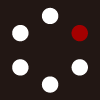
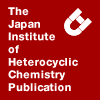
HETEROCYCLES
An International Journal for Reviews and Communications in Heterocyclic ChemistryWeb Edition ISSN: 1881-0942
Published online by The Japan Institute of Heterocyclic Chemistry
e-Journal
Full Text HTML
Received, 5th March, 2015, Accepted, 6th April, 2015, Published online, 14th April, 2015.
DOI: 10.3987/COM-15-13206
■ Diasterospecific Etherification and Diasteroselective Monobromination of (R)-(—)-1-(2-Hydroxy-1-phenylethyl)-3,4-dihydropyridin-2(1H)-one
Anna Vargas, María L. Orea,* Joel L. Terán, David M. Aparicio, Jorge R. Juárez, Raúl G. Enríquez, and Dino Gnecco
Centro de Química, Instituto de Ciencias, Benemérita Universidad Autónoma de Puebla, Edif. 103G, 103H, 72570, Mexico
Abstract
A one pot diasterospecific etherification and diasteroselective monobromination with bromine or N-bromosuccinimide (NBS) of the endocyclic enamide (R)-(—)-1-(2-hydroxy-1-phenylethyl)-3,4-dihydropyridin-2(1H)-one 1 is described. The enantiopure 8-bromo-3-phenyltetrahydro-2H-oxazolo[3,2-a]pyridin-5(3H)-ones trans-2 and cis-2 were obtained in good yield, fully characterized and its relative stereochemistry was unambiguously assigned via single-crystal X-ray structure.The rather unique reactivity of the endocyclic enamides derived from (S)-(+)- or (R)-(-)-2-phenylglycinol allow these compounds to be valuable starting materials to prepare enantiopure bicyclic lactams.1a-d These lactams continue to be a topic of significant interest since they are key intermediates in the stereocontrolled synthesis of alkaloids.2a-c
In previous work, we have described the preparation of endocyclic enamides, their transformations into the corresponding bicyclic lactams, and their utilization in the enantioselective synthesis of alkaloids (Scheme 1).3a-d
Now, based on our interest in developing an asymmetric methodology toward chiral intermediates from chiral endocyclic enamides derived from (R)-(-)-2-phenylglycinol, we report herein the diastereoselective monobromination-etherification of 1 with bromine or N-bromosuccinimide with the purpose to obtain chiral monobromo bicyclic lactams (Scheme 2).
Firstly, we carried out the monobromo-etherification of 1 following the methodology reported by Amat et al.4,5 (Br2/MeONa/MeOH) and the diastereomeric mixture trans-2 + cis-2 was obtained in 50% yield with a 80:20 diastereomeric ratio (Table 1, entry 1). Because of this low yield, it was therefore decided to carry out screening experiments to find out a better diastereoselectivity and chemical yields. When this reaction was carried out with Br2/Et3N/MeOH, the yield was raised to 65% in a 65:35 diastereomeric ratio (Table 1, entry 2). The use of Et3N as a base and CCl4 as a solvent afforded a mixture of trans-2 + cis-2 compounds in almost 50:50 ratio (Table 1, entries 3 and 4). Then, we decided to change the brominating agent by NBS6a-c (Table 1, entries 5 and 6). In contrast, treatment of 1 with NBS/MeCN afforded the diastereomeric mixture trans-2+cis-2 in only 2 hours in a 23:77 ratio in favor of the syn addition product in a 78% chemical yield. This latter condition is rather surprising since it affords the highest overall yield while a reversal in diastereoselectivity is observed (Table 1, entry 6).7 In order to establish if the choice of solvent and base is more influential in the diastereoselectivity outcome, we perform crossover experiments. When we used Br2/Et3N/MeCN, the diastereomeric mixture trans-2 + cis-2 was obtained in 67% yield with a 60:40 diastereomeric ratio (Table 1, entry 7), while, when the reaction was performed with NBS/MeONa/MeOH the compounds obtained were a mixture of 5-bromo-1-((R)-2-hidroxy-1-phenylethyl-6-methoxypiperidin-2-one diastereoisomers in 88% chemical yield in a 1:1:1:1 diastereomeric ratio (not shown in the Table).
