixture was stirred for 4 h, filtered with celite, and the solvent was removed from the filtrate to provide the crude product. Purification with flash chromatography on silica gel (EtOAc:hexane = 1:5) gave the compound 14 (37 mg, 95%) as a colorless oil.
1H NMR (CDCl3, 400 MHz): δ 9.64 (s, 1H), 4.18-3.97 (m, 4H), 2.99-2.91 (m, 2H), 2.84 (d, J = 13.5 Hz, 1H), 2.76-2.72 (m, 3H), 2.63 (d, J = 13.2 Hz, 1H), 2.39 (dd, J = 12.5, 2.0 Hz, 1H), 2.04-1.95 (m, 1H), 1.91 (dd, J = 11.1, 2.8 Hz, 1H), 1.73 (td, J = 7.9, 2.6 Hz, 2H), 1.24 (t, J = 5.8 Hz, 3H), 1.20 (t, J = 5.6 Hz, 3H), 1.05 (d, J = 5.8 Hz, 3H). 13C NMR (CDCl3, 100 MHz): δ 200.5, 192.6, 173.2, 170.6, 148.5, 145.6, 61.0, 60.7, 51.1, 46.3, 41.0, 39.7, 35.5, 23.9, 23.4, 20.7, 16.1, 14.0, 13.9. HRMS (EI) calcd. for C19H26O6: 350.1729, found:350.1729.
(4S*,6R*,7R*,8S*)-7-Carbethoxy-7-(carbethoxymethyl)-6-methyl-3-oxatricyclo[6.3.1.04,12]dodec-1,3-dien-2-one (15): To a solution of p-toluenesulfonic acid (35 mg, 0.21mmol) in benzene (5 mL) at room temperature was added the solution of compound 13 (80 mg, 0.22 mmol) in benzene (2 mL) under nitrogen. The resulting mixture was then heated to reflux for 15 min, allowed to cool to room temperature, diluted with CH2Cl2 (3 mL), and quenched with dilute aqueous sodium bicarbonate (3 mL). The aqueous layer was separated and extracted with CH2Cl2 (3 x 3 mL), and the combined organic layers was washed with brine, dried over anhydrous magnesium sulfate. Filtration, concentration, and purification with flash chromatography on silica gel (EtOAc:hexane = 1:1) gave the desired product 15 (48 mg, 98%) as a colorless liquid.
1H NMR (CDCl3, 400 MHz): δ 5.21 (d, J = 2.0 Hz, 1H), 4.12 (qd, J = 5.7, 1.5 Hz, 2H), 4.08-3.95 (m, 2H), 3.14-3.07 (m, 2H), 2.91 (d, J = 12.3 Hz, 1H), 2.75 (d, J = 12.3 Hz, 1H), 2.37 (dd, J = 14.4, 3.9 Hz, 1H), 2.20-2.12 (m, 1H), 2.08 (dd, J = 8.9, 3.8 Hz, 2H), 1.66-1.57 (m, 1H), 1.24 (t, J = 5.7 Hz, 3H), 1.13 (d, J = 6.6 Hz, 3H), 1.09 (t, J = 5.7 Hz, 3H), 0.8-0.7 (m, 1H). 13C NMR (CDCl3, 100 MHz): δ 171.0 (C), 170.9 (C), 169.8 (C), 153.4 (C), 147.4 (C), 121.9 (C), 108.7 (CH), 60.8 (CH2), 60.7 (CH2), 54.2 (C), 37.0 (CH), 35.6 (CH2), 35.3 (CH), 29.0 (CH2), 22.4 (CH2), 19.3 (CH2), 16.3 (CH3), 14.1 (CH3), 13.9 (CH3). HRMS (EI) calcd. for C19H24O6: 348.1573, found: 348.1572.
(4’R*,6R*,7R*,8S*)-6-Methyl-4-hydroxy-3-oxatricyclo[6.3.1.04,12]dodec-1-en-2-one-7-spiro-1’-[4’-(3-furyl)-3’-oxacyclopetan-2’-one] (16'): To a solution of compound 3' (4 mg, 0.012 mmol) in DMF (2 mL) at room temperature was added PDC (18 mg, 0.048 mmol) under a nitrogen atmosphere. The resulting mixture was stirred for 4 h, filtered with celite, and the solvent was removed from the filtrate to provide the crude product. Purification with flash chromatography on silica gel (EtOAc:hexane = 1:2) gave the compound 16' (2 mg, 38%) as a colorless oil.
