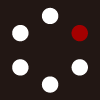
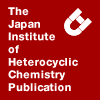
HETEROCYCLES
An International Journal for Reviews and Communications in Heterocyclic ChemistryWeb Edition ISSN: 1881-0942
Published online by The Japan Institute of Heterocyclic Chemistry
e-Journal
Full Text HTML
Received, 19th November, 2014, Accepted, 19th January, 2015, Published online, 23rd January, 2015.
DOI: 10.3987/COM-14-13132
■ Iriomoteolide-2a, a Cytotoxic 23-Membered Macrolide from Marine Benthic Dinoflagellate Amphidinium Species
Keiko Kumagai, Masashi Tsuda,* Atsunori Masuda, Eri Fukushi, and Jun Kawabata
Center for Advanced Marine Core Research , Kochi University, Nankoku, Kochi 783-8502, Japan
Abstract
The first 23-membered macrolide, iriomoteolide-2a (1), was isolated from cultivated algal cells of a marine benthic dinoflagellate Amphidinium species. The structure was determined based on detailed analysis of 2D NMR data. The relative stereochemistries were assigned on the basis of coupling constants and ROESY data, and the absolute configurations were elucidated by analyzing the NMR data of the MTPA esters for 1 and its reduced product. Iriomoteolide-2a (1) exhibited potent cytotoxic activities in vitro and in vivo.INTRODUCTION
Marine phytoplankton such as dinoflagellates have been proven to produce a variety of chemically unique and biologically interesting secondary metabolites such as long-chain polyketides and polyethers.1 It is well known that marine dinoflagellates of the genus Amphidinium produce unique metabolites such as macrolides and/or linear polyketides.2 These macrolides possess various carbon chains forming 12–29-membered macrolactone rings, including odd-numbered lactone rings. Biosynthetically unique structural features such as vicinally located C1 branches are found in a majority of these molecules, and most exhibit potent cytotoxicity.
Our continuing search for new cytotoxic metabolites from marine benthic dinoflagellate Amphidinium species3 has resulted in the isolation of the first naturally occurring 23-membered macrolide, iriomoteolide-2a (1) (Scheme 1), consisting of a continuous carbon chain. Compound 1 exhibited potent cytotoxic activity against tumor cells and in vivo activity against tumor. Here, we describe the isolation, structural elucidation, and biological activities of 1.
RESULTS AND DISCUSSION
The dinoflagellate Amphidinium species (HYA024 strain) was monoclonally separated from benthic sea sand collected off Iriomote Island, Japan. The algal cells (15.3 g, dry weight) obtained from 400 L of the medium were extracted with MeOH/toluene (3:1), and the extracts were partitioned between toluene and H2O. The toluene-soluble materials were subjected to chromatography with SiO2 gel, C18, and amino-SiO2 gel columns, and then C18 HPLC, to afford iriomoteolide-2a4 (1, 0.032% from dry weight).
Iriomoteolide-2a (1) was obtained as an optically active colorless oil {[α]D20 +44 (c 0.23, CHCl3)}. The molecular formula of 1 was established to be C33H54O7 by HRESIMS data [m/z 585.3749 (M + Na)+, Δ −1.8 mmu], indicating seven degrees of unsaturation. 1H and 13C NMR data (CDCl3; Table 1) showed the presence of an ester carbonyl, two sp2 quaternary carbons, three sp2 methines, an sp2 methylene, eleven sp3 methines including eight oxygenated ones, ten sp3 methylenes, and five methyls, accounting for four degrees of unsaturation. The remaining double bond equivalents were inferred that there are three rings in 1. Proton resonances of the ten sp3 methylenes were nonequivalent and close to each other, so their corresponding carbons were assigned using CH2-selected E-HSQC correlations.
