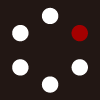
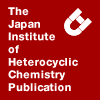
HETEROCYCLES
An International Journal for Reviews and Communications in Heterocyclic ChemistryWeb Edition ISSN: 1881-0942
Published online by The Japan Institute of Heterocyclic Chemistry
e-Journal
Full Text HTML
Received, 2nd June, 2015, Accepted, 15th October, 2015, Published online, 23rd October, 2015.
DOI: 10.3987/COM-15-13259
■ Iron(III) Tetranitrophthalocyanine Chloride Immobilized on Activated Carbon: Efficient, Excellent Chemoselectivity and Recyclable Catalyst for Synthesis of 2-Substituted Benzimidazoles
Jun Qiu, Yaodu Zhang, Chengwen Hua, Xiaofeng Gou, Bang Chen, and Junlong Zhao*
College of Chemistry & Materials Science, Northwest University, Xi’an, Shaanxi 710069, China
Abstract
The efficient and chemoselective preparation of 2-substituted benzimidazoles was established through the coupling of o-phenylenediamine with aldehydes by using iron(III) tetranitrophthalocyanine chloride immobilized on activated carbon as efficient catalyst in ethanol at room temperature. This method tolerated a variety of functional groups and had several advantages such as environmental friendliness, ease of manipulation, and a short reaction time. In addition, this catalyst can be recovered and reused for multiple cycles without loss in its catalytic activity.Among the various nitrogen containing heterocycles, benzimidazole derivatives exhibit antiviral, antiulcer, antihypertension, and anticancer properties.1 The benzimidazoles are biologically potent and this moiety is an important pharmacophore in drug discovery and also good intermediate for synthesis of many important organic compounds.2 There are two general methods for the synthesis of 2-substituted benzimidazoles. The one is the coupling of o-phenylenediamines and carboxylic acids3 or their derivatives (nitriles, imidates, or orthoesters),4 which often require strong acidic conditions and sometimes combines with very high temperatures (i.e., phenylpropanolamine, 180 ºC) or the use of microwave irradiation.5 These derivatives also often generated from the condensation of phenylenediamines and aldehydes under oxidative conditions using various oxidative and catalytic reagents, such as nitrobenzene (high-boiling point oxidant/solvent),6 Yb(OTf)3,7 CAN (ceric ammonium nitrate),8 FeCl3,9 (bromodimethyl)sulfonium bromide,10 ZrCl4,11 and Co(OH)2/CoO12 have been employed as the reagents or catalysts for the synthesis of benzimidazoles. Although the reaction was efficiently promoted by the above conditions they are often homogeneous catalysts and some of these methods suffer from one or more disadvantages, such as usage of stoichiometric or more quantity of reagent, high cost of the catalysts, prolonged reaction times, occurrence of several side reactions, severe reaction conditions, difficulty in separation of the products from the reaction mixture and strong oxidizing nature of the reagents. Therefore, the discovery of mild and practicable, stable, cheap, recyclable, and ecofriendly heterogeneous catalysts for the synthesis of 2-substituted benzimidazoles continues to attract the attention of researchers.
Metallophthalocyanines (MPcs) have many interesting properties and applications in several important technological fields,13 and they are very well known to catalyze a variety of organic reactions,14 so investigation into the redox properties of MPcs continue to be active areas of research.15 The driving forces for the catalytic use of phthalocyanines are (i) their economical and facile preparation in a large scale; (ii) the structure analogy with that of porphyrins, which are widely used by nature in the active sites of oxygenase enzymes; (iii) their chemical and thermal stability.16 Despite the various advantages, their separation from the reaction media is the main drawback from the viewpoint of the application in catalysis. This problem can be overcome by the immobilization of MPcs on activated carbon supports in order to make catalysts recoverable and reusable.
Thus, considering the above advantages and in continuation of our research work on designing recyclable heterogeneous catalytic systems for various organic transformations, herein we report the excellent chemoselectivity oxidative cyclization of o-phenylenediamine with aldehydes in presence of newly synthesized iron(III) tetranitrophthalocyanine chloride immobilized on activated carbon as recyclable catalyst using air as an oxidant.
