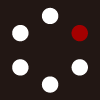
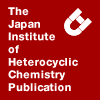
HETEROCYCLES
An International Journal for Reviews and Communications in Heterocyclic ChemistryWeb Edition ISSN: 1881-0942
Published online by The Japan Institute of Heterocyclic Chemistry
e-Journal
Full Text HTML
Received, 22nd October, 2014, Accepted, 5th February, 2015, Published online, 17th February, 2015.
DOI: 10.3987/REV-14-812
■ Chemistry of Macrocyclic β-Lactam: An Overveiw
Vijaya Bhaskar Vangala, Mahagundappa Rachappa Maddani, Rama Mohan Hindupur, and Hari Narayan Pati*
Department of Chemistry, Advinus Therapeutics Ltd., 21 and 22 Peenya Industrial Area, 560058, India
Abstract
β-Lactam macrocycles displayed major contributions in the filed of drug discovery. The chemical potentiality of β-lactam has proved their usefulness in the discovery of variety of conformationally restricted macrocycles. Macrocycles containing versatile functionalities derived from β-lactam have also been employed in the fields of supramolecular chemistry. In this review, attempts were made to summarize the recent methods for synthesis of potential β-lactam containing macrocycles.CONTENTS
1. Introduction
2. Macrocycles Containing β-Lactam
2.1 Synthesis of Macrocyclic β-Lactam Containing Enediynes
2.2 Synthesis of Macrocyclic bis-β-Lactams from Macrocyclic bis-Imines
2.3 RCM Approach for the Synthesis of Macrocyclic β-Lactams
2.4 Synthesis of Macrocyclic bis-β-Lactams Containing Calixarenes
3. Conclusion
1. INTRODUCTION
β-Lactams are heterocyclic systems of great importance and occupy a unique segment of chemical space. They exist as structural subunits in many products of interest as pharmaceuticals and have applications in synthetic chemistry.1 β-Lactam nucleus is the most fruitful pharmacophore in developing variety of therapeutic agents. The 1,3,4-trisubstituted derivatives of β-lactams have been utilized in various biological activities such as, antibacterial,2 potent cholesterol absorption inhibitors,3 human cytomegalovirus protease inhibitors,4 and thrombin inhibitors.5 The resistance developed by the microorganisms against the most traditional β-lactam antibiotic drugs raised the interest of organic chemists in exploring the development of novel β-lactam drugs to improve activity of β-lactams.6 As a consequence, a plethora of literature precedence is available on the compounds having β-lactam ring7 and the number of transformations in which the 2-azetidinone ring is involved.8 Ojima has shown the utility of bis-β-lactam for the synthesis9 of peptides. Later on, in 2012, these authors have explored the chemistry advances of β-lactam and its medicinal applications.10 The synthesis of bis-β-lactam in general has been reported by stepwise construction of β-lactam rings.11 Recently, β-lactam linked with an ethylene bridge has also been reported.12
A variety of synthetic methods for β-lactam are now available, and the topic has been reviewed on more than one occasion. In addition, remarkable advances in combining two effective bioactive agents in a single molecule as macrocyclic conjugates have been successfully growing in the medicinal field. For example, sultamicillin13 (1) a conjugate of two drugs with expected combined bioactivities and Ro 23-942414 (2) in which two antibiotics enhance each other through a mechanism: when a β-lactamase deactivates the cephalosporin by opening the β-lactam ring, the second antibacterial agent is released to exert its activity.15
The chemical diversity of macrocycles expanded significantly, supported by advances in bioinformatics and synthetic methodology. Macrocyclization is an efficient way to re-organize a peptidomimetic ligand in the β-turn conformation suitable for binding to the active site of serine, aspartyl, cysteine, and metalloproteases. Thus, macrocyclization is an excellent way to lock out alternative conformations that may lead to liabilities such as side effects or poor bioavailability. Macrocyclization also reduced overall polarity, enhanced cellular penetration and increased bioavailability. The outcome of macrocyclization in peptide chemistry is an efficient way to restrict conformation, reduce polarity, increase proteolytic stability, and consequently to improve the drug ability.16 Macrocycles supported the transition from non-drug like peptidic leads to druggable clinical candidates. On other enzymes, it was found to be an efficient way to fine-tune the selectivity profile.
