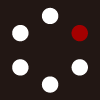
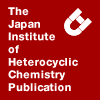
HETEROCYCLES
An International Journal for Reviews and Communications in Heterocyclic ChemistryWeb Edition ISSN: 1881-0942
Published online by The Japan Institute of Heterocyclic Chemistry
e-Journal
Full Text HTML
Received, 6th September, 2015, Accepted, 21st October, 2015, Published online, 26th October, 2015.
DOI: 10.3987/COM-15-13318
■ Bifurans via Palladium-Catalyzed Suzuki Coupling
Jun Zhang, Peijun Ye, Lu He, Ting Yuan, and Qiancai Liu*
School of Chemistry and Molecular Engineering, East China Normal University, Shanghai 200241, China
Abstract
Sixteen 2,2’-bifurans with aryl substituents are reported. The copper-mediated oxidative coupling of lithium salt of furan led to the formation of 2,2’-bifuran, which was brominated with either 2 eq. or 4 eq. NBS to afford 5,5’-dibromo-2,2’-bifuran and 3,3’,5,5’-tetrabromo-2,2’-bifuran. The palladium- catalyzed Suzuki reactions between dibromo or tetrabormo-bifuran and arylboronic acid were employed to furnish the title compounds, which were characterized by NMR spectra and mass spectra.Benzofuran-based molecules have attracted numerous attention for their potentials in materials chemistry. Among which small organic molecules and polymers based on arene-fused difurans are widely used in OLED, OPV and solar cells.1 In contrast to benzofuran-containing molecules, α-oligofurans have called less attention, although they can act as an emerging class of conjugated oligomers for organic electronics. The recent progress in the study of long α-oligofurans in the point of their synthesis and characterization has been reviewed.2
Synthesis of short oligofurans began with the initial synthesis of 2F and 4F using the Ullmann reaction by Kauffmann in 1981, and there was only one example of a substituted α-oligofuran consisting of five rings before a series of long α-oligofurans (Scheme 1) were reported by Gidron et al. and some others from the Stille coupling of α,ω-dibromooligofurans with monostannyloligofurans in good yields. Later substituted 5F was obtained from a Negishi-type coupling reaction.3-5 A Stille coupling to synthesize 3F was also applied by Ishida et al.6 Approaches to longer oligofurans resulted in unstable and inseparable mixtures,7 the enhanced solubility of the intermediates could secure the synthesis of very long, unsubstituted oligofurans up to 9F.4 Zhang et al. reported a different approach to substituted oligofurans by a Co(II)-based metallo-radical catalyst from a new type of tandem radical cyclization of acetylenes with substituted diazo-reagents, and a series of specifically functionalized oligofurans up to four rings were prepared by this method.8
However oligomeric furans are still limited. Most of reported examples are approached by Stille coupling from halogenated furan and furan-stannane, or homocoupling of furanstannane.3-6,9 Since Bendikov and others have already indicated the potential application of diaryl-substituted bifurans in organic electronics, it is worthy to explore a more convenient method with lower toxic substrates.10 No report about substituted bifurans prepared directly from Suzuki coupling was found, which might be due to the limited availability of the key precursor 5,5’-dibromo-2,2’-bifuran (2). Although 2,2’-bifuran (1) has been reported long time ago,11 we found that copper-mediated oxidative coupling of furan is troublesome, the low yield might be due to the high volatility of 2,2’-bifuran, which could be distilled along with solvents during workup step. Therefore cautious handling is needed to avoid the loss of this compound. 5,5’-Dibromo-2,2’-bifuran (2) is easily synthesized by bromination of 1 with NBS in benzene. However compound 2 is quite light-sensitive at solid state, which gradually changes from white to yellow then deep blue, it is necessary to handle it with strict absence of light. In contrast to 2, compound 3 is quite stable to air and light, and it could easily prepared by bromination of 1 with 4 equivalent NBS.3,6
We envisaged that the targeted bifurans with different aryl substituents might be obtained by Suzuki coupling of 2 with different arylboronic acids since we have reported some heterocyclic molecules by this method. Then the Suzuki couplings of 2 with eight different arylboronic acid have been performed with Pd(PPh3)4 (10 mol%), the target molecules 4a-h could be separated in the yield of 43% to 66%. However, when the same condition was applied to the precursor 3, only a complicated mixture was formed, which could not be separated. Inspired by our previous experience on naphthyridine-based molecules by [(A-taPhos)2PdCl2] (CAS No. 887919-35-9, Pd-132), which is highly efficient for Suzuki cross coupling reactions between arylboronic acids and naphthyridine bromide,12 we believe that Pd-132 might be a suitable catalyst for the Suzuki coupling to reach 5a-h. The experimental for the reaction of compound 3 and phenylboronic acid indicates that this is quite practical, and it is pleased to find that all reactions of selected arylboronic acids with 3 could proceed with moderate to excellent yields (39% to 93%) with a low catalyst loading (ca. 1.5%) (Scheme 2).
In conclusion, we developed an easy and simple synthetic method towards bifurans with two or four aryl substituents. The Ullmann coupling of furan was employed to afford 2,2’-bifuran, which was converted into key intermediates 2 and 3. The palladium-catalyzed Suzuki coupling between 2 or 3 and arylboronic acid was employed to approach target molecules with either Pd(PPh3)4 or Pd-132 as a catalyst. The target molecules are quite soluble and stable in common organic solvents.