Interpretation of the 1H–1H COSY and TOCSY spectra revealed the following three proton–proton connectivities: from H-2 to H-17, H3-29, and H3-30; from H2-19 to H-24 and H3-32; and from H-26 to H3-28 (Figure 1). These networks were supported by CH2-selected E-HSQC-TOCSY correlations for H-5/C-3, H-6/C-8, H2-19/C-20, H2-19/C-21, H-22/C-20, and H3-29/C-3. It was deduced from the J(H-5/H-6) value (15.5 Hz) that the disubstituted double bond at C-5−C-6 had E geometry. The presence of the E-trisubstituted double bond at C-17−C-18 was suggested by HMBC correlations for H3-31/C-17, H3-31/C-18, and H3-31/C-19 and the intensive ROESY correlations for H-16/H3-31 and H-17/H2-19. Attachment of the C-25−C-33 exomethylene to C-24 and C-26 was confirmed from HMBC correlations for H-26/C-25, H3-32/C-25, and H2-33/C-25. HMBC correlations for H-22/C-1 and H3-29/C-1 suggested that C-22 was involved in an ester linkage with C-1. The presence of two tetrahydrofuran rings (C-9−
C-12 and C-13−C-16) was implied by a ROESY correlation for H-9/H-12 and an HMBC correlation for H-13/C-16. The planar structure of iriomoteolide-2a was therefore concluded to be 1.
The stereostructure of 1 was elucidated using the following procedure: 1) elucidation of the relative stereochemistries of C-9−C-16 and C-22−C-27, 2) determination of the absolute configurations of C-11, C-23, and C-26, and 3) elucidation of the absolute configuration of C-2. The 1H–1H coupling constants needed for stereochemical assignments were obtained from resolution-enhanced 1H NMR spectra, and the long-range 13C–1H coupling constants for J-based configuration analysis5 were estimated from the intensities of the correlations in the phase-sensitive HMBC spectrum.6
The relative stereochemistries of the bis-tetrahydrofuran moiety at C-9−C-16 were investigated using ROESY data, as shown in Figure 2a. For the C-9−C-12 moiety, syn relations for H-9−H-11 and H-9−H-12 were assigned by ROESY correlations for H-9/H-11 and H-9/H-12; anti and syn relations were deduced for H-13−H-15 and H-13−H-16, respectively, of the other tetrahydrofuran ring (C-13−C-16) from ROESY correlations for H-13/H-16 and H-13/H3-30. The rotation of the C-12−C-13 bond between the two tetrahydrofuran rings was analyzed using J-based configuration analysis as shown in Figure 2b. The J(H-12/H-13) value (3.1 Hz) indicated a gauche relation for H-12−H-13, and the 2J(C-12/H-13) and 2J(C-13/H-12) values (almost 0 Hz) suggested anti relations for H-12−13-O and H-13−12-O. Considering the HSQC-ROESY correlation for H-11/C-13 and ROESY correlation for H-12/H-14a,7 the relative stereochemistry for C-12−C-13 was concluded to be threo.
For the C-22−C-23 bond (Figure 3a), a gauche orientation for H-22−H-23 was implied by the relative small J(H-22/H-23) value (2.3 Hz), and the relatively large J(C-22/H-23) and small J(C-21/H-23) values (−6 and 7 Hz, respectively) indicated gauche and anti orientations for H-23−O-22 and H-23−C-21, respectively. ROESY correlations for H-21b/H-24 and H-22/H3-32 suggested that the C-22−C-23 bond had the erythro configuration. Based on the relatively large J(H-23/H-24) (10.3 Hz) and J(C-23/H-24) (−8 Hz) values and the ROESY correlation for H-22/H3-32, the relative configuration for C-23−C-24 was inferred to be erythro (Figure 3b). ROESY correlations for H-23/H-26, H-24/H-33b, and H-27b/H3-32 indicated a 1,3-syn configuration for C-24−C-26 through the exomethylene unit. Therefore, the relative configurations of C-22, C-23, C-24, and C-26 were concluded as shown in Figure 3c.
