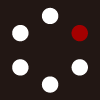
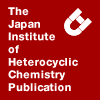
HETEROCYCLES
An International Journal for Reviews and Communications in Heterocyclic ChemistryWeb Edition ISSN: 1881-0942
Published online by The Japan Institute of Heterocyclic Chemistry
e-Journal
Full Text HTML
Received, 9th May, 2015, Accepted, 29th June, 2015, Published online, 14th July, 2015.
DOI: 10.3987/REV-15-822
■ Synthesis and Bioactivity of Pyrroloindazoles: An Overview
Manas Chakrabarty,* Moumita Rakshit, and Gandhi K Kar
Department of Chemistry, Presidency University, 86/1, College Street, Kolkata-700073, India
Abstract
In this review, the synthesis and bioactivity of pyrroloindazoles have been presented. The pyrroloindazoles were synthesised either by the heteroannulation of tetrahydro-indolones/isoindolones, aminoindazoles, Sonogashira/Cacchi indazolic substrates or acyl/aroylindole ketoxime dinitrophenyl ethers or by the cycloaddition of indolynes, indazolic betaines, styrylpyrazoles and nitroindazoles. Several pyrroloindazoles showed analgesic, antiinflammatory and antitumor properties, inhibition of Pim kinase and soluble guanylate cyclase, and antagonism of NMDA-receptor.CONTENTS
1. Introduction
2. Review
2.1 Synthesis of pyrrolo[2,3-g]indazoles (I)
2.2 Synthesis of pyrrolo[3,2-e]indazoles (II)
2.3 Synthesis of pyrrolo[3,2-f]indazoles (III)
2.4 Synthesis of pyrrolo[2,3-e]indazoles (IV)
2.5 Synthesis of pyrrolo[3,2,1-hi]indazoles (V)
2.6 Synthesis of pyrrolo[1,2-b]indazoles (VI)
2.7 Synthesis of pyrrolo[3,4-g]indazoles (VII)
2.8 Synthesis of pyrrolo[3,2-g]indazoles (VIII)
3. Bioactivity of pyrroloindazoles
4. Conclusion
5. References
1. iNTRODUCTION
Heterocycles are integral parts of the majority of current drugs1 because they modulate the druglikeness of such molecules.2,3 Heterocyclic compounds, therefore, play a crucial role in drug development4,5 and consequently in our daily life.6 Amongst heterocycles, indolic substances are by far the most thoroughly studied class7-9 displaying diverse bioactivities.10-12 Two current reviews on the biomedical importance of indoles13,14 are pertinent in this regard.
In contrast, indazoles, the bioisosters of indoles, have been studied to a lesser extent.15-17 There are three indazolic natural products (Nigella alkaloids),18-20 a few indazolic drugs, viz. bendazac lysine,21 benzydamine,22 granisetron,23,24 lonidamine,25-28 YC-129-31 and axitinib32 and an illegal cannabinoid substitute (APINACA or AKB-48) reported from Japan33 and detected in “herbal incense” or “spice” in Italy.34 Two recent reviews35,36 cover the bioactivities of indazoles. The medicinal aspects of both indoles and indazoles have also been reviewed recently.37
Since both indoles and indazoles are useful molecules, pyrroloindazoles (which may also be considered as pyrazoloindoles), henceforth abbreviated as PIZs, are a matter of curiosity since a PIZ embodies both an indole and an indazole nucleus. Although a large number of PIZs have been synthesised and quite a few of them display a variety of bioactivities, no review appears to exist on the synthesis and bioactivities of PIZs. The present review is expected to fill in this lacuna.
2. REVIEW
Eight isomeric classes of PIZs (I-VIII) (Figure 1) have been synthesised till date. Of them, P[2,3-g]IZs (I) were the first to have been synthesised and their synthesis has also been more extensively studied.
Accordingly, the PIZs have been discussed here chronologically, beginning with the P[2,3-g]IZs. Since the PIZs are, as per the mancude system of IUPAC nomenclature, considered as dihydro-PIZs, the P[2,3-g]IZs, for example, are represented as 1,6-dihydro- or 1H,6H-P[2,3-g]IZs.