IR 3347, 1764 cm-1. 1H NMR (CDCl3, 400 MHz): δ 7.45 (s, 1H), 7.43 (d, J = 1.7 Hz, 1H), 6.38 (d, J = 0.9 Hz, 1H), 5.36 (t, J = 8.5 Hz, 1H), 2.70 (tt, J = 3.0, 2.96 Hz, 1H), 2.62 (dd, J = 14.0, 7.4 Hz, 1H), 2.40 (dd, J = 13.9, 7.4 Hz, 1H), 2.35-2.25 (m, 2H), 2.22 (t, J = 12.6 Hz, 3H), 2.18-2.04 (m, 3H), 2.00-1.78 (m, 2H), 1.16 (d, J = 6.9 Hz, 3H). 13C NMR (CDCl3, 100 MHz): δ 175.6 (C), 170.6 (C), 159.9 (C), 144.3 (CH), 139.6 (CH), 128.7 (C), 125.0 (C), 107.9 (CH), 102.0 (C), 71.9 (CH), 54.3 (C), 40.3 (CH2), 40.0 (CH), 38.2 (CH2), 37.9 (CH), 29.6 (CH2), 24.2 (CH2), 21.6 (CH2), 14.1 (CH3). HRMS (FAB) calcd. for C19H21O6 [M+1]+: 345.1338, found:345.1337.
12-epi-Teuscorolide (4'): To a solution of p-toluenesulfonic acid (2 mg, 0.009 mmol) in benzene (2 mL) at room temperature was added the solution of compound 16' (2 mg, 0.006 mmol) in benzene (5 mL) under nitrogen. The resulting mixture was then heated to reflux for 15 min, allowed to cool to room temperature, diluted with CH2Cl2 (3 mL), and quenched with dilute aqueous sodium bicarbonate (1 mL). The aqueous layer was separated and extracted with CH2Cl2 (3 x 3 mL), and the combined organic layers were washed with brine, dried over anhydrous magnesium sulfate. Filtration, concentration, and purification with flash chromatography on silica gel (EtOAc:hexane = 1:1) gave the desired product 4' (2 mg, 99%) as a colorless liquid.
IR 1763, 1708 cm-1. 1H NMR (CDCl3, 400 MHz): δ 7.45 (s, 1H), 7.44 (d, J = 1.6 Hz, 1H), 6.37 (t, J = 0.6 Hz, 1H), 5.40 (t, J = 8.4 Hz, 1H), 5.23 (d, J = 1.5 Hz, ,1H), 2.89 (t, J = 6.7 Hz, 1H), 2.73-2.67 (m, 1H), 2.59 (dd, J = 14.0, 8.4 Hz, 1H), 2.43 (dd, J = 14.0, 8.4 Hz, 1H), 2.37 (d, J = 6.0 Hz, 1H), 2.35-2.20 (m, 1H), 2.20-2.10 (m, 1H), 2.02 (dq, J = 12.0, 3.6 Hz, 1H), 1.70-1.50 (m, 2H), 1.33 (d, J = 7.4 Hz, 3H). 13C NMR (CDCl3, 100 MHz): δ 174.7, 169.6, 150.9, 148.0, 144.3, 139.6, 125.2, 124.5, 107.9, 107.3, 71.6, 53.5, 40.1, 39.4, 39.4, 23.5, 22.4, 19.4, 17.2. HRMS (FAB) calcd. for C19H19O5 [M+H]+: 327.1232, found: 327.1229.
Synthesis of Teucvin (1) from Montanin (3): The solution of 3 (18 mg, 0.056 mmol) in CHCl3 (2 mL) was stirred at room temperature under an air atmosphere for 3 days. The solvent was removed then the residue was purified by flash chromatography giving teucvin (1) (15 mg, 82%).