In previous studies, efficient catalytic systems for metal phthalocyanines catalyzed synthesis of 3,4-dialkoxythiophenes through decarboxylation.15a In a continuation of our ongoing program on the
development of efficient, environmentally benign protocols for the synthesis of 2-substituted benzimidazoles, we report here the first example of oxidative cyclization reaction to synthesis of 2-substituted benzimidazoles catalyzed by iron(III) tetranitrophthalocyanine chloride immobilized on activated carbon in alcohol under 1 atmosphere pressure of air at room temperature (Scheme 1).
In terms of resource conservation, the selection of simple and cost-effective catalysts are also an important topic. Initially, we performed the oxidative cyclization of o-phenylenediamine with benzaldehyde in the presence of MPcs and air as an oxidant. The related results are listed in Table 1. In the control experiment, the oxidative cyclization could not occur without the metal phthalocyanine catalyst (Table 1, entry 1). During further catalyst screening, we found that MPcs could catalyze the reaction under air or oxygen atmosphere (Table 1, entries 2-9, 11), but only iron(III) phthalocyanine complexes have excellent chemoselectivity to synthesis of 2-substituted benzimidazole (Table 1, entries 4 and 11). Next, we prepared the iron(III) tetranitrophthalocyanine chloride immobilized on activated carbon, and catalyzed this reaction. We were pleasantly surprised to find that it exhibited higher chemical activity and selectivity of oxidative cyclization of o-phenylenediamine with benzaldehyde (Table 1, entry 12). Subsequently, we changed the amount of catalyst or the reaction time, the results show that they were little change compared with entry 12 (Table 1, entries 13-14). It is interesting that the iron(III) tetranitrophthalocyanine chloride immobilized on activated carbon catalyst was more reactive than the other MPcs, especially in the presence of a catalytic amount of acetic acid, giving good conversions at room temperature (Table 1, entry 15).
The oxidative cyclization could be accomplished with ease in the presence of a catalytic amount of iron(III) tetranitrophthalocyanine chloride immobilized on activated carbon stirred in alcohol at room temperature. The progress of the reaction was monitored by TLC using petroleum ether/ethyl acetate (4:1) as eluent, after completion of the reaction, the catalyst was filtered off, and the resulting solid was treated with ethanol and filtered again to recover the catalyst. The filtrate was added an equal volume of water, and natural crystallization. So the products could be easily separated from the system, in good yields and purities, using a facile filtration. In order to understand the scope and generality of the reaction, a wide range of aldehydes was subjected to the reaction with o-phenylenediamine under these reaction conditions (Table 2). All of the products are known compounds and characterized easily by comparison with authentic samples.8-12,17-20 As can be seen, the catalytic system worked exceedingly well in case of aromatic aldehydes with both electron-rich and electron-withdrawing groups, and giving excellent yields of the corresponding 2-substituted benzimidazoles.
The recovered catalyst is capable of being reused in subsequent cycles after washing with ethanol. Furthermore, we found that the recovered catalyst could be successfully used more than eight times without loss of catalytic activity and selectivity was observed compared with the fresh catalyst. The results obtained by recycling of the catalyst are shown in Table 3. The recycling result suggests heterogeneity of the catalyst.
According to the above experimental phenomena, a plausible reaction mechanism for the oxidative cyclization reaction using NO2-FePcCl/C as a catalyst has been proposed as shown in Scheme 2. First, the reaction proceeds via o-phenylenediamine with aldehyde condense followed by imine formation and this resulting imine further reacts with another -NH2 group of 1,2-phenylenediamine resulting in the formation of dihydrobenzimidazole 7. The catalytical cycle begins with the catalyst 1 combined with acetic acid to form complex 2. In the next step the replacement of the acetic acid with oxygen takes place affording 3, which combined with another catalyst 1 followed by oxidative cleavage give oxoferryl radical cation 4. The first electron transfer leads to formation of radical cation 8 which after remove a proton gives the radical 9. The oxoferryl radical cation 4 with subsequent protonation to form hydroxyferryl complex 5. By this process the iron(IV) does not change its oxidation state but free oxygen radical is paired and stabilized. The second electron transfer leads to formation of protonated imidazole 10 which after deprotonation gives the product I. The catalyst 1 is regenerated by reductive elimination of water molecule and thus closing the catalytic cycle.21
If the imine 6 continue to aldehydes condensation to double imine derivatives 11, it will be formed byproducts II. Therefore, this is a competitive reaction of single imide oxidative cyclization and double imine derivatives formation. If the double imine derivatives formative rate more than single imide oxidative cyclization, it will generate 1,2-disubstituted benzimidazoles. This catalyst can activate molecular oxygen, and have fast rate and high efficiency to oxidation of dihydrobenzimidazoles 7, thus avoiding the formation of byproducts II.