2.1 Synthesis of pyrrolo[2,3-g]indazoles (I)
The first synthesis of PIZs was reported in a US Patent in 1968.38 A large number of substituted 7-methyl-3H,6H-P[2,3-g]IZs were reportedly prepared by the heteroannulation of the known α-hydroxymethylenetetrahydroindol-4-one (1B) or its precursor (1A) with (substituted) hydrazines, followed by aromatisation (Pd-C) and indolic N-debenzylation (Na/liq NH3) and, in some cases, substitution at indolic C-3. The crucial indolone 1B was prepared from 2-acetonylcyclohexane-1,3-dione via 1A (Scheme 1).
Some of the resulting novel PIZs proved to be bioactive, discussed later.
Several 1- and 2-substituted derivatives of 2a and 2b were also prepared by derivatisation (Scheme 2).
3-Amino (4a) and 1-phenyl (4b) derivatives of the parent 7-methyl-P[2,3-g]IZ (2b) were prepared from 1B in a similar way (Scheme 3).
The precursor 1A was converted to 3-phenyl and 3-hydroxy derivatives of 2b by initial acylation, followed by similar routes (Scheme 4).
6-Ethyl analogues of 2b, viz. 6a,b were similarly prepared from the corresponding tetrahydroindol-4-ones which, in turn, were prepared from appropriately substituted cyclohexane-1,3-diones (Scheme 5).
In a subsequent patented report,39 several P[2,3-g]IZs were prepared by treating a substituted 6-hydrazinoindazole with a ketone and then cyclising the resulting hydrazone in an acidic or aq. alcoholic medium at room temperature to boiling point. The details of this work could not be retrieved.
Subsequently, N. Anand et al. followed the same path to prepare four 4,5-dihydro-1H,6H-P[2,3-g]IZs (8a-d) starting from the tetrahydroindolones (Scheme 6).40
The 8-Me protons were stated to appear at δ 2.56 in 8a but at δ 1.66 at 8b due to the positioning of the methyl group in the shielding zone of the phenyl group of the latter.
In search of fluorescent condensed indazoles, each of 6-aminoindazole (9a) and its 2-methyl derivative (9b) was separately condensed with benzoin in acetic acid under reflux to furnish the corresponding 7,8-diphenyl-1H,6H- (10) and -2H,6H-P[2,3-g]IZs (11) (Scheme 7).41 The angular structures were claimed primarily on the basis of 1H NMR spectroscopic data. None of the products showed strong fluorescence.
In search of novel potential NR2B subtype-selective N-methyl-D-aspartate (NMDA)- receptor antagonists for the treatment of neuropathic pain, 7-carbethoxy-1H,6H-P[2,3-g]IZ was prepared from 9a by the Fischer/Japp-Klingemann procedure. The structures were fully supported by 1H NMR and HRMS data. The derived carboxamide (12) (Scheme 8) proved to be bioactive,42-44 discussed later.
In search of inhibitors of sGC (soluble guanylate cyclase), potential therapeutic agents in hypotension, 1-methyl/phenyl-1H,6H-P[2,3-g]IZs were prepared from 1-substituted 5-hydroxymethylene-4-oxo- 4,5,6,7-tetrahydroindoles (Scheme 9), following the earliest reported method. The two 4,5-dihydro- 6(N)-SEM-PIZs (14a,b) were oxidised (DDQ) and then deprotected by BF3·Et2O, followed by Triton B to the corresponding 1-substituted PIZs in 37/26% yields.45 Some of the products displayed expected bioactivity, discussed later.
The starting 5-hydroxymethylenetetrahydroindolones were prepared from cyclohexnane-1,3-dione in a three-step procedure, shown below (Scheme 10).
Indolynes, generated in situ, function as electrophilic indole surrogates and can react with synthetically useful nucleophiles regioselectively. During studies on understanding and modulating the regioselectivities of indolynes, generated in situ, it was hypothesised that a bromine at C-6 of the 4,5-indolyne 15 would reverse its regioselectivity at C-4. This hypothesis was validated by the energy minimised structure of 6-bromo-4,5-indolyne and proven by actual chemical reactions. Thus, 15, generated in situ, reacted with ethyl diazoacetate to furnish a mixture of the 1H,6H-P[2,3-g]IZs (16a/b) and the 1H,6H-P[3,2-e]IZs (17a/b) (Scheme 11).46,47
The PIZs 16a,b resulted from nucleophilic attack at C-5 whereas the PIZs 17a,b resulted from attack at C-4 of the respective indolynes (15), generated in situ. The observed reversal of regioselectivity was stated to have arisen from a combination of indolyne distortion and steric effects caused by the bromide substituent.