1H NMR (CDCl3, 400 MHz): δ 7.44 (m, 2H), 6.37(m, 1H), 5.43 (t, J = 8.5 Hz, 1H), 4.74-4.73 (m, 1H), 2.67-2.62 (m, 1H), 2.53 (d, J = 8.6 Hz, 2H), 2.13-2.25 (m, 5H), 2.02-1.99 (m, 3H), 1.92-1.86 (m, 1H), 1.05 (d, J = 6.8 Hz, 3H). 13C NMR (CDCl3, 100 MHz): δ 175.9 (C), 173.0 (C), 161.3 (C), 144.3 (CH), 139.5 (CH), 126.2 (C), 125.0 (C), 108.0 (CH), 78.2 (CH), 71.7 (CH), 53.5 (C), 42.1 (CH), 40.9 (CH2), 36.0 (CH), 35.2 (CH2), 24.8 (CH2), 21.7 (CH2), 19.6 (CH2), 17.0 (CH3). HRMS (EI) calcd. for C19H20O5: 328.1311, found: 328.1308.
Synthesis of 12-epi-Teucvin (2) from 12-epi-Montanin A (3'): The solution of 3' (12 mg, 0.039 mmol) in CHCl3 (2 mL) was stirred at room temperature under an air atmosphere for 3 days. The solvent was removed then the residue was purified by flash chromatography giving 12-epi-teucvin (2) (10 mg, 82%).
1H NMR (CDCl3, 400 MHz) δ 7.44 (m, 2H), 6.40 (m, 1H), 5.37 (t, J = 8.6 Hz, 1H), 4.76-4.71 (m, 1H), 2.60 (dd, J = 14.0, 8.1 Hz, 1H), 2.57-2.54 (m, 1H), 2.40 (dd, J = 14.0, 9.1 Hz, 1H), 2.30-2.24 (m, 1H), 2.18-1.92 (m, 7H), 1.42-1.32 (m, 1H), 1.20 (d, J = 6.6 Hz, 3H). 13C NMR (CDCl3, 100 MHz): δ 175.4 (C), 173.0 (C), 161.5 (C), 144.3 (CH), 139.7 (CH), 126.9 (C), 125.0 (C), 107.9 (CH), 78.3 (CH), 71.9 (CH), 53.8 (C), 40.7 (CH2), 40.1 (CH), 38.2 (CH), 35.5 (CH2), 24.3 (CH2), 21.9 (CH2), 19.6 (CH2), 16.8 (CH3). HRMS (EI) calcd. for C19H20O5: 328.1311, found: 328.1305.
ACKNOWLEDGEMENTS
We are grateful to the National Science Council of Taiwan and National Tsing Hua University for financial support, and Mr. Yun-Sheng, Wen from Institute of Chemistry of Academia Sinica of Taiwan for the X-ray analysis.
References
1. (a) A. T. Merritt and S. V. Ley, Nat. Prod. Rep., 1992, 9, 243; CrossRef (b) B. Rodriguez, M. C. de la Torre, A. Perales, P. Y. Malakov, G. Y. Papanov, M. S. J. Simmonds, and W. M. Blaney, Tetrahedron, 1994, 50, 5451. CrossRef
2. For examples on total synthesis of clerodanes, see: see: a) P. S. Jones, S. V. Ley, N. S. Simpkins, and A. J. Whittle, Tetrahedron, 1986, 42, 6519; CrossRef b) E. Piers, M. L. Breau, Y. X. Han, G. L. Plourde, and W. L. Yeh, J. Chem. Soc., Perkin Trans. 1, 1995, 963; CrossRef c) H. Hagiwara, T. Nojima, Y. Suka, T. Hoshi, and T. Suzuki, Nat. Prod. Commun., 2011, 333; d) K. M. Lovell, K. M. Prevatt-Smith, A. Lozama, and T. E. Prisinzano, “Chemistry of Opioids, Topics in Current Chemistry”, Springer, vol. 299, 2011, pp. 141-185. CrossRef
3. (a) M. S. J. Simmonds, W. M. Blaney, S. V. Ley, M. Bruno, and G. Savonia, Phytochemistry, 1989, 28, 1069; CrossRef (b) R. D. Enriz, H. A. Baldoni, M. A. Zamora, E. A. Jáuregui, M. E. Sosa, C. E. Tonn, J. M. Luco, and M. Gordaliza, J. Agric. Food Chem., 2000, 48, 1384; CrossRef (c) S. F. Zhou, C. C. Xue, X. Q. Yu, and G. Wang, Curr. Drug Metab., 2007, 526; CrossRef (d) M. S. Stankovic, M. G. Curcic, J. B. Zizic, M. D. Topuzovic, S. R. Solujic, and S. D. Markovic, Int. J. Mol. Sci., 2011, 12, 4190. CrossRef