These results show that, regardless of the reaction conditions used in this process, the intramolecular etherification of 1 is always diastereospecific and this could be explained in terms of an intramolecular bridge between the methyne hydrogen and carbonyl oxygen of the amide group8 allowing the hydroxyl group attack to the N-acyliminium carbon more favored to generate the chiral center C-8a (S).
In addition, the configuration as (R) or (S) at C-8, as well as the diastereomeric ratio of the mixture depend on the nature of brominating agent used. A proposed mechanism could be explained as follows. Under basic conditions, in where the trans-product is obtained as a major diastereoisomer, the diastereoselectivity is controlled by an electrostatic repulsion between the negative charge on the alkoxide function and bromine, favor the addition of bromine from the re-face of the double bond, while the cis-product is obtained as a major diastereoisomer as consequence of an hydrogen bridge between oxygen of the hydroxyl function and succinimide and the steric repulsion between the phenyl group and succinimide which determines the formation of the π-complex from the si-face (Scheme 3).
The diastereoisomers were readily separated by column chromatography and then, the two diastereoisomers trans-2 and cis-2 crystallized, and the absolute configuration was determined by X-ray analysis (Figure 1 and 2).9
In conclusion, from the data described herein, it is clear that the diastereomeric ratio trans-2:cis-2 depends on the bromination agent used. This result is clearly useful because it allows the access of the diastereomer required as starting material for the asymmetric synthesis of alkaloids in good yield. In addition, it is the first time that the X-ray diffraction is reported for the compounds 2.
EXPERIMENTAL
The 1H and 13C NMR spectra were determined in CDCl3 using TMS as an internal reference with a Varian VX400 FT NMR spectrometer operating at 400 and 100 MHz respectively. IR spectra were obtained with a Nicolet FTIR Magna 750 spectrometer. Optical rotations were determined at room temperature with a Perkin-Elmer 341 polarimeter, using a 1dm cell with a total volume of 1 mL and are referenced to the D-line of sodium. Mass spectra were recorded with a JEOL JEM-AX505HA instrument at a voltage of 70 eV.
Monobromoetherification of 1 with NBS
To a solution of 1 (150 mg, 0.691 mmol) in MeCN (5 mL) at room temperature was added N-bromosuccinimide (147 mg, 0.829 mmol) and the reaction was stirred for 2 h. Then the reaction was concentrated, water added and extracted with CH2Cl2 (3x20 mL). The organic phase was dried over anhydrous Na2SO4, filtered, and the solvent removed. The diastereomeric mixture trans-2+cis-2 was obtained in 78% yield and a 23:77 ratio. The mixture was resolved by flash chromatography on silica gel (petroleum ether/EtOAc 3:1).
(3R,8R,8aS)-(-)-8-Bromo-3-phenylhexahydrooxazolo[3,2-a]pyridin-5-one trans-2: solid; mp 176 °C, [α]20 -38.5 (c 1.0, CH2Cl2). 1H NMR (500 MHz, CDCl3) 2.27 (m, 1H, H-7,), 2.42 (m, 1H, H-7), 2.51 (m, 1H, H-6), 2.65 (m, 1H, H-6), 3.86 (ddd, J = 12.8, 8.0, 3.8 Hz, 1H, H-8), 3.89 (dd, J = 9.1, 7.2 Hz, 1H, H-2), 4.49 (dd, J = 9.1, 7.8 Hz, 1H, H-2), 5.15 (d, J = 8.0 Hz, 1H, H-8a), 5.32 (dd, J = 7.8, 7.2 Hz, 1H, H-3), 7.29-7.36 (m, 5H, ArH). 13C NMR (125 MHz, CDCl3) 28.6 (C-7), 31.6 (C-6), 45.0 (C-8), 59.0 (C-2), 72.1 (C-3), 92.1 (C-8a), 126.2 (2CH), 127.9 (CH), 128.9 (2CH), 138.7 (C i), 166.7 (CO). MS (EI): calcd for C13H1479BrNO2 ([M+H])+ 295; found: 295.