The absolute configuration of 1 was examined by application of modified Mosher’s method.8 Treatment of 1 with (R)-(−)- and (S)-(+)-2-methoxy-2-trifluoro-2-phenylacetyl chloride (MTPACl) gave 11,23,26-tris-(S)- and 11,23,26-tris-(R)-MTPA esters (2a and 2b, respectively) of 1. The 1H NMR data
for 2a and 2b were assigned by analyzing the 1H–1H COSY and TOCSY spectra, and Δδ values (Δδ = δS − δR) were calculated as shown in Figure 4. Around C-11 of the MTPA ester, the Δδ values of H-12, H-13, H2-14, and H3-30 were positive and those of H-9 and H2-10 were negative, suggesting that C-11 had the S configuration. In contrast, positive Δδ values for H-26, H2-27, and H3-28 and negative values for H-22 and H-23 corresponded to Δδ distribution patterns typical of the anti-1,4-diols with chiral anisotropic reagents described by Riguera et al.,9 suggesting the 23R,26R-configuration for 1. Considering the relative stereochemistries, the absolute configurations at C-9, C-12, C-13, C-15, C-16, C-22, and C-24 were elucidated as R, R, R, S, S, S, and R, respectively.
The absolute configuration at C-2 with a methyl group was elucidated on the basis of signal patterns of the two geminal protons at C-110 for the MTPA esters of the seco-alcohol of 1. Reduction of 1 with LAH followed by treatment with (R)- and (S)-MTPACl gave mainly 3a and 3b (Figure 5a). The methylene protons of C-1 in 3a were observed as an overlapping 2H signal at δH 4.15 (Figure 5b), whereas those in 3b appeared as separate signals at δH 4.09 and 4.23 (Figure 5c), indicating that the absolute configuration at C-2 was R.
Iriomoteolide-2a (1) exhibited potent cytotoxicity against human B lymphocyte DG-75 cells (IC50: 0.006 µg/mL) and human cervix adenocarcinoma HeLa cells (IC50: 0.03 µg/mL). Although the compound showed in vivo activity against murine tumor P388 (T/C 132%) at a dose of 0.2 mg/kg, therapeutic effects did not appear at higher doses. Investigations for molecular targets of 1 using surface plasmon resonance revealed to have an affinity for actin. Amphidinolide H,11 a potent cytotoxic macrolide from the Amphidinium dinoflagellate, is actin inhibitor that covalently binds to actin,12 however, 1 seems to bind non-covalently with actin because the resonance disappeared through washing with a running solution.
CONCLUSION
Iriomoteolide-2a (1) is the first reported natural product possessing a 23-membered macrolactone ring consisting of a continuous polyketide chain.13 More than half of the macrolides obtained from Amphidinium species have odd-numbered lactone rings, e.g., 13-, 15-, 17-, 19-, 25-, 27-, and 29-membered macrolides.2 Although Amphidinium macrolides such as amphidinolides C,14 F,15 and M16 have two tetrahydrofuran rings in their molecules, macrolide 1, possessing a bis-tetrahydrofuran moiety, is unique.3 Vicinally-locating C1 branches (C-32 and C-33) on a C-23−C-25 portion are also characteristic of Amphidinium macrolides. Compound 1 showed the potent cytotoxic activity against tumor cells and in vivo activity against tumor.
EXPERIMENTAL
General. Optical rotation and IR were measured on a JASCO DIP-370 polarimeter and a JASCO FT/IR-5300 spectrophotometer, respectively. NMR data were recorded using 2.5 mm microcells for CDCl3 (Shigemi Co., Ltd.). NMR spectra were measured on a Bruker AMX-500 spectrometer equipped with 2.5 mm C/H dual probe. Chemical shifts in CDCl3 are reported in ppm with reference to the solvent residual proton and carbon signals (δH 7.26 and δC 77.0). ESIMS spectra were obtained on a JEOL JMS 700-TZ spectrometer.
Isolation. Material, cultivation and extraction of the dinoflagellate were described previously.17 The toluene-soluble fraction (2 g) of the extract was subjected to SiO2 gel column chromatography (40 X 200 mm) using a stepwise elution of CHCl3 (200 mL) and CHCl3–MeOH (98:2, 200 mL and then 95:5, 200 mL). The fraction eluted with 95% CHCl3–MeOH was chromatographed successively by using C18 (MeCN–H2O, 7:3) and then NH2–SiO2 gel columns (n-hexane–EtOAc, 2:1). A macrolide-containing fraction was separated by C18 HPLC [YMC-Pack Pro C18, 5 μm, YMC Co., Ltd., 10 X 250 mm; eluent, MeCN–H2O (60:40); flow rate, 2 mL/min; UV detection at 210 nm] to afford iriomoteolide-2a (1, 4.3 mg, 0.032% from dry weight).