Macrocycles have the unique ability to span large surface areas while remaining conformationally restricted compared to acyclic molecules of equivalent molecular weight. As a result, macrocycles are more conformationally restricted than their acyclic analogues, which potentially can disclose higher target binding, selectivity and improved oral bioavailability. This characteristic makes them especially appropriate for targets displaying shallow surfaces, which can prove to be quite challenging for acyclic small molecules. Appreciably, this structural motif has now been successfully tested on most biological target classes.17 However, the macrocycles containing β-lactam unit have not been studied frequently. In this context we have attempted to put into perspective the current applications, and opportunities associated with synthetic macrocycles containing β-lactam in drug discovery. Accordingly, this article is dedicated to the synthetic aspects of β-lactam macrocycles and highlights salient features of their medicinal chemistry and drug discovery aspects. This article is also aimed at providing the reader an appreciation of the structural diversity generated for β-lactam containing macrocycles.
2. MACROCYCLES CONTAINING β-LACTAM
2.1 Synthesis of macrocyclic β-lactam containing enediynes
The structures of naturally occurring enediynes have been established in the eighties.18 The enediynes antitumor antibiotics19 are a small family of natural compounds. The novel molecular architecture of these compounds was intriguing, since conjugated enediynes were considered to be rather unstable structures; hence they were expected not to be found in nature. Moreover, natural enediynes were found to be highly cytotoxic and characterized by a potent antitumor activity. Consequently, their unprecedented mode of action opened the avenue to the several research groups for the development of various artificial enediynes endowed with interesting biological properties. In this direction, Giuseppe Guanti20 and coworkers, successfully prepared several 10-membered macrocyclic enediynes class of compounds fused with a β-lactam ring as shown in the following Schemes 1–3. The commercially available 4-acetoxyazetidinone (3) was employed for double propargylation to obtain O-protected trans derivative (5) via intermediate monopropargylated compound (4). The p-methoxybenzyl groups were deprotected to get bis propargylic alcohol derivative, which was then subjected to Swern oxidation to result the bis-aldehyde compound (6). The compound 7 was obtained by vanadium complex catalyzed stereoselective reductive pinacol coupling reaction. The desired macrocyclic β-lactam derivative (8) was finally achieved by thionocarbonate formation and reduction with an activated phosphine in an overall 13% yield as shown in Scheme 1. Though this synthesis was relatively short and straightforward, the macrocyclic β-lactam obtained here in Scheme 1 was very stable. This β-lactam, was not only stable but did not undergo ring opening under physiological conditions. Therefore, authors decided to introduce an activating substituent or a triggering device in order to optimize activity to show any biological activity.
An alternative synthetic pathway was designed to have macrocyclic β-lactam with some additional functional groups.21 In this alternative strategy, the authors performed the assembly of the conjugated enediyne system first on an acyclic precursor, and then cyclized to obtain macrocyclic β-lactam as shown in the Scheme 2. The synthesis started with preparation of racemic mono substituted β-lactam 9 from racemic aspartic acid. The methylation of β-lactam nitrogen provided the stable compound to explore subsequent reactions. A stereoselective propargylation of β-lactam enolate was followed to get the compound 10. A selective removal of silyl group at alkyne was achieved with the help of silver nitrate to furnish the compound 11. The acyclic enediyne 12 was assembled via stereospecific Sonogashira reaction with 1-chloro-4-(trimethylsilyl)but-1-en-3-yne (Z). Probably, this preparation of acyclic enediyne is widely approached strategy in macrocyclic enediyne chemistry by further cyclization. Interestingly, it usually takes an advantage of the intramolecular addition of an acetylide anion onto an aldehyde. Hence, it was decided to have an aldehyde group by oxidizing a primary alcohol from the compound 13 which in turn was obtained by two steps from compound 12 as shown in the Scheme 2. Subsequently, oxidation of alcohol followed by Nozaki cyclization using chromous chloride furnished the desired macrocyclic β-lactam 14 in very good yields.
An almost similar approach22 was used to prepare another macrocyclic β-lactam having a removable silyl group on nitrogen atom as shown in Scheme 3. Removal of silyl group results the free amine functionality, which can be further elaborated as per the research planning. However, in this scheme, the β-lactam 16 was prepared more convergently by the use of a Staudinger condensation with 15, having a styryl group as synthetic equivalent of a hydroxymethyl group. As usual mono propargylation was carried out to give the compound 17. The removal of p-methoxybenzyl group on nitrogen atom was found to be troublesome at later stages of the synthesis; hence, its deprotection followed by protection with silyl group was performed in few steps at this stage to afford compound 18. The chemoselective ozonolysis of the double bond of 18 in the presence of the unprotected terminal triple bond was found to be successful, which is a key step in this synthesis to get the compound 19. Subsequent reactions were carried out according to the earlier synthesis via key intermediate 20, to obtain the desired macrocyclic β-lactam 21 in good yield.