EXPERIMENTAL
1H NMR spectra were measured on an INOVA-400 spectrometer at 400 MHz. NMR spectra were obtained on solution in CDCl3 using TMS as internal standard, the chemical shifts are relative to TMS. Infrared spectra (KBr) were recorded on BRUKER EQUINOX55 FT-IR spectrometer. Mass spectra were recorded on a BRUKER micrOTOF-QII Mass spectrometer. The melting points were determined using XT-4 melting point microscope apparatus.
Starting Materials. All chemicals were commercially available and used without further purification. All the phthalocyanine complexes were prepared according to the methods described in the literatures.22-24
General procedure for the synthesis of 2-substituted benzimidazoles. The o-phenylenediamine (1.0 mmol) was dissolved in EtOH 95% (10 mL), and added aldehydes (1.0 mmol), two drops of acetic acid and catalyst (2 mg) were magnetically stirred in the reaction flask at room temperature. The progress of the reaction was monitored by TLC using petroleum ether/EtOAc (4:1) as eluent. The reactions were stopped after 1 h. After completion of the reaction, the catalyst was separated from the reaction mixture by filtration, the filtrate was added an equal volume of water, natural crystallization, filtration, and dried to give the products. The all products of Table 2 were confirmed from the ESI-MS, IR and 1H NMR.
Benzimidazole (a). White solid; mp 170-172 ºC; ESI-MS: m/z (C7H6N2) 119.0607 (calcd. for [M+H]+ 119.0609); IR cm-1: 3443, 1619, 1585, 1456; 1H NMR (400 MHz; DMSO-d6) δ: 12.46 (s, 1H), 7.18 (m, 8H).
2-Phenyl-1H-benzimidazole (b). Pale yellow solid; mp 294-296 ºC; ESI-MS: m/z (C13H10N2) 195.0934 (calcd. for [M+H]+ 195.0922); IR cm-1: 3047, 1805, 1587, 1540; 1H NMR (400 MHz; DMSO-d6) δ: 12.90 (s, 1H), 7.19-8.18 (m, 9H).
2-(2-Fluorophenyl)-1H-benzimidazole (c). Brown solid; mp 206-208 ºC; ESI-MS: m/z (C13H9N2F) 213.0845 (calcd. for [M+H]+ 213.0828); IR cm-1: 3443, 1620, 1583, 1464; 1H NMR (400 MHz; DMSO-d6) δ: 12.69 (s, 1H), 7.22-8.27 (m, 8H).
2-(3-Fluorophenyl)-1H-benzimidazole (d). Pale yellow solid; mp 255-257 ºC; ESI-MS: m/z (C13H9N2F) 213.0845 (calcd. for [M+H]+ 213.0828); IR cm-1: 3051, 1615, 1587, 1485; 1H NMR (400 MHz; DMSO-d6) δ: 13.03 (s, 1H), 7.20-8.05 (m, 8H).
2-(4-Fluorophenyl)-1H-benzimidazole (e). Pale yellow solid; mp 253-255 ºC; ESI-MS: m/z (C13H9N2F) 213.0853 (calcd. for [M+H]+ 213.0828); IR cm-1: 3445, 1597, 1497; 1H NMR (400 MHz; DMSO-d6) δ: 12.93 (s, 1H), 7.17-8.24 (m, 8H).