Iriomoteolide-2a (1). Colorless oil; [α]D20 +44 (c 0.23, CHCl3); IR (neat) νmax 3430 (broad), 2920 1712, 1219, and 1005 cm-1; 1H and 13C NMR (Table 1); ESIMS (pos.) m/z 585 (M+Na)+; ESIMS (neg.) m/z 561 (M-H)-, 597 (M+35Cl)-, and 599 (M+37Cl)-; HRESIMS m/z 585.3749 calcd for C33H54O7Na, (M+Na)+, 585.3767].
11,23,26-Tris-(S)-MTPA ester (2a) of iriomoteolide-2a (1).
To a solution of iriomoteolide-2a (1, 0.2 mg) in 1% DMAP solution in CH2Cl2 (20 µL) were added triethylamine (2 µL) and (R)-(-)-MTPACl (1 µL), and the mixture was stirred at 4 °C for 15 h. After addition of N,N-dimethyl-1,3-propanediamine (2 µL), the solvent was evaporated in vacuo. The residue was passed through a SiO2 gel column (hexane/acetone, 4:1) to afford 5-(S)-MTPA ester (2a) of 1 (0.02 mg): 1H NMR (CDCl3); δ 0.80 (3H, t, J = 6.8 Hz, H3-28), 0.98 (3H, d, J = 6.8 Hz, H3-30), 1.12 (3H, d, J = 6.8 Hz, H3-29), 1.18 (3H, d, J = 6.8 Hz, H3-32), 1.32 (1H, m, H-20b), 1.40 ~ 1.53 (4H, m, H-3b, H-27b, H-20a, and H-21b), 1.63 (1H, m, H-8b), 1.64 (1H, m, H-27a), 1.64 (3H, s, H3-31), 1.72 (1H, H-14b), 1.80~1.85 (4H, m, H-3a, H-8a, H-10b, and H-21a), 1.90~2.04 (3H, m, H-4b, and H2-19), 2.07 (1H, m, H-7b), 2.09 (1H, m, H-4a), 2.20 (1H, m, H-15), 2.21 (1H, m, H-7a), 2.34 (1H, m, H-14a), 2.37 (1H, m, H-10a), 2.49 (1H, m, H-2), 2.53 (1H, m, H-24), 3.51 (3H, s, OCH3), 3.53 (6H, s, 2 X OCH3), 3.68 (1H, m, H-12), 3.90 (1H, m, H-9), 4.20 (1H, m, H-16), 4.47 (1H, m, H-13), 4.97 (1H, s, H-33b), 4.98 (1H, m, H-22), 5.00 (1H, s, H-33a), 5.01 (1H, m, H-17), 5.14 (1H, m, H-23), 5.32 (1H, m, H-26), 5.35 (1H, m, H-11), 5.37 (1H, m, H-5), 5.46 (1H, m, H-6), 7.38 (9H, m, Ph), and 7.50 (6H, m, Ph); HRESIMS m/z 1233.4958 [calcd for C63H75O13F9Na, (M+Na)+: 1233.4916].