A number of 7- and 8-substituted and 7,8-disubstituted 1H,6H-P[2,3-g]IZs were synthesised using palladium-catalysed annulations of the ortho-amino-iodoindazole (19) with activated acetylenes.48 The starting material was prepared from commercially available 6-nitroindazole (Scheme 12).
The reaction of 19 with TMS-acetylene using Pd(OAc)2/BINAP as the catalyst furnished a 1H,6H-P[2,3-g]IZ (20) efficiently (Scheme 13).
Changing the catalyst to Pd(PPh3)4/XPhos led to an enhanced yield of 20 (from 19) and, when applied to phenylacetylene, efficiently provided a PIZ (21) with reverse regioselectivity. But for other terminal acetylenes used, the reagent led to a mixture of the corresponding 7/8-isomers (22a-e + 23a-e) in each case (Scheme 14). The observed unusual reactivity and regioselectivity were thought to be due, in part, to an effect of the bulky electron-rich ligand XPhos. Larock annulation was assumed to be most likely involved in the formation of 20.
Internal alkynes furnished mixed results (Scheme 15).
Subsequently, a number of deprotected 5-nitro-1H,6H-P[2,3-g]IZs and the corresponding 5-amino-PIZs were prepared (Scheme 16).49 Some of the PIZs (25-27) inhibited Pim kinases (see later).
2.2 Synthesis of pyrrolo[3,2-e]indazoles (II)
In the same work where P[2,3-g]IZs were prepared from 6-aminoindazoles by Fischer/Japp-Klingemann procedure,42 a P[3,2-e]IZ was prepared from 5-aminoindazole using the same route. It was converted to the corresponding carboxamide (28) (Scheme 17) which proved to be bioactive (see later).
The indolyne route to [3,2-e]-type PIZs has already been discussed.46,47
In search of novel antineoplastic agents, 4-formyl-3-methylpyrazole was converted in several steps to a number of 4,5-dihydro-1H,6H-P[3,2-e]IZs (29A, 29B) (Scheme 18).50 Since patented, the details of the work could not be retrieved.
The authors of the present review developed the first general synthesis of 7-substituted 1H,6H-P[3,2-e]IZs (33a-h) by tandem Sonogashira/Cacchi coupling-heteroannulation of 4-iodo-1- phenylsulfonyl-5-trifluoroacetamidoindazole (30) with a number of terminal acetylenes (31a-h) using Pd(PPh3)2Cl2 (10 mol%) as the catalyst, CuI (10 mol%) as the co-catalyst and Et3N (10 eqv) as the base in DMF under argon atmosphere at 110 °C, followed by N(3)-deprotection of the resulting PIZs (32a-h) by aq. methanolic K2CO3 under reflux (Scheme 19).51
The starting indazole was efficiently prepared from commercially procured 5-nitroindazole by successive N(3)-protection (-SO2Ph), reduction of the nitro to the amine, iodination (ortho- to the amine) and N-trifluoroacetylation (Scheme 20).
2.3 Synthesis of pyrrolo[3,2-f]indazoles (III)
Following their previously reported route to P[2,3-g]IZs,41 Seshadri et al. blocked the 7-position of 6-aminoindazole and carried out its acid-catalysed condensation with benzoin, which efficiently furnished 1H,7H-P[3,2-f]IZ (35) (Scheme 21). Pertinently, this compound was erroneously named as a pyrrolo[2,3-f]indazole. Although this work was undertaken in search of fluorescent PIZs, 35 did not show strong fluorescence.
2.4 Synthesis of pyrrolo[2,3-e]indazoles (IV)
In this first report on the use of heterocyclic quinones in Nenitzescu reaction, 1,3-diphenyl- 4,7-dioxo-4,5,6,7-tetrahydroindazole was condensed with four ethyl β-(alkyl/aryl)aminocrotonates (36a-d), which furnished the four P[2,3-e]IZs (37a-d) (Scheme 22).52 Varying amounts of the corresponding furoindazoles were also formed (not shown here).
In continuation, the reaction of the same dioxoindazole with 3-enaminoketones was carried out, which similarly furnished the P[2,3-e]IZs (38a-c) (Scheme 23).