During the investigation of these macrocyclic β-lactam enediynes, it was found that, these macrocycles were very much stable in the dry state (quite unusual for enediyne molecules) and did not give any cycloaromatization products even if heated at 100 °C. Interestingly, when the β-lactam ring was opened, a complete different behavior was observed, in fact cycloaromatization could be achieved at 37 °C even under physiological conditions.23 These activated macrocyclic β-lactam were therefore tested against plasmid DNA, giving encouraging results being able to bind the DNA strands. These compounds were able to provoke single and double strand break in plasmid DNA (the ratio between single and double break was about 15:1) with an overall activity about ten times higher than that of simple monocyclic enediynes. Similarly, several other macrocyclic β-lactam compounds were also successfully prepared.24
2.2 Synthesis of macrocyclic bis--lactam from macrocyclic bis-imines
In 2006, Raghunathan25 and coworkers reported the synthesis of a series of grafted macrocyclic bis-β-lactam derivatives by stereoselective [2+2] cycloadditions reactions in good yields. Authors achieved the incorporation of β-lactam in macrocyclic ring as shown in Schemes 4 and 5. Salicylaldehyde and α,α’-xylene dibromide 22 were condensed to get compounds 23, which were further treated with ethylene diamine to afford the corresponding bis-imines 24 in good yields. This synthesized bis-imine was further reacted with phenoxy acetyl chloride in presence of base in dichloromethane to afford the diastereomeric mixture of cis macrocyclic bis-β-lactam (25) in a 50% yield (diasteremeric ratio = 58:42). These diastereomeric mixtures were separated by column chromatography and confirmed by IR and NMR spectral analysis. The stereochemistries of 25 were determined by X-ray analysis.
The above methodology was extended for the synthesis of O-ethylene linked macrocyclic bis-β-lactam (27b and 27c) as shown in Scheme 5.
Recently, Sierra26 and coworkers designed and synthesized several macrocyclic β-lactam derivatives in order to use as efficient scaffolds to prepare highly functionalized macrocycles. In this context, very attractive macrocyclic bis-imines (30-33) were prepared by treatment of dialdehydes (26, 28 and 29) with 1,3-diaminopropane and 1,4-diamino-2-butyne as shown in the Scheme 6. In continuation, the 1:1 mixture of two isomeric β-lactams was prepared from the reaction of diimine 30 with phenoxyacetyl chloride in dry dichloromethane at -78 °C with 78% yield. The mixture was isolated by column chromatography to yield compounds 34 and 35 (Scheme 7), having a cis-cis stereochemistry in the β-lactam ring as deduced from the coupling constants using NMR spectroscopy. The coupling constants of H3-H4 and H3¢-H4¢ protons (J3, 4) 4.5 and 4.4 Hz for compounds 34 and 35, respectively.27 Therefore, the reaction yielded both the cis-syn-cis 34 and the cis-anti-cis 35 isomers. This cis-syn-cis stereochemistry was further confirmed by a single crystal X-ray diffraction analysis.
Similarly, reactions of imines 31-33 with phenoxyacetyl chloride under the Staudinger reaction conditions yielded products 36-38 in yields of 78%, 74%, and 45%, respectively. All the synthesized compounds 36-38 have the signal corresponding to the β-lactam H4 shielded δ 4.88, 4.88, and 5.38 ppm, respectively. This shielding was also observed in compound 35 (δH4 = 5.17) compared to compound 34 (δH4 = 5.41) from which the relative stereochemistry was further ascertained by X-ray analysis. Therefore, the cis-anti-cis stereochemistry was assigned to products 36-38 (Scheme 8).