11,23,26-Tris-(R)-MTPA ester (2b) of iriomoteolide-2a (1). A solution of iriomoteolide-2a (1, 0.2 mg) in CH2Cl2 (20 µL) containing 1% DMAP was treated with triethylamine (5 µL) and (S)-(+)-MTPACl (1.5 µL) by the same procedure as described above to afford 5-(R)-MTPA ester (2b) of 1 (0.12 mg): 1H NMR (CDCl3); δ 0.78 (3H, t, J = 6.8 Hz, H3-28), 0.96 (3H, d, J = 6.8 Hz, H3-30), 1.12 (3H, d, J = 6.8 Hz, H3-29), 1.15 (3H, d, J = 6.8 Hz, H3-32), 1.33 (1H, m, H-20b), 1.40 ~ 1.53 (4H, m, H-3b, H-27b, H-20a, and H-21b), 1.60 (1H, m, H-27a), 1.63 (1H, m, H-8b), 1.64 (3H, s, H3-31), 1.70 (1H, m, H-14b), 1.80~1.85 (4H, m, H-3a, H-8a, H-10b, and H-21a), 1.90~2.04 (3H, m, H-4b, and H2-19), 2.08 (1H, m, H-7b), 2.10 (1H, m, H-4a), 2.17 (1H, m, H-15), 2.21 (1H, m, H-7a), 2.29 (1H, m, H-14a), 2.41 (1H, m, H-10a), 2.49 (1H, m, H-2), 2.51 (1H, m, H-24), 3.51 (3H, s, OCH3), 3.52 (3H, s, OCH3), 3.53 (3H, s, OCH3), 3.59 (1H, m, H-12), 3.93 (1H, m, H-9), 4.19 (1H, m, H-16), 4.43 (1H, m, H-13), 4.90 (1H, s, H-33b), 5.00 (H-17), 5.02 (1H, s, H-33a), 5.04 (1H, m, H-22), 5.06 (1H, m, H-23), 5.26 (1H, m, H-26), 5.33 (1H, m, H-11), 5.37 (1H, m, H-5), 5.46 (1H, m, H-6), 7.38 (9H, m, Ph), and 7.50 (6H, m, Ph); HRESIMS m/z 1233.4937 [calcd for C63H75O13F9Na, (M+Na)+: 1233.4916].
1-(S)-MTPA ester (3a) of seco-alcohol of iriomoteolide-2a (1). To a solution of iriomoteolide-2a (1, 0.5 mg) in THF (20 µL) was added LiAlH4 (2 mg) and the mixture was stirred at 4 °C for 1 h. After addition of MeOH (10 µL), the mixture was partitioned between EtOAc and brine. After evaporation of the organic solvent, the residue was treated with triethylamine (2 µL) and (R)-(-)-MTPACl (1 µL) in 1% DMAP solution in CH2Cl2 (20 µL), and the mixture was stirred at 4 °C for 15 h. After addition of N,N-dimethyl-1,3-propanediamine (2 µL), the solvent was evaporated in vacuo. The residue was passed through a SiO2 gel column (hexane/acetone, 2:1) to afford 1-(S)-MTPA ester (3a) of seco-alcohol of 1 (0.05 mg): 1H NMR (CDCl3); δ 0.87 (3H, t, J = 6.8 Hz, H3-28), 1.00 (3H, d, J = 6.8 Hz, H3-30), 1.08 (3H, d, J = 6.8 Hz, H3-29), 1.12 (3H, d, J = 6.8 Hz, H3-32), 1.30~1.50 (7H, m), 1.64 (3H, s, H3-31), 1.60~1.90 (5H, m), 1.90~2.27 (9H, m), 2.36 ~2.48 (2H, m, H-2 and H-24), 3.51 (3H, s, OCH3), 3.65 (1H, m, H-12), 3.62 (1H, m, H-13), 3.78 (1H, m, H-22), 3.98 ~ 4.06 (2H, m, H-9 and H-26), 4.15 (2H, m, H2-1), 4.23 (1H, m, H-16), 4.40 ~ 4.45 (2H, m, H-11 and H-13), 5.01 (1H, s, H-33a), 5.05 (1H, s, H-33b), 5.12 (1H, m, H-17), 5.40 ~ 5.50 (2H, m, H-5 and H-6), 7.38 (3H, m, Ph), and 7.50 (2H, m, Ph); HRESIMS m/z 805.4488 [calcd for C43H65O9F3Na, (M+Na)+: 805.44784].