2.5 Synthesis of pyrrolo[3,2,1-hi]indazoles (V)
The anti-2,4-dinitrophenyl ethers (41a-f) of the ketoximes of 7-acyl/aroyl-indoles (39a-f) underwent base-catalysed cyclisation to lead to a novel series of P[3,2,1-hi]IZs (42a-f) in poor to good yields (Scheme 24).53 The alternative possible structures of the products resulting from initial Beckmann rearrangement, followed by cyclisation, were ruled out mainly from observed 1H-15N correlations (HSQC-HMBC NMR spectra) of the products. The anti-configuration of compounds 41 supports a displacement mechanism.
The PIZs 42a-f exhibited bright yellow fluorescence under long-wavelength UV light.
2.6 Synthesis of pyrrolo[1,2-b]indazoles (VI)
N-Heterocyclic carbene-induced cycloaddition reaction of indazoles with activated acetylenes furnished novel P[1,2-b]IZs. Thus, the treatment of the mesomeric betaine 1,2-dimethylindazolium-3-carboxylate (43) with methyl/ethyl 3-phenylpropiolates under thermal condition furnished 3,5-dihydro- 2H-P[1,2-b]IZs (44a,b) in low yields (Scheme 25).54
Analogously, the betaines 45a-c reacted with dimethyl and diethyl acetylenedicarboxylates (DMAD and DEAD, respectively) separately to furnish the 5,9b-dihydro-3H-P[1,2-b]IZ triesters (46a-d) as yellow oils. The reaction of 45a with DEAD furnished two by-products, one of which was a 9b-(substituted but-2-en-2-yl)-3H-P[1,2-b]IZ derivative (47) (Scheme 26).
2.7 Synthesis of pyrrolo[3,4-g]indazoles (VII)
It had earlier been shown that E-1-acetyl-3-(2-hydroxyphenyl)-5-styrylpyrazole (48a) reacts with N-methylmaleimide (NMM) to give the expected cycloadduct (49a) in poor yield along with by-products.55
In continuation, they treated the E/Z-styrylpyrazoles (48a-e) with NMM under microwave irradiation (MWI; 800 Watt) in a solvent-free manner to furnish an epimeric mixture (at C-5) of the adducts (49a-e from E-isomers and 50a’-c’ from Z-isomers). Treatment of both classes of products by DDQ led to aromatisation and N-deacetylation, furnishing the 6,8-dioxo-1H-P[3,4-g]IZs (51) (Scheme 27).56 When the reactions were carried out thermally (anhydrous 1,2,4-trichlorobenzene, DDQ, 170 ºC), it furnished the products (51) in better yields.
The cis-configurations of (i) H-5 and H-5a, H-5a and H-8a, and H-8a and H-8b in 49a-e and (ii) H-5a and H-8a, and H-8a and H-8b in 50a’-c’ were ascertained from the observed 1H-1H COSY correlations for 49 (H-4 to H-5a to H-8a to H-8b) and 50 (H-8a to H-5a to H-2’’,6’’).
The reaction of 48d (E-isomer) with N-phenylmaleimide (NPM) and DMAD separately under similar conditions as above provided the cycloadduct (52) as the major product and the oxidised PIZ 53 as the minor product from NPM and a trace amount of the corresponding conjugate addition product (54) from DMAD (Scheme 28). Throughout these studies, the products were characterised by 1H NMR, HSQC, HMBC and EI-MS data.
In continuation of their first report on the 1,3-dipolar cycloaddition (DCA) reactions of azomethine ylides with nitroarenes,57 Bastrakov et al. studied the DCA reactions of the known indazoles 57a-d with the unstabilised azomethine ylide 55. While 55 was generated in situ from sarcosine and paraformaldehyde in refluxing toluene,58 57a-d were prepared from the corresponding 4,6-dinitrophenylindazoles (56a,b). A single DCA reaction, followed by loss of nitrous acid, led to the formation of 6,8-dihydro-1H,7H-P[3,4-g]IZs (58a-d). An attempt to aromatise one of these PIZs (58a) by manganese dioxide led to the exclusive formation of the corresponding 6,8-dihydro-6,8 dioxo-1H,7H-P[3,4-g]IZ (59), albeit in low yield (Scheme 29).59,60
A novel class of 4,5-dihydro-1H,7H-P[3,4-g]IZs were synthesised regioselectively by the annulation of the pyrazole ring on the isoindole moiety using the 5-hydroxymethylenetetrahydroisoindol-4-ones (61a-f) as the key intermediates. Thus, when 61a-f, prepared from the corresponding tetrahydroisoindolones (60a-f),61,62 were separately treated with hydrazine hydrate or methyl/benzyl/4-methoxyphenylhydrazine, the 4,5-dihydro-P[3,4-g]IZs (62) were formed. Some of these dihydro-PIZs were oxidized by DDQ to the corresponding 1H,7H- or 2H,7H-P[2,3-g]IZs (63) (Scheme 30).63
When treated with methylhydrazine and benzylhydrazine separately, 61b furnished a mixture of the N(1)- and regioisomeric N(2)-substituted 4,5-dihydro-P[3,4-g]IZs (62) (Scheme 31).