EXPERIMENTAL
All chemical are commercially available as analytic grade and used without further purification. Solvents are purified according to standard methods prior to use. 1H NMR and 13C NMR spectra were measured on a Bruker Avance III 500 MHz spectrometer (1H NMR 500 MHz, 13C NMR 125 MHz). Mass spectra (EI) were measured on Bruker Daltonics maXis Impact spectrometer. Melting point was measured on an X-4 micrographic melting point measuring instrument. Mass spectra (ESI) were measured on micOTOF II.
Synthetic procedures
2,2’-Bifuran (1) and 5,5’-dibromo-2,2’-bifuran (2) and 3,3’,5,5’-tetrabromo-2,2’-bifuran (3) are prepared by literature methods (see supplementary materials for details).3,6
General procedure for 5,5’-diaryl-2,2’-bifuran (4a-h)
A three-necked round flask was charged with 5,5’-dibromo-2,2’-bifuran (0.10 g, 0.34 mmol) and arylboronic acid (0.70 mmol), K2CO3 (0.19 g, 1.75 mmol), freshly distilled THF (20 mL) and distilled water (5 mL). The mixture was purged with nitrogen for three times before Pd(PPh3)4 (81 mg, 0.069 mmol) was added. The resulting mixture was refluxed for 12 h and the TLC monitoring indicated the reaction was completed. Brine was added to quench the reaction before it was extracted with CH2Cl2 (3 x 50 mL). The organic phase was washed with water (2 x 50 mL) and the solvents were removed under vacuum. The residue was purified by column separation to afford target molecule.
5,5’-Diphenyl-2,2’-bifuran (4a), pale yellow solid (42 mg, 43%), mp 173-174 oC. Rf 0.16 (PE). 1H NMR δ: 7.74 (d, J = 7.9 Hz, 4H), 7.41 (t, J = 7.2 Hz, 4H), 7.29 (d, J = 7.2 Hz, 2H), 6.79-6.70 (m, 4H). 13C NMR δ: 153.27, 145.98, 130.49, 128.73, 127.47, 123.76, 107.42, 107.03. HR-MS (ESI) required for C20H14O2: 286.0988, found 286.0995 (M+H)+.
5,5’-Bis(2-methylphenyl)-2,2’-bifuran (4b), pale yellow solid (50 mg, 47%), mp 139-140 oC. Rf 0.17 (PE). 1H NMR δ: 7.77 (d, J = 7.7 Hz, 2H), 7.29 (d, J = 7.3 Hz, 4H), 7.25-7.19 (m, 2H), 6.74 (s, 2H), 6.64 (s, 2H), 2.56 (s, 6H). 13C NMR (125 MHz, CDCl3) δ: 152.94, 145.70, 134.57, 131.30, 129.83, 127.56, 126.92, 126.08, 110.45, 107.06, 22.06. HR-MS (ESI) required for C22H18NaO2: 337.1199, found 337.1201 (M+Na)+.
5,5’-Bis(4-methylphenyl)-2,2’-bifuran (4c), pale yellow solid (50 mg, 47%), mp 127-128 oC. Rf 0.21 (PE). 1H NMR δ: 7.65 (d, J = 8.2 Hz, 4H), 7.24 (d, J = 7.9 Hz, 4H), 6.71 (q, J = 3.5 Hz, 4H), 2.40 (s, 6H). 13C NMR δ: 153.43, 145.74, 137.32, 129.41, 127.88, 123.73, 107.19, 106.29, 21.33. HR-MS (ESI) required for C22H18O2: 314.1307, found 314.1301 (M+H)+.
5,5’-Bis(2-chlorophenyl)-2,2’-bifuran (4d), pale yellow solid (66 mg, 55%), mp 169-170 oC. Rf 0.45 (PE). 1H NMR δ: 7.96 (dd, J = 7.9, 1.2 Hz, 2H), 7.46 (d, J = 7.9 Hz, 2H), 7.36 (t, J = 7.5 Hz, 2H), 7.22 (td, J = 8.0, 1.3 Hz, 4H), 6.79 (d, J = 3.6 Hz, 2H). 13C NMR δ: 149.68, 145.68, 130.82, 130.09, 128.79, 128.12, 127.76, 126.94, 113.00, 107.89. HR-MS (ESI) required for C20H12O2Cl2Na: 377.0107, found 377.0114 (M+Na)+.
5,5’-Bis(4-butylphenyl)-2,2’-bifuran (4e), pale yellow solid (123 mg, 62%), mp 133-134 oC. Rf 0.35 (PE). 1H NMR δ: 7.66 (d, J = 8.2 Hz, 4H), 7.24 (d, J = 8.2 Hz, 4H), 6.71 (q, J = 3.5 Hz, 4H), 2.69-2.61 (m, 4H), 1.64 (t, J = 7.7 Hz, 4H), 1.40 (dd, J = 14.9, 7.4 Hz, 4H), 0.96 (t, J = 7.4 Hz, 6H). 13C NMR δ: 153.46, 145.76, 142.40, 128.78, 128.08, 123.74, 107.18, 106.30, 35.47, 33.56, 22.35, 13.97. HR-MS (ESI) required for C28H30O2: 398.2240, found 398.2238 (M+H)+.
5,5’-Bis(2-thienyl)-2,2’-bifuran (4f), pale yellow solid (62 mg, (55%), mp 153-154 oC. Rf 0.20 (PE). 1H NMR δ: 7.34 (dd, J = 3.6, 1.0 Hz, 2H), 7.27 (dd, J = 5.0, 1.0 Hz, 2H), 7.08 (dd, J = 5.0, 3.6 Hz, 2H), 6