It is well known that cyclic imines prefer to form the trans-β-lactam due to the fixed Z-configuration of the imine C=N bond forced by the cyclic structure.28 However, the E-configured29 macrocyclic imines 30-33 follow the usual pattern giving only the cis-β-lactam as observed for open-chain imines.30 More fascinating is the observed selectivity during the formation of the bis-β-lactam system. The imines 31-33 have a clear bias for the formation of cis-anti-cis isomers, while diimine 30, which forms the equimolecular amount of cis-syn-cis and cis-anti-cis isomers. These facts suggest a direct influence of one of the emerging four-membered rings on the torquo selectivity31 of the second ring closure, but only when both centers are not too close to each other as in compound 30, in which the linkage between the O-Ar groups is a CH2-CH2 chain. Moreover, the formation of 1:1 syn/anti diastereomeric mixtures has been also reported in the synthesis of acyclic cis-bis-β-lactam linked by an ethylene bridge.32 Hence, the origin of the selectivity in the formation of β-lactam and the influence of several factors (structural, electronic, reaction conditions) is still a subject of debate.
Similarly, multicomponent macrocyclizations including bifunctional building blocks (MiB) strategy, which has been shown to be quite useful for the preparation of a range of complex macrocyclics and has been exemplified with a number of different substrates and reaction modalities, including the generation of combinatorial libraries.33 In addition to the Ugi’s multi component reaction (MCR), the Passerini and Staudinger’s three-component reaction has been successfully applied in the MiB strategy as shown in the Scheme 9. Three components involved are pyridine dialdehyde, amino meta xylene derivative and methoxy acetyl chloride have been used in MiB strategy for the preparation of macrocycle containing multiple β-lactam units (39) from Staudinger process.
2.3 RCM Approach for the Synthesis of Macrocyclic β-Lactams
It is well known that, the more conventional method for the synthesis of macrocyclic β-lactams includes the synthesis of macrocyclic imines followed by the Staudinger [2 + 2] cycloaddition of suitable imine with appropriate ketenes. Interestingly, Ibrahim34 and coworkers were recently developed an efficient ring closure metathesis (RCM) approach for the synthesis of macrocyclic β-lactams. Authors have utilized RCM methodology towards the synthesis of macrocyclic aza-crown ethers fused with β-lactam via bridgehead (ring junction) nitrogen. The treatment of 1,2-bis-o-allyloxybenzylideneamines 40a,b with aryloxyacetyl chloride in dichloromethane in the presence of triethylamine gave a mixture of cis-anti-cis (racemic) 41a,b, 42a,b, and 43a,b and cis-syn-cis (meso) 44a,b, 45a,b, and 46a,b diastereomers as shown in the Scheme 10. The RCM reactions of the above mixtures 41-46 were successfully accomplished to give 57-98% yields of the corresponding β-lactam macrocycles 47-52 with Z/E mixtures using Grubbs’ catalyst 53 (5 mol%) as shown in Scheme 10. Interestingly, it was found that, the RCM of 41b-45b with Grubbs’ catalyst 54 (5 mol%) led to disappearance of the starting materials and formation of the corresponding 22– membered macrocyclic β-lactams 47b-52b in over 90% yield with only E stereochemistry. Authors prepared these macrocyclic β-lactams through the sequential application of Staudinger [2 + 2] ketene-imine
cycloaddition followed by RCM reactions in an overall 83% of yield, which is higher than the conventional methods. Therefore, Staudinger-RCM approach was a better yielding process for the preparation of macrocyclic β-lactams. These macrocyclic β-lactams are potential starting materials useful for further functionalization of macrocyclic aza-crown ethers.
2.4 Synthesis of macrocyclic bis--lactam containing calixarenes
The calixarene derivatives have often been employed in recent years as drug carriers in medicinal field due to their specific conformations. A very few reports are available devoting to the study of calixerene derivatives as active therapeutics agents against the treatment of various diseases.35 In this regard, a new kind of potentially therapeutically active macrocyclic calixarene derivatised β-lactam was synthesized and characterized by Regnouf-de-Vains36 as shown in the Scheme 11. Two penicillin derivatives have been grafted at the lower rim of the p-tert-butylcalix[4]arene, giving a new kind of podand which was fully characterized by IR, Mass and NMR analysis. The calixarene diacid 55 was activated using dicyclohexylcarbodiimide and N–hydroxysuccinimide to get the corresponding bis-activated ester 56 with 85% of yield. The tosylate salt of penicillin 57 was prepared, as described by Daehne37 et al., and Matlin37 et al. and Ogura38 et al. by reaction of pivaloyloxymethyl chloride and 6-aminopenicillanic acid thus giving a lipophilic pro-drug which should liberate the biologically active free acid after hydrolysis by esterases, as initially developed with the commercial pivmecillinam and pivampicillin. The mild controlled peptide bond condition39 was chosen in order to avoid probable degradation of the β-lactam ring. The last step consisted of the reaction of 56 and 57 in mild conditions, at room temperature under argon. The bis-penicillin podand 58 was obtained with purity (> 95%) after chromatography purification with 70% of yield.