In a similar manner, N-methyl/benzylisoindol-4-ones (60e,f) furnished, via the derived 5-methoxycarbonyl derivatives, 7-methyl/benzyl derivatives of 2-methyl-4,5-dihydro-2H,7H- P[3,4-g]IZ-3-ones (64a,b) (Scheme 32).
2.8 Synthesis of pyrrolo[3,2-g]indazoles (VIII)
8-Methyl derivatives of the then unknown 1(2)H,8H-P[3,2-g]IZs (65a,b) were synthesised by the heteroannulation of the 6-hydroxymethylene derivative of 1-methyl-4,5,6,7-tetrahydroindol-7-one, followed by aromatisation (Scheme 33).64 The new PIZs resemble the structures of the B and C subunits of the antitumor, pyrroloindole antibiotic CC-1065.65-67
In the same report, 3-carboxy-2,8-dimethyl-2H,8H-P[3,2-g]IZ (68), a product more closely related to two other naturally occurring pyrroloindoles PDE-I and PDE-II,68,69 was also synthesised from the same tetrahydroindolone (Scheme 34).
3. BIOACTIVITY OF PIZs
Amongst the eight classes of PIZs reported till date, only three classes, viz. the [2,3-g]-, [3,2-e]- and [3,4-g]-types displayed bioactivities of some kind. The rest were not reportedly tested for any biological activity.
Thus, some of the novel P[2,3-g]IZs, presented in Schemes 1-5, were claimed to be useful as non-addicting analgesics and anti-inflammatory agents in warm-blooded animals. They were active in antagonising the phenyl-p-quinone (PPQ) “writhing” syndrome.
When tested at oral doses of 100 or 200 mg/kg in mice, six PIZs (2b-d, 3a-c) proved to be active.38
None of the 4,5-dihydro-1H,6H-P[2,3-g]IZs 8a-d showed antibacterial (Candida albicans), antifungal (T. mentagraphite, M. cannis) or antiamoebic activity.40
The P[2,3-g]IZ carboxamide 12 showed acceptable NMDA-receptor antagonist activity in an assay using [3H-Ro-25-6981] as radioligand.42-44
Both the N-EOM-4,5-dihydro-P[2,3-g]IZs 13a and 13b showed significant inhibition of sGC. Indeed, the 6,7-dihydroindole ring present in 13a and 13b was considered to be a promising core structure for the inhibition of sGC, although 13b (R’ = Ph) transpired to be a superior inhibitor in the in vitro experiments.45
The three P[2,3-g]IZs 25-27 were tested for inhibition of Pim kinases. Of them, 26 was found to be the most active inhibitor of Pim kinase-1 (IC50 = 0.23 µM), while 27 transpired to be the most potent inhibitor of Pim-3 (IC50 = 33 nM), both at 10 µM concentrations. Based on molecular modeling experiments, the authors pointed out that the PIZ 27 can be used as a tool to study the biological role of Pim-3 compared to that of Pim-1 and Pim-2 and that the P[2,3-g]IZ scaffold could be used for the development of new potent inhibitors of Pim kinases.49
The P[3,2-e]IZ caboxamide 28 exhibited good NMDA-receptor antagonist activity.42
At 10 μM concentrations, the novel 4,5-dihydro-P[3,4-g]IZ-3-ones 62a (R = R2 = Me; R1 = CO2Et; R3 = 1-H), 62b (R = R2 = Me; R1 = CO2Et; R3 = 1-(4-MeOBn)) and 62c (R = Bn; R1 = H; R2 = Me; R3 = 1-H) exhibited modest in vitro antitumor properties against a panel of about 60 human tumour cell lines.63
4. CONCLUSION
This is the first review on the synthesis and bioactivity of all eight isomeric classes of PIZs, reported till date, which also includes a Sonogashira/Cacchi coupling-heteroannulation route to the general synthesis of 5-substituted P[3,2-e]IZs developed by the present authors. Although various isomeric PIZs have shown diverse bioactivities, the analgesic, anti-inflammatory and Pim kinase-inhibitory properties of P[2,3-g]IZs proved to be promising.