(3R,8S,8aS)-(-)-8-Bromo-3-phenylhexahydrooxazolo[3,2-a]pyridin-5-one cis-2: mp 118 °C, [α]20 -98.8 (c 1.0, CH2Cl2). 1H NMR (500 MHz, CDCl3) 2.29 (m, 1H, H-7), 2.49 (m, 1H, H-7), 2.52 (m, 1H, H-6), 2.74 (m, 1H, H-6), 4.02 (dd, J = 8.5, 5.2 Hz, 1H, H-2), 4.50 (dd, J = 8.5, 7.2, Hz, 1H, H-2), 4.71 (m, 1H, H-8), 5.07 (d, J = 2.8 Hz, 1H, H-8a), 5.40 (dd, J = 7.2, 5.2 Hz, 1H, H-3), 7.29-7.35 (m, 5H, ArH). 13C NMR (125 MHz, CDCl3) 25.0 (C-7), 27.4 (C-6), 49.0 (C-8), 58.1 (C-2), 72.2 (C-3), 87.4 (C-8a), 126.3 (2CH), 127.8 (CH), 128.8 (2CH), 139.0 (C i), 166.6 (CO). MS (EI): calcd for C13H1479BrNO2 ([M+H])+ 295; found: 295.
ACKNOWLEDGEMENTS
We are grateful to CONACyT (Project CB-2009-01-132822) and VIEP-BUAP for financial support.
References
1. J. B. Schwarz and A. I. Meyers, J. Org. Chem., 1998, 63, 1732; CrossRef M. J. Munchhof and A. I. Meyers, J. Am. Chem. Soc., 1995, 117, 5399; CrossRef M. J. Munchhof and A. I. Meyers, J. Org. Chem., 1995, 60, 7084; CrossRef M. D. Groaning and A. I. Meyers, Tetrahedron, 2000, 56, 9843. CrossRef
2. M. Amat, C. Escolano, O. Lozano, N. Llor, and J. Bosch, Org. Lett., 2003, 5, 3139; CrossRef M. Amat, C. Escolano, N. Llor, O. Lozano, A. Gómez-Esqué, R. Griera, and J. Bosch, ARKIVOC, 2005, ix, 115; CrossRef M. Amat, N. Llor, J. Hidalgo, C. Escolano, and J. Bosch, J. Org. Chem., 2003, 68, 1919. CrossRef
3. J. L, Terán, D. Gnecco, A. Galindo, J. Juárez, S. Bernes, and R. G. Enríquez, Tetrahedron: Asymmetry, 2001, 12, 357; L. F. Roa, D. Gnecco, A. Galindo, and J. L. Terán, Tetrahedron: Asymmetry, 2004, 15, 3393; CrossRef A. M. Lumbreras, D. Gnecco, J. L. Terán, J. Juárez, M. L. Orea, R. G. Enríquez, and S. Hernández, J. Mex. Chem. Soc., 2007, 51, 103; D. Gnecco, A. M. Lumbreras, J. L. Terán, A. Galindo, J. Juárez, M. L. Orea, A. Castro, R. Enríquez, and W. F. Reynolds, Heterocycles, 2009, 78, 2589. CrossRef
4. M. Amat, N. Llor, M. Huguet, E. Molins, E. Espinosa, and J. Bosch, Org. Lett., 2001, 3, 3257. CrossRef
5. Amat et al.4 did not describe the 1H NMR and 13C NMR of trans and cis compounds.
6. Ch. K. Tan, W. Z. Yu, and Y.-Y. Yeung, Chirality, 2014, 26, 328; CrossRef D. F. Taber and P. W. DeMatteo, J. Org. Chem., 2012, 77, 4235; CrossRef J. Chen, S. Chng, L. Zhou, and Y.-Y. Yeung, Org. Lett., 2011, 13, 6456. CrossRef
7. Identification of the cis and trans addition product was confirmed by the displacement and magnitude of the coupling constant of H-8a (trans: 5.15 ppm, J = 8.0 Hz), (cis: 5.07 ppm, J = 2.8 Hz).
8. Identification of the cis and trans addition product was confirmed by the displacement and magnitude of the coupling constant of H-8a (trans: 5.15 ppm, J = 8.0 Hz), (cis: 5.07 ppm, J = 2.8 Hz).
9. CCDC-841458 (for trans-2), CCDC 841457 (for cis-2). These data can be obtained free of charge from The Cambridge Crystallographic Data Centre via www.ccdc.cam.ac.uk/data_request/cif.