2-(4-Chlorophenyl)-1H-benzimidazole (f). Pale yellow solid; mp 292-294 ºC; ESI-MS: m/z (C13H9N2Cl) 229.0549 (calcd. for [M+H]+ 229.0533); IR cm-1: 3444, 1589, 1429; 1H NMR (400 MHz; DMSO-d6) δ: 13.01 (s, 1H), 7.21-8.21 (m, 8H).
2-(3-Bromophenyl)-1H-benzimidazole (g). White solid; mp 296-300 ºC; ESI-MS: m/z (C13H9N2Br) 273.0039 (calcd. for [M+H]+ 273.0027); IR cm-1: 3445, 1647, 1561, 1540, 1439; 1H NMR (400 MHz; DMSO-d6) δ: 13.05 (s, 1H), 7.20-8.37 (m, 8H).
2-(2,4-Dichlorophenyl)-1H-benzimidazole (h). Pale yellow solid; mp 226-228 ºC; ESI-MS: m/z (C13H8N2Cl2) 263.0164 (calcd. for [M+H]+ 263.0143); IR cm-1: 3102, 1590, 1557, 1423; 1H NMR (400 MHz; DMSO-d6) δ: 12.79 (s, 1H), 7.21-7.93 (m, 7H).
2-(2-Hydroxyphenyl)-1H-benzimidazole (i). White solid; mp 241-243 ºC; ESI-MS: m/z (C13H10N2O) 211.0881 (calcd. for [M+H]+ 211.0871); IR cm-1: 3235, 1627, 1590, 1488; 1H NMR (400 MHz; DMSO-d6) δ: 13.23 (s, 1H), 13.17 (s, 1H), 7.01-8.07 (m, 8H).
2-(4-Hydroxyphenyl)-1H-benzimidazole (j). Brown solid; mp 277-279 ºC; ESI-MS: m/z (C13H10N2O) 211.0883 (calcd. for [M+H]+ 211.0871); IR cm-1: 3604, 3450, 1608, 1564, 1496; 1H NMR (400 MHz; DMSO-d6) δ: 12.66 (s, 1H), 9.98 (s, 1H), 6.19-8.02 (m, 8H).
2-(4-Methoxyphenyl)-1H-benzimidazole (k). Pale yellow solid; mp 225-227 ºC; ESI-MS: m/z (C14H12N2O) 225.1041 (calcd. for [M+H]+ 225.1028); IR cm-1: 3743, 1614, 1582, 1500; 1H NMR (400 MHz; DMSO-d6) δ: 12.75 (s, 1H), 7.10-8.13 (m, 8H), 3.84 (s, 3H).
2-(3-Methoxy-4-hydroxyphenyl)-1H-benzimidazole (l). Pale yellow solid; mp 221-222 ºC; ESI-MS: m/z (C14H12N2O2) 241.0992 (calcd. for [M+H]+ 241.0977); IR cm-1: 3539, 3318, 1595, 1503; 1H NMR (400 MHz; DMSO-d6) δ: 12.66 (s, 1H), 9.55 (s, 1H), 6.91-7.75 (m, 8H), 3.89 (s, 3H).
2-(3-Hydroxy-4-methoxyphenyl)-1H-benzimidazole (m). Red-brown solid; mp 222-225 ºC; ESI-MS: m/z (C14H12N2O2) 241.0992 (calcd. for [M+H]+ 241.0977); IR cm-1: 3608, 3271, 1616, 1588, 1502; 1H NMR (400 MHz; DMSO-d6) δ: 12.68 (s, 1H), 9.32 (s, 1H), 7.08-7.63 (m, 8H), 3.85 (s, 3H).
2-(4-Dimethylaminophenyl)-1H-benzimidazole (n). Pale yellow solid; mp 232-234 ºC; ESI-MS: m/z (C15H15N3) 238.1363 (calcd. for [M+H]+ 238.1344); IR cm-1: 3416, 1610, 1503, 1443; 1H NMR (400 MHz; DMSO-d6) δ: 12.53 (s, 1H), 6.82-8.00 (m, 8H), 3.00 (s, 6H).