Similarly in 2007, Rogalska40 and coworkers studied interaction of calixarene-β-lactam antibiotic derivatives with biological membranes. The study designed to evaluate the capacity of the three new amphiphilic p-tert-butylcalix-[4]arene derivatives to interact with biological membranes. 1,2-Dimyristoyl-sn-glycero-3-phosphoethanolamine (DMPE), a model bacterial membrane lipid, was used to prepare the monolayers. These conjugates were considered as possible drug carriers since, these releases the antibiotic by hydrolysis reaction in vitro and can be expected to take place in physiological conditions as well. In this context, authors prepared three macrocyclic derivatives of calixarene-β-lactam conjugates 59-61, which are shown in the Figure 1 below.
Calix 59 is the derivatives of N-acetyl-pivaloyloxymethyl-6-aminopenicillanic acid (6-pivAPA) grafted on the 1,3-bis-(O-acetyl)-p-tert-butylcalix[4]arene cone conformer.41 Similarly, Calix 60 is a derivative of benzylpenicillin (penicillin G or PG) ethyl ester and Calix 61 is benzylpenicillin (penicillin G or PG) propyl ester.42 The miscibility properties of Calix 59, are different from Calix 60 and Calix 61 due to aliphatic pivaloyl terminal moieties present in Calix 59, which decrease the molecule packing in the mixed films of biological membranes as compared to benzyl moieties present in Calix 60 and Calix 61. Decreased molecule packing in the case of Calix 59 is accompanied by an increased ordering of the DMPE molecules in the lipid-rich phase. On the other hand, the higher ∆Gex obtained with Calix 61 compared to Calix 60 indicates that a higher conformational flexibility of the former facilitates the interactions with DMPE. The results obtained suggest that the incorporation of Calix 60 and Calix 61 into biological membranes can be expected, whereas Calix 59 could be more easily trans located across the membrane compared to the benzylpenicillin derivatives.
3. CONCLUSION
Macrocycles have been exploited on most classes of pharmaceutical targets. Topologically, macrocycles can cover a broad surface area in a conformationally restricted way, as compared to acyclic small molecules of similar molecular weights. As a result, it seems that macrocyclization extends the acceptable range of molecular weight and polarity toward higher values. Macrocyclization offers two directions for diversity generation. First, it represents an efficient way to scan chemical space without adding molecular weight, which is often a problem with acyclic molecules. Examples where ring size and topology greatly affected biological activity and PK profile are numerous. Second, macrocycles can be used as templates for the restricted spatial display of pharmacophores. Macrocycles appear to have an advantage over their acyclic counterparts for those targets that require large interacting surface areas and/or potentially distant epitopes such as the shallow targets of protein-protein interactions.
This review report is an outcome of the attempts made towards the collection of recent advances in the synthesis of macrocyclic β-lactam derivatives. Synthetic macrocycles have already made significant contributions to drug discovery. Synthetic macrocyclic β-lactams represent the most recent subclass; and their chemical diversity will be limited only by our imagination. Potentially large libraries of macrocyclic β-lactams could be made using MiB approaches, and the chemistry proved to be rather versatile. The obtained macrocyclic β-lactams are potential starting materials for the synthesis of highly functionalized macrocycles through the chemical transformation of the β-lactam ring moiety. Such transformation of β-lactam ring into macrocyclic crown compounds containing suitable functionalities results potential applications in diverse fields of supramolecular chemistry.43 This includes the transformation to macrocyclic aza-crown ethers, macrocyclic bis-amides, and macrocyclic β-amino acids.26,44 The β-lactam antibiotics have occupied a central role in the fight against pathogenic bacteria and the subsequent rise in the quality of life for the world population as a whole.
ACKNOWLEDGEMENTS
The author Mr. Vijaya Bhaskar V. is thankful to Advinus Therapeutics Limited for providing all necessary facilities as a part of his Ph. D. program and Mangalore University, Karnataka for Ph. D. registration.