1-(R)-MTPA ester (3b) of seco-alcohol of iriomoteolide-2a (1). Iriomoteolide-2a (0.5 mg) was reduced using LiAlH4 and then esterified with (S)-(+)-MTPACl by the same procedure as described above to afford 1-(R)-MTPA ester (3b) of seco-alcohol of 1 (0.08 mg): 1H NMR (CDCl3); δ 0.87 (3H, t, J = 6.8 Hz, H3-28), 0.97 (3H, d, J = 6.8 Hz, H3-30), 1.07 (3H, d, J = 6.8 Hz, H3-29), 1.10 (3H, d, J = 6.8 Hz, H3-32), 1.30~1.50 (7H, m), 1.64 (3H, s, H3-31), 1.60~1.90 (5H, m), 1.86~2.27 (9H, m), 2.34 ~2.50 (2H, m, H-2 and H-24), 3.58 (1H, m, H-12), 3.60 (1H, m, H-13), 3.87 (1H, m, H-22), 3.97 (1H, m, H-9), 4.07 (1H, m, H-26), 4.09 (1H, m, H-1a), 4.23 (1H, m, H-1b), 4.26 (1H, m, H-16), 4.30 (1H, m, H-11), 4.34 (1H, m, H-13), 4.97 (1H, s, H-33a), 5.07 (1H, s, H-33b), 5.08 (1H, m, H-17), 5.40 ~ 5.50 (2H, m, H-5 and H-6) 7.38 (3H, m, Ph), and 7.50 (2H, m, Ph); HRESIMS m/z 805.4488 [calcd for C43H65O9F3Na, (M+Na)+: 805.44784].
Cytotoxic assay. Cytotoxic assay using DG-75 and Hela cells was carried out at a density of 5,000 cells per well into 96-well plates in DMEM medium containing 10% fetal calf serum at 37 ºC in 5% CO2. After 72 h, the number of viable cells was counted using Cell Counting Kit 8 (Dojindo Co., Kumamoto, Japan) according to the manufacturer's instructions. The assay reagent is a tetrazolium compound (WST-8) that is reduced by live cells into a colored formazan product measured at 450 nm using a microplate reader (Bio-Rad, USA). The experiments were repeated in triplicate wells. The viability of the treated groups was estimated as a percentage of control groups. The cytotoxicity was shown as the concentration causing a 50% reduction of cell growth (IC50). Doxorubicin (IC50 against DG-75 and Hela cells: 0.03 and 0.04 µg/mL) was used as a positive control.
In vivo antitumor activity. The ascites fluid containing 106 cells of murine leukemia P388 cell were transplanted i.p. into CDF1 mice. Iriomoteolide-2a (1) was dissolved in EtOH. The 0.1 mL solution of 1 was administered at day 1 and day 5 after the tumor inoculation (day 0). Dose of 1 was in the range of 1 ~ 20 µg per mice. Antitumor activity was expressed by T/C (the mean survival time of the treated group divided by that of the untreated group) at the each dosage of 1. Five mice were used for each experimental group.
Surface plasmon resonance assay. The binding affinities of objective samples to the actin were determined using SPR biosensor technology (BIACORE J, GE Healthcare, Wisconsin, USA). The purified tubulin and actin were immobilized onto the CM5 chip surface by amino coupling method using HBS-N (10 mM Hepes/0.15 M NaCl, pH7.4) buffer as a running buffer. 1µM DMSO solution of iriomoteolide-2a (1) was injected for 2 min over the immobilized surface .The specific binding profiles of 1 to immobilized tubulin and actin were obtained after subtracting the response signal from the control flow cells. All the data were collected at 25 °C with running buffer HBS-N at a constant flow of 30 μl/min. Binding affinities of the compounds to actin were evaluated with Biacore Evaluation Version 3.0 software (GE Healthcare, Wisconsin, USA).
ACKNOWLEDGEMENTS
We thank R. Iwamoto, Y. Okammoto, Y. Endo, Y. Nagakita, and Y. Fukuda, for dinoflagellate cultivation and measurement of bioactivity and S. Oka and A. Tokumitsu of the Equipment Management Center, Hokkaido University, for ESI-MS measurements. This study was partially supported by a Grant-in-aids for Scientific Research (Nos. 25860079 to K. K. and 26670045 to M. T.) from the Ministry of Education, Culture, Sports, Science, and Technology (MEXT) of Japan.