2-(3-Nitrophenyl)-1H-benzimidazole (o). White solid; mp 200-202 ºC; ESI-MS: m/z (C13H9N3O2) 240.0789 (calcd. for [M+H]+ 240.0773); IR cm-1: 3457, 1685, 1623, 1437, 1520, 1347; 1H NMR (400 MHz; DMSO-d6) δ: 13.32 (s, 1H), 7.23-9.03 (m, 8H).
2-(4-Nitrophenyl)-1H-benzimidazole (p). Yellow solid; mp > 300 ºC; ESI-MS: m/z (C13H9N3O2) 240.0791 (calcd. for [M+H]+ 240.0773); IR cm-1: 3035, 1601, 1514, 1435, 1338; 1H NMR (400 MHz; DMSO-d6) δ: 13.32 (s, 1H), 7.26-8.43 (m, 8H).
2-(2-Furanyl)-1H-benzimidazole (q). Brown solid; mp 281-284 ºC; ESI-MS: m/z (C11H8N2O) 185.0721 (calcd. for [M+H]+ 185.0715); IR cm-1: 3442, 1628, 1587, 1521; 1H NMR (400 MHz; DMSO-d6) δ: 12.93 (s, 1H), 6.74-7.96 (m, 7H).
5-Nitro-2-phenylbenzimidazole (r). Orange solid; mp 208-209 ºC; ESI-MS: m/z (C13H9N3O2) 240.0761 (calcd. for [M+H]+ 240.0768); IR cm-1: 3423, 1642, 1563, 1538, 1328; 1H NMR (400 MHz; DMSO-d6) δ: 13.60 (s, 1H), 8.47-7.55 (m, 8H).
ACKNOWLEDGEMENTS
We gratefully acknowledge financial support from the Industrial Research Project of Shaanxi Science and Technology Department (2014K08-29), National Basic Science Personnel Training Fund (J1103311 and J1210057) and Scientific Research Foundation of Northwestern University.
References
1. (a) J. Bauer, S. Kinast, A. Burtge-Kentischer, D. Finkelmeier, G. Kleymann, W. A. Rayyan, A. Singh, G. Jung, S. Rupp, and H. Eickhoff, J. Med. Chem., 2011, 54, 6993; CrossRef (b) D. A. Horton, G. T. Bourne, and M. L. Smythe, Chem. Rev., 2003, 103, 893. CrossRef
2. (a) S. M. Sondhi, N. Singh, A. Kumar, O. Lozach, and L. Meijer, Bioorg. Med. Chem., 2006, 14, 3758; CrossRef (b) J. Vinsova, K. Cermakova, A. Tomeckova, M. Ceckova, J. Jampilek, P. Cermak, J. Kunes, M. Dolezal, and F. Staud, Bioorg. Med. Chem., 2006, 14, 5850; CrossRef (c) R. Trivedi, S. K. De, and R. A. Gibbs, J. Mol. Catal. A, 2006, 245, 8. CrossRef
3. J. B. Wright, Chem. Rev., 1951, 48, 397. CrossRef
4. T. A. Fairley, R. R. Tidwell, I. Donkor, N. A. Naiman, K. A. Ohemeng, R. J. Lombardy, J. A. Bentley, and M. Cory, J. Med. Chem., 1993, 36, 1746. CrossRef
5. K. Bougrin, A. Loupy, and M. Soufiaoui, Tetrahedron, 1998, 54, 8055. CrossRef
6. R. S. Harapanhalli, L. W. McLaughlin, R. W. Howell, D. V. Rao, S. J. Adelstein, and A. I. Kassis, J. Med. Chem., 1996, 39, 4804. CrossRef
7. C. Massimo, E. Francesco, and M. Francesca, Synlett, 2004, 1832. CrossRef
8. K. Bahrami, M. M. Khodaei, and F. Naali, J. Org. Chem., 2008, 73, 6835. CrossRef
9. M. A. Alibeik and M. Moosavifard, Synth. Commun., 2010, 40, 2686. CrossRef
10. B. Das, H. Holla, and Y. Srinivas, Tetrahedron Lett., 2007, 48, 61. CrossRef
11. Z. H. Zhang, L. Yin, and Y. M. Wang, Catal. Commun., 2007, 8, 1126. CrossRef