References
1. D. M. Smith, A. Kazi, L. Smith, T. E. Long, B. Heldreth, E. Turos, and Q. Ping dou, Mol. Pharmacol., 2002, 61, 1348; CrossRef N. De Kimpe, A. R. Katritzky, C. W. Rees, and E. F. V. Scriven, Comprehensive Heterocyclic Chemistry II, Eds. Pergamon Press: London, 1996, 8, pp. 507-512; H. Staudinger, Ann., 1907, 356, 51; T. T. Tidwell, Eur. J. Org. Chem., 2006, 563; CrossRef N. Fu and T. T. Tidwell, Tetrahedron, 2008, 64, 10465; CrossRef J. Xu, ARKIVOC, 2009, ix, 21; L. Jiao, Q. F. Zhang, Y. Liang, S. W. Zhang, and J. X. Xu, J. Org. Chem., 2006, 71, 815; CrossRef Y. Wang, Y. Liang, L. Jaoo, D.-M. Du, and J. X. Xu, J. Org. Chem., 2006, 71, 6983; CrossRef L. Jiao, Y. Liang, Q. Zhang, S. Zhang, and J. Xu, Synthesis, 2006, 659; L. Jiao, Y. Liang, and J. X. Xu, J. Am. Chem. Soc., 2006, 128, 6060; CrossRef Y. Liang, S. W. Zhang, and J. X. Xu, J. Org. Chem., 2005, 70, 334; CrossRef E. G. Lee, B. L. Hodous, E. Bergan, C. Shih, and G. C. Fu, J. Am. Chem. Soc., 2005, 127, 11586. CrossRef
2. I. Chaeuk, S. Maiti, R. Micetich, M. Daneshtalab, K. Atchison, O. A. Phillips, and C. Kunugita, J. Antibiot., 1994, 47, 1030. CrossRef
3. W. D. Vaccaro and H. R. Davis, Bioorg. Med. Chem. Lett., 1998, 8, 313; CrossRef W. D. Vaccaro, R. Sher, and H. R. Davis, Bioorg. Med. Chem. Lett., 1998, 8, 35; CrossRef D. A. Burnett, Tetrahedron Lett., 1994, 35, 7339. CrossRef
4. A. D. Borthwick, G. Weingarte, T. M. Haley, M. Tomaszewski, W. Wang, Z. Hu, J. Bedard, H. Jin, L. Yuen, and T. S. Mansour, Bioorg. Med. Chem. Lett., 1998, 8, 365. CrossRef
5. W. T. Han, A. K. Trehan, J. J. K. Wright, M. E. Federici, S. M. Seiler, and N. A. Meanwell, Bioorg. Med. Chem., 1995, 3, 1123. CrossRef
6. R. Ponsford and P. H. Bentley, Recent Advances in the Chemistry of Anti-infective Agents, Royal Society of Chemistry, Cambridge, 1993, pp. 79–92.
7. M. Gómez-Gallego, M. J. Mancheño, and M. A. Sierra, Tetrahedron, 2000, 56, 5743; CrossRef W. Dürckheimer, J. Blumbach, R. Lattrell, and K. H. Scheunemann, Angew. Chem., Int. Ed. Engl., 1985, 24, 180; CrossRef G. I. Georg, The Organic Chemistry of α-Lactam; VCH: New York, 1992.
8. N. Hatanaka, R. Abe, and I. Ojima, Chem. Lett., 1982, 445; CrossRef b) I. Ojima, Adv. Asymmetric Synth., 1995, 1, 95; CrossRef M. S. Manhas, D. R. Wagle, J. Chiang, and A. K. Bose, Heterocycles, 1988, 27, 1755; CrossRef C. Palomo, J. M. Aizpurua, I. Ganboa, and M. Oiarbide, Pure Appl. Chem., 2000, 72, 1763. CrossRef
9. I. Ojima, N. Hatanaka, N. Yoda, R. Abe, M. Yatabe, and M. Yanashita, In Peptide Chemistry, ed. by S. Sakakibara; Protein Research Foundation: Osaka, 1983; pp. 29–34; M. Yamashita, R. Abe, N. Hatanaka, and I. Ojima, In Peptide Chemistry, ed, by S. Sakakibara; Protein Research Foundation: Osaka, 1983; pp. 85–90.