ACKNOWLEDGEMENTS
Financial support in the form of an Emeritus Scientistship (to M. C.) from the CSIR, Govt. of India, a Research Fellowship (to M. R.) from the CSIR, Govt. of India and financial support (to G. K. K.) from the Presidency University, Kolkata are gratefully acknowledged.
References
1. D. J. Mack, M. L. Weinrich, E. Vitaku, and J. T. Njardarson, http://cbc.arizona.edu/njardson/group/top-pharmaceuticals-poster.
2. C. A. Lipinsky, F. Lombardo, B. W. Dominy, and P. J. Feeney, Adv. Drug Deliv. Rev., 1997, 23, 3. CrossRef
3. M. J. Waring, Expert Opin. Drug Discov., 2010, 5, 235. CrossRef
4. A. Gomtsyan, Khim. Geterotsikl. Soed., 2012, 12; Chem. Heterocycl. Compd., 2012, 48, 7. CrossRef
5. J. J. Li, ‘Heterocyclic Chemistry in Drug Discovery’, John Wiley & Sons, Hoboken, New Jeresy, 2013.
6. A. F. Pozharsky, A. T. Soldatenkov, and A. R. Katritzky, ‘Heterocycles in Life and Society’, John Wiley & Sons, Chichester, U.K., 1997.
7. W. J. Houlihan, ‘Indoles’, Part One, 1st edn., John Wiley & Sons, New York, 1972.
8. R. J. Sandburg, ‘Indoles’, Academic Press, New York, 1996.
9. J. A. Joule and K. Mills, ‘Heterocyclic Chemistry’, 4th edn., Blackwell Science, Oxford, 2000, pp. 324-379.
10. V. Sharma, P. Kumar, and D. Pathak, J. Heterocycl. Chem., 2010, 47, 491.
11. R. Dhani, A. Avinash, S. K. Salenaagina, M. V. S. Teja, P. Masthanaiah, P. R. Rathnam, and V. C. Silpa, J. Chem. Pharm. Res., 2011, 3, 519.
12. T. C. Barden, Top. Heterocycl. Chem., 2011, 26, 31. CrossRef
13. F. R. de Sa Alves, E. J. Barreiro, and C. A. M. Fraga, Mini-Rev. Med. Chem., 2009, 9, 782. CrossRef
14. N. K. Kaushik, N. Kaushik, P. Attri, N. Kumar, C. H. Kim, A. K. Verma, and E. H. Choi, Molecules, 2013, 18, 6620. CrossRef
15. J. Elguero, ‘Comrehensive Heterocyclic Chemistry: Pyrazoles and their Benzo Derivatives’, Vol. 5, ed. by A. R. Katritzky and C. W. Rees, Pergamon Press, Oxford, 1984, pp. 167-303.
16. J. Elguero, ‘Comprehensive Heterocyclic Chemistry II: Pyrazoles’, Vol. 3, ed. by A. R. Katritzky, C. W. Rees, and E. F. Scriven, Pergamon Press, Oxford, 1996, pp. 1-75. CrossRef
17. L. Yet, ‘Comprehensive Heterocyclic Chemistry III: Pyrazoles’, Vol. 4, ed. by A. R. Katritzky, C. A. Ramsden, E. F. Scriven, and R. J. K. Taylor, Elsevier, Oxford, 2008, pp. 1-141. CrossRef