12. M. A. Chari, D. Shobha, and T. Sasaki, Tetrahedron Lett., 2011, 52, 5575. CrossRef
13. (a) S. L. Jain, J. K. Joseph, S. Singhal, and B. Sain, J. Mol. Catal. A, 2007, 268, 134; CrossRef (b) J. Zhou and Q. Sun, J. Am. Chem. Soc., 2011, 133, 15113; CrossRef (c) J. T. F. Lau, P. C. Lo, Y. M. Tsang, W. P. Fong, and D. K. P. Ng, Chem. Commun., 2011, 47, 9657. CrossRef
14. (a) J. L. Zhao, X. F. Gou, C. W. Hua, and L. Y. Wang, Chin. J. Catal., 2012, 33, 1262; CrossRef (b) Ü. Salan, N. Kobayashi, and Ö. Bekaroglu, Tetrahedron Lett., 2009, 50, 6775; CrossRef (c) I. M. Geraskin, M. W. Luedtke, H. M. Neu, V. N. Nemykin, and V. V. Zhdankin, Tetrahedron Lett., 2008, 49, 7410; CrossRef (d) A. A. Lamar and K. M. Nicholas, Tetrahedron, 2009, 65, 3829; CrossRef (e) L. X. Alvarez, E. V. Kudrik, and A. B. Sorokin, Chem. Eur. J., 2011, 17, 9298; CrossRef (f) E. Kockrick, T. Lescouet, E. V. Kudrik, A. B. Sorokin, and D. Farrusseng, Chem. Commun., 2011, 47, 1562; CrossRef (g) W. M. Li, A. P. Yu, D. C. Higgins, B. G. Llanos, and Z. W. Chen, J. Am. Chem. Soc., 2010, 132, 17056; CrossRef (h) D. L. Usanov and H. Yamamoto, Angew. Chem. Int. Ed., 2010, 49, 8169. CrossRef
15. (a) A. B. P. Lever, J. Porphyrins Phthalocyanines, 1999, 3, 488; CrossRef (b) J. Mack and N. Kobayashi, Chem. Rev., 2011, 111, 281; CrossRef (c) M. J. Stillman, J. Porphyrins Phthalocyanines, 2000, 4, 374; CrossRef (d) T. Nyokong and H. Isago, J. Porphyrins Phthalocyanines, 2004, 8, 1083. CrossRef
16. R. K. Sharma, S. Gulati, and S. Sachdeva, Green Chem. Lett. Rev., 2012, 5, 83. CrossRef
17. Y. C. Lin, N. C. Li, and Y. J. Cherng, J. Heterocycl. Chem., 2014, 51, 808. CrossRef
18. S. D. Pardeshi, J. P. Sonar, S. S. Pawar, D. Dekhane, S. Gupta, A. M. Zine, and S. N. Thore, J. Chil. Chem. Soc., 2014, 59, 2335. CrossRef
19. J. A. Gladysz, H. Reza Safaei, and S. Nouri, Helv. Chim. Acta, 2014, 97, 1539. CrossRef
20. S. S. Panda and S. C. Jain, Synth. Commun., 2011, 41, 729. CrossRef
21. (a) P. Afanasiev, E. V. Kudrik, F. Albrieux, V. Briois, O. I. Koifman, and A. B. Sorokin, Chem. Commun., 2012, 48, 6088; CrossRef (b) A. B. Sorokin and E. V. Kudrik, Catal. Today, 2011, 159, 37; CrossRef (c) H. Mahdi, M. Hamid, S. Nasser, and M. M. Anahita, J. Porphyrins Phthalocyanines, 2012, 16, 93; CrossRef (d) F. L. Mirela, L. Mladen, and V. Vladimir, Bioorg. Med. Chem., 2008, 16, 9276. CrossRef
22. A. Burczyk, A. Loupy, D. Bogdala, and A. Petitb, Tetrahedron, 2005, 61, 179. CrossRef
23. M. P. Somashekarappa and J. Keshavayya, Synth. React. Inorg. Met.-Org. Chem., 2001, 31, 811.
24. J. L. Zhao, M. Wang, C. Q. Cheng, X. F. Gou, and C. W. Hua, CN Patent, 103272643A, 2013.