4. For examples, see: a) E. Fujita, I. Uchida, and T. Fujita, J. Chem. Soc., Chem. Commun., 1973, 793; CrossRef b) P. V. Malakov, G. Y. Papanov, and N. M. Mollov, Tetrahedron Lett., 1978, 23, 2025; CrossRef c) G. Savona, M. C. García-Alvarezt, and B. Rodríguez, Phytochemistry, 1982, 21, 721; CrossRef d) J. L. Marco, B. Rodriguez, G. Savona, and F. Piozzi, Phytochemistry, 1982, 21, 2567; CrossRef e) M. C. de la Torre, A. Maggio, and B. Rodíguez, Tetrahedron, 2000, 56, 8007; CrossRef f) M. Bruno, M. L. Bondi, S. Rosselli, A. Maggio, F. Piozzi, and N. A. Arnold, J. Nat. Prod., 2002, 65, 142; and references cited therein. CrossRef
5. a) M. S. J. Simmonds and W. M. Blaney, “Labiate-insect interactions: effects of Libiate-derived compounds on insects behavior. In Advances in Labiate Science”, R. M. Harley and T. Reynolds, Eds.; Royal Botanical Gardens: Kew, 1992, pp. 375-392; b) F. Ortego, B. Rodríguez, and P. Castañera, J. Chem. Ecol., 1995, 21, 1375. CrossRef
6. X. Liu and C.-S. Lee, Org. Lett., 2012, 14, 2886. CrossRef
7. a) A. T. Merritt, R. H. Pouwer, D. J. Williams, C. M. Williams, and S. V. Ley, Org. Biomol. Chem., 2011, 9, 4745; CrossRef b) M. E. Jung, C. N. Zimmerman, G. T. Lowen, and S. I. Khan, Tetrahedron Lett., 1993, 34, 4453. CrossRef
8. H. J. Liu, J. L. Zhu, I. C. Chen, R. Jankowska, Y. Han, and K. S. Shia, Angew. Chem. Int. Ed., 2003, 42, 1851. CrossRef
9. a) H. J. Liu, T. K. Ngooi, and E. N. C. Browne, Can. J. Chem., 1988, 66, 3143; CrossRef b) H. J. Liu, W. L. Yeh, and Y. C. Sew, Tetrahedron Lett., 1993, 34, 4435; CrossRef c) H. J. Liu and Y. Han, Tetrahedron Lett., 1993, 34, 423; CrossRef d) Y. K. Wu, T. W. Ly, and K. S. Shia, Curr. Org. Synth., 2010, 7, 78. CrossRef
10. I. C. Chen, Y. K. Wu, H. J. Liu, and J. L. Zhu, Chem. Commun., 2008, 4720. CrossRef
11. a) G. Savona, M. P. Paternostro, F. Piozzi, and B. Rodriguez, Tetrahedron Lett., 1979, 20, 379; CrossRef b) M. Bruno, C. Fazio, F. Pioozi, B. Rodriguez, and M. C. Torre, Phytochemistry, 1995, 40, 148.
12. Several reducing agents including Red-Al, Dibal-H, LiAlH4 and NaBH4 were attempted for the reduction, but only Dibal-H offered the cleanest reaction profile.
13. T. T. Tidwell, Synthesis, 1990, 857. CrossRef
14. S. Hanessian, D. H. Wong, and M. Therien, Synthesis, 1981, 394. CrossRef
15. M. Fétizon, M. Golfter, and P. Mourgues, Tetrahedron Lett., 1972, 17, 4445. CrossRef
16. H. Tomioka, K. Takai, K. Oshima, and H. Nozaki, Tetrahedron Lett., 1981, 22, 1605. CrossRef
17. The title compound has not been identified as a natural product yet.
18. E. J. Corey and G. Schmidt, Tetrahedron Lett., 1979, 52, 399. CrossRef
19. The stereochemistry at C-6 was tentatively assigned based on the structure of 13.
20. a) G. C. M. Lee, E. T. Syage, D. A. Harcourt, J. M. Holmes, and M. E. Garst, J. Org. Chem., 1991, 56, 7007; CrossRef b) L. A. Badovskaya and L. V. Povarova, Chem. Heterocycl. Compd., 2009, 45, 1023. CrossRef
21. S. Sathiyabalan and P. E. Hoggard, Inorg. Chem., 1995, 34, 4562. CrossRef