10. A. B. Kamath and I. Ojima, Tetrahedron, 2012, 68, 10640. CrossRef
11. I. Ojima, N. Hatanaka, N. Yoda, R. Abe, M. Yatabe, and M. Yamashita, In Peptide Chemistry, ed. by S. Sakakibara; Protein Research Foundation: Osaka, 1983; pp. 29-34; M. Yamashita, R. Abe, N. Hatanaka, and I. Ojima, In Peptide Chemistry, ed. by S. Sakakibara; Protein Research Foundation: Osaka, 1983; pp. 85-90; I. Ojima, M. Zhao, T. Yamato, K. Nakahashi, M. Yamashita, and R. Abe, J. Org. Chem., 1991, 56, 5263; CrossRef I. Ojima, K. Nakahashi, S. M. Branstadter, and N. Hatanaka, J. Am. Chem. Soc., 1987, 109, 1798; CrossRef A. K. Bose, J. F. Womensdors, L. Krishnan, Z. Urbanczyk-Lipkowska, D. C. Shelly, and M. S. Manhas, Tetrahedron, 1991, 47, 5379; CrossRef M. Jayaram, V. G. Puranik, and B. M. Bhawal, Tetrahedron, 1996, 52, 9005. CrossRef
12. K. Karupaiyan, V. G. Puranik, A. R. A. S. Deshmukh, and B. M. Bhawal, Tetrahedron, 2000, 56, 8555. CrossRef
13. H. A. Friedel, Drugs, 1997, 37, 491. CrossRef
14. S. L. Dax, Antibacter. Chemother. Agents, 1997, 74; C. H. O’Callagham, R. B. Sykes, and S. E. Staniford, Antimicrob. Agents Chemother., 1976, 10, 245. CrossRef
15. D. D. Keith, H. A. Albrecht, G. Beskid, K. K. Chan, J. G. Christenson, R. Cleeland, K. Deitcher, M. Delaney, N. H. Georgopapadakou, F. Konzelmann, M. Okebe, D. Pruess, P. Rossman, A. Specian, R. Then, C. C. Wei, and M. Weigle, Rec. Adv. Chem. Anti-infective Agents, 1994, 79.
16. C. Adessi and C. Soto, Curr. Med. Chem., 2002, 9, 963; CrossRef C. Gilon, D. Halle, M. Chorev, Z. Selinger, and G. Byk, Biopolymers, 1991, 31, 745; CrossRef R. T. Borchardt, A. Jeffrey, T. J. Siahaan, S. Gangwar, and G. M. Pauletti, Adv. Drug Delivery Rev., 1997, 27, 235; CrossRef ; CrossRef R. P. McGeary and D. P. Fairlie, Curr. Opin. Drug Discovery Dev., 1998, 1, 208.
17. W. Brandt, V. J. Haupt, and L. A. Wessjohann, Curr. Top. Med. Chem., 2010, 10, 1361. CrossRef
18. K. Edo, M. Mizugaki, Y. Koide, H. Seto, K. Furihata, N. Otake, and N. Ishida, Tetrahedron Lett., 1985, 26, 331; CrossRef J. S. Thorson, E. L. Sievers, J. Ahlert, E. Shepard, R. E. Whitwam, K. C. Onwueme, and M. Ruppen, Curr. Pharm. Design, 2000, 6, 1841. CrossRef
19. K. C. Nicolaou, Angew. Chem., Int. Ed. Engl., 1991, 32, 1387; CrossRef A. L. Smith and K. C. Nicolaou, J. Med. Chem., 1996, 39, 2103. CrossRef