18. Atta-ur-Rahman, S. Malik, C. He, and J. Clardy, Tetrahedron Lett., 1985, 26, 2759.
19. Atta-ur-Rahman, S. Malik, S. S. Hasan, M. I. Choudhary, C.-Z. Ni, and J. Clardy, Tetrahedron Lett., 1995, 36, 1993.
20. Y.-M. Liu, J.-S. Yang, and Q.-H. Liu, Chem. Pharm. Bull., 2004, 52, 454. CrossRef
21. J. A. Balfour and S. P. Clissold, Drugs, 1990, 39, 575. CrossRef
22. N. H. Fankai and M. A. el-Nakeeb, J. Chemother., 1992, 4, 347.
23. J. Bermudez, C. S. Fake, and G. J. Sanger, J. Med. Chem., 1990, 33, 1924. CrossRef
24. H. Harada, T. Morie, Y. Hirokawa, H. Terauchi, I. N. Yoshida, and S. Kato, Chem. Pharm. Bull., 1995, 43, 1912. CrossRef
25. B. Silvestrini and C. Y. Cheng, US Patent 601865, 1999.
26. C. Y. Cheng, M.-y. Mo, J. M.-y. Grima, L. Saso, B. Tita, D. Mruk, and B. Silvestrini, Contraception, 2002, 65, 265. CrossRef
27. L. Ricotti, A. Tesei, F. De Paula, C. Milandri, D.Amadori, G. L. Frassineti, P. Ulivi, and W. Soli, J. Chemother., 2003, 15, 480.
28. M. K. Brawer, Rev. Urol., 2005, 7, S21.
29. S. C. Kuo, K. Y. Lee, and C. M. Teng, Eur. Patent EP667345 (Chem. Abstr., 1995, 123, 340113j).
30. C. C. Wu, F. N. Ko, S. C. Kuo, F. Y. Lee, and C. M. Teng, J. Pharmacol., 1995, 116, 1973.
31. E. J. Yeo, Y. S. Chun, Y. S. Cho, J. Kim, J. C. Lee, M. S. Kim, and J. W. Park, J. Natl. Cancer Inst., 2003, 95, 516. CrossRef
32. F. Tzanetou, S. Liekens, K. M. Kasiotis, N. Fokialakis, and S. A. Haroutounian, Arch. Pharm. Chem. Life Sci., 2012, 345, 804. CrossRef
33. N. Uchiyama, M. Kawamura, R. Kikura-Hanajiri, and Y. Goda, Forensic Toxicol., 2012, 30, 114. CrossRef
34. J. Amato, N. Iaccarino, B. Pagano, V. Compagnone, F. Di Rosa, G. Peluso, E. Novellino, and A. Randazzo, J. Forensic Sci. Criminol., 2014, 1, 1. CrossRef
35. H. Cerecetto, A. Gerpe, M. González, V. J. Arán, and C. O. de Ocáriz, Mini-Rev. Med. Chem., 2005, 5, 869. CrossRef
36. M. J. Haddadin, W. E. Conrad, and M. Kurth, Mini-Rev. Med. Chem., 2012, 12, 1293. CrossRef
37. N. A. S. Ali, B. A. Dar, V. Pradhan, and M. Farooqui, Mini-Rev. Med. Chem., 2013, 13, 1792. CrossRef
38. F. J. McEvoy, J. M. Smith, Jr., and D. S. Allen, Jr., US Patent 3, 404, 157, 1968.
39. N. Ya. Maksimov, V. P. Chetverikov, and A. N. Kost, US Patent 685664, 1979.
40. R. Singh, H. Bhagavateeswaran, P. C. Jain, and N. Anand, Indian J. Chem., 1982, 21B, 853.
41. S. Sequeria and S. Seshadri, Indian J. Chem., 1987, 26B, 436.
42. I. Borza, É. Bozó, G. Barta-Szalai, C. Kiss, G. Tárkányi, Á. Demeter, T. Gáti, V. Háda, S. Kolok, A. Gere, L. Fodor, J. Nagy, K. Galgóczy, I. Magdó, B. Ágai, J. Fetter, F. Bertha, G. M. Keserü, C. Horváth, S. Farkas, I. Greiner, and G. Domány, J. Med. Chem., 2007, 50, 901. CrossRef
43. V. Mutel, D. Buchy, A. Klingelschmidt, J. Messer, Z. Bleuel, J. A. Kemp, and J. G. Richards, J. Neurochem., 1998, 70, 2147. CrossRef
44. J. G. Richards, J. Messer, D. Buchy, A. Klingelschmidt, and V. Mutel, Br. J. Pharmacol., 1997, 120 (Suppl.), 39P.
45. K. Spyridonidou, M. Fousteris, M. Antonia, A. Chatzianastasiou, A. Papapetropoulos, and S. Nikolaropoulos, Bioorg. Med. Chem. Lett., 2009, 19, 4810. CrossRef