References
1. J. W. Blunt, B. R. Copp, R. A. Keyzers, M. H. G. Munro, and M. R. Prinsep, Nat. Prod. Rep., 2014, 31, 160 and its previous reviews. CrossRef
2. J. Kobayashi and T. Kubota, J. Nat. Prod., 2007, 70, 451; CrossRef J. Kobayashi and M. Tsuda, Nat. Prod. Rep., 2004, 21, 77 and references cited therein. CrossRef
3. M. Akakabe, K. Kumagai, M. Tsuda, Y. Konishi, A. Tominaga, M. Tsuda, E. Fukushi, and J. Kawabata, Tetrahedron, 2014, 70, 2962; CrossRef K. Kumagai, M. Tsuda, E. Fukushi, and J. Kawabata, Hetereocycles, 2013, 87, 2615 and references cited therein. CrossRef
4. M. Tsuda, K. Oguchi, R. Iwamoto, and Y. Okamoto, Japanese Patent 2006-351043 JP, 2006.
5. N. Matsumori, D. Kaneno, M. Murata, H. Nakamura, and K. Tachibana, J. Org. Chem., 1999, 64, 866. CrossRef
6. nJ(C/H) values were obtained by the equation [ICa/H/ICb/H = sin2(πnJ(Ca/H)Δ)/sin2(πnJ(Cb/H)Δ)]7 supposing 3J(C-21/H3-23) as 7 Hz: H.-O. Kalinowski, S. Berger, and S. Braun, ‘Carbon-13 NMR Spectroscopy,’ John Wiley & Sons, Chichester, 1988, p. 533.
7. Low- and high-field resonances of geminal pairs were denoted as a and b.
8. I. Ohtani, T. Kusumi, Y. Kashman, and H. Kakisawa, J. Am. Chem. Soc., 1991, 113, 4092. CrossRef
9. J. M. Seco, M. Martino, E. Quiñoá, and R. Riguera, Org. Lett., 2000, 2, 3261. CrossRef
10. M. Tsuda, Y. Toriyabe, T. Endo, and J. Kobayashi, Chem. Pharm. Bull., 2003, 51, 448. CrossRef
11. J. Kobayashi, H. Shigemori, M. Ishibashi, T. Yamasu, H. Hirota, and T. Sasaki, J. Org. Chem., 1991, 56, 5221; CrossRef J. Kobayashi, K. Shimbo, M. Sato, M. Shiro, and M. Tsuda, Org. Lett., 2000, 2, 2805. CrossRef
12. T. Usui, S. Kazami, N. Dohmae, Y. Mashimo, H. Kondo, M. Tsuda, G. Terasaki, K. Ohashi, J. Kobayashi, and H. Osada, Chemistry & Biology, 2004, 11, 1269. CrossRef
13. Tacrolimus is a 23-membered macrolide containing a lactam group in the macrocyclic ring: T. Kino, H. Hatanaka, M. Hashimoto, M. Nishiyama, T. Goto, M. Okuhara, M. Kohsaka, H. Aoki, and H. Imanaka, J. Antibiot., 1987, 40, 1249. CrossRef
14. J. Kobayashi, M. Ishibashi, M. R. Wälchli, H. Nakamura, Y. Hirata, T. Sasaki, and Y. Ohizumi, J. Am. Chem. Soc., 1988, 110, 490; CrossRef T. Kubota, M. Tsuda, and J. Kobayashi, Org. Lett., 2001, 3, 1363. CrossRef
15. J. Kobayashi, M. Tsuda, M. Ishibashi, H. Shigemori, T. Yamasu, H. Hirota, and T. Sasaki, J. Antibiot., 1991, 44, 1259. CrossRef
16. J. Kobayashi, N. Yamaguchi, and M. Ishibashi, J. Org. Chem., 1994, 59, 4698. CrossRef
17. M. Tsuda, K. Oguchi, R. Iwamoto, Y. Okamoto, J. Kobayashi, E. Fukushi, J. Kawabata, T. Ozawa, A. Masuda, Y. Kitaya, and K. Omasa, J. Org. Chem., 2007, 72, 4469. CrossRef