20. L. Banfi, A. Basso, G. Guanti, and R. Riva, ARKIVOC, 2006, vii, 261. CrossRef
21. L. Banfi, A. Basso, and G. Guanti, Tetrahedron, 1997, 53, 3249. CrossRef
22. L. Banfi and G. Guanti, Tetrahedron Lett., 2002, 43, 7427. CrossRef
23. L. Banfi and G. Guanti, In Italian Patent, 2002.
24. L. Banfi and G. Guanti, Eur. J. Org. Chem., 1998, 1543. CrossRef
25. N. Arumugam and R. Raghunathan, Tetrahedron Lett., 2006, 47, 8855. CrossRef
26. M. A. Sierra, D. Pellico, M. G. Gallego, M. Mancheño, and R. Torres, J. Org. Chem., 2006, 71, 8787. CrossRef
27. M. S. Manhas, M. Ghosh, and A. K. Bose, J. Org. Chem., 1990, 55, 575; CrossRef A. K. Sharma, S. N. Mazumdar, and M. P. Mahajan, J. Org. Chem., 1996, 61, 5506; CrossRef R. Alcázar, P. Ramírez, R. Vicente, M. J. Mancheño, M. A. Sierra, and M. G. Gallego, Heterocycles, 2001, 55, 511. CrossRef
28. L. Jiao, Y. Liang, and J. Xu, J. Am. Chem. Soc., 2006, 128, 6060. CrossRef
29. P. Leoni, E. Grilli, M. Pasquali, and M. Tomassini, J. Chem. Soc., Dalton Trans., 1985, 2561; CrossRef . CrossRef
30. S. Dumas and L. S. Hegedus, J. Org. Chem., 1994, 59, 4967; CrossRef L. S. Hegedus, J. Montgomery, Y. Narukawa, and D. C. Sanustad, J. Am. Chem. Soc., 1991, 113, 5784. CrossRef
31. F. P. Cossio, A. Arrieta, B. Lecea, and J. M. Ugaldby, J. Am. Chem. Soc., 1994, 116, 2085. CrossRef
32. K. Karupaiyan, V. G. Puranik, A. R. A. S. Deshmukh, and B. M. Bhawal, Tetrahedron, 2000, 56, 8555. CrossRef
33. L. A. Wessjohann, D. G. Rivera, and O. E. Vercillo, Chem. Rev., 2009, 109, 796. CrossRef
34. Y. A. Ibrahim, T. F. Al-Azemi, M. D. Abd El-Halim, and E. John, J. Org. Chem., 2009, 74, 4305. CrossRef
35. S. J. Harris, WO Patent 95-IE8 19950124, 1995; K. M. Hwang, Y. M. Ki, S. Y. Liu, T. C. Lee, W. Choy, and J. Chen, WO Patent 9403165, 1985; K. M. Hwang, Y. M. Ki, S. Y. Liu, W. Choy, and J. Chen, WO Patent 9403164, 1984; D. Hulmes, A. Coleman, and E. Aubert-Foucher, WO patent 0007585, 2000; J. W. Cornforth, P. D’Arcy Hart, G. A. Nicholls, R. J. W. Rees, and J. A. Stock, J. Pharmacol., 1955, 10, 73; P. D’Arcy Hart, A. J. Armstrong, and E. Brodaty, Infect. Immun., 1996, 64, 1491; A. Casnati, M. Fabbi, N. Pelizzi, A. Pochini, F. Sansone, R. Ungaro, E. Di Modigno, and G. Tarzia, Bioorg. Med. Chem. Lett., 1996, 6, 2699. CrossRef
36. A. B. Salem and J.-B. Regnouf-de-Vains, Tetrahedron Lett., 2001, 42, 7033. CrossRef
37. W. V. Daehne, E. Fredericksen, E. Gundersen, F. Lund, P. Morch, H. J. Petersen, K. Roholt, L. Tybring, and W. O. Godtfredsen, J. Med. Chem., 1970, 13, 607; CrossRef S. A. Matlin, L. Chan, and B. Catherwood, J. Chem. Soc., Perkin Trans. 1, 1990, 89. CrossRef
38. H. Ogura, K. Takeda, and K. Kajima, Chem. Pharm. Bull., 1978, 26, 1688. CrossRef
39. Y. Molard, C. Bureau, H. Parrot-Lopez, R. Lamartine, and J.-B. Regnouf-de-Vains, Tetrahedron Lett., 1999, 40, 6383; CrossRef Y. Molard, Thèse d’Universite, Université Claude Bernard Lyon I, December, 2000.
40. B. Korchowiec, A. B. Salem, Y. Corvis, J.-B. Regnouf de Vains, J. Korchowiec, and E. Rogalska, J. Phys. Chem. B, 2007, 111, 13231. CrossRef
41. A. B. Salem and J.-B. Regnouf de Vains, Tetrahedron Lett., 2001, 42, 7033. CrossRef
42. A. B. Salem and J.-B. Regnouf de Vains, Tetrahedron Lett., 2003, 44, 6769. CrossRef
43. D. Pellico, M. Gmez-Gallego, P. Ramrez-López, M. J. Mancheno, M. A. Sierra, and M. R. Torres, Chem. Eur. J., 2009, 15, 6940. CrossRef
44. M. A. Sierra, M. Rodriguez-Fernandez, L. Casarrubios, M. Gómez-Gallego, C. P. Allen, and M. J. Mancheno, Dalton Trans., 2009, 8399. CrossRef