46. S. M. Bronner, A. E. Goetz, and N. K. Garg, Synlett, 2011, 2599.
47. S. M. Bronner, A. E. Goetz, and N. K. Garg, J. Am. Chem. Soc., 2011, 133, 3832. CrossRef
48. L. Gavara, F. Anizon, and P. Moreau, Tetrahedron, 2011, 67, 7330. CrossRef
49. L. Gavara, V. Suchaud, L. Nauton, V. Théry, F. Anizon, and P. Moreau, Bioorg. Med. Chem. Lett., 2013, 23, 2298. CrossRef
50. P. Cozzi, P. G. Baraldi, I. Beria, L. Capolongo, and G. Spalluto, GB Patent 2300857 A, 1996.
51. S. K. Barik, M. Rakshit, G. K. Kar, and M. Chakrabarty, ARKIVOC, 2014, v, 1. CrossRef
52. V. M. Lyubchanskaya, L. M. Alekseeva, S. A. Savina, and V. G. Granik, Chem. Heterocycl. Compd., 2000, 36, 1276. CrossRef
53. T. D. Wahyuningsih, K. Pchalek, N. Kumar, and D. StC. Black, Tetrahedron, 2006, 62, 6343. CrossRef
54. A. Schmidt, B. Snovydovych, and S. Hemmen, Eur. J. Org. Chem., 2008, 4313. CrossRef
55. V. L. M. Silva, A. M. S. Silva, D. C. G. A. Pinto, and J. A. S. Cavaleiro, Synlett, 2006, 1369. CrossRef
56. V. L. M. Silva, A. M. S. Silva, D. C. G. A. Pinto, J. Elguero, and J. A. S. Cavaleiro, Eur. J. Org. Chem., 2009, 4468. CrossRef
57. M. A. Bastrakov, A. M. Starosotnikov, S. Yu. Pechenkin, V. V. Kachala, I. V. Glukhov, and S. A. Shevelev, J. Heterocycl. Chem., 2010, 47, 893. CrossRef
58. O. Tsuge and S. Kanemasa, ‘Advances in Heterocyclic Chememistry: Recent Advances in Azomethine Ylide Chemistry’, Vol. 45, ed. by A. R. Katritzky, Elsevier, Oxford, 1989, pp. 231-349.
59. A. M. Starosotnikov, M. A. Bastrakov, S. Yu. Pechenkin, M. A. Leontieva, V. V. Kachala, and S. A. Shevelev, J. Heterocycl. Chem., 2011, 48, 824. CrossRef
60. A. M. Starosotnikov, D. V. Khakimo, M. A. Bastrakov, S. Yu. Pechenkin, S. A. Shevelev, and T. S. Pivina, Khim. Geterotsikl. Soed., 2011, 271; Chem. Heterocycl. Compd., 2011, 47, 215. CrossRef
61. P. Barraja, P. Diana, A. Montalbano, A. Carbone, G. Viola, G. Basso, A. Salvador, D. Vedaldi, F. Dall’Acqua, and G. Cirrincione, Bioorg. Med. Chem., 2011, 19, 2326. CrossRef
62. R. O. Clinton, A. J. Manson, F. W. Stonner, H. C. Neumann, R. G. Christiansen, R. L. Clarke, J. H. Ackerman, D. F. Page, J. W. Dean, W. B. Dickinson, and C. Carabateas, J. Am. Chem. Soc., 1961, 83, 1478. CrossRef
63. V. Spanò, A. Montalbano, A. Carbone, B. Parrino, P. Diana, G. Cirrincione, and P. Barraja, Tetrahedron, 2013, 69, 9839. CrossRef
64. S. Massa, G. Stefancich, M. Artico, F. Corelli, and R. Silvestri, Il Farmaco, 1985, 42, 567.
65. L. J. Hanka, A. Dietz, S. A. Gerpheide, S. L. Kuentzel, and D. G. Martin, J. Antibiot., 1978, 31, 1211. CrossRef
66. D. G. Martin, C. Biles, S. A. Gerpheide, L. J. Hanka, W. C. Krueger, J. P. McGovern, S. A. Mizsak, G. L. Neil, J. C. Stewart, and J. Visser, J. Antibiot., 1981, 34, 1119. CrossRef
67. V. L. Reynolds, J. P. McGovern, and L. H. Hurley, J. Antibiot., 1986, 39, 319. CrossRef
68. Y. Enomoto, Y. Furutani, H. Naganawa, M. Hamada, T. Takeuchi, and H. Umezawa, Agric. Biol. Chem., 1978, 42, 1331. CrossRef
69. H. Nakamura, Y. Enomoto, T. Takeuchi, H. Umezawa, and Y. Iitaka, Agric. Biol. Chem., 1978, 42, 1337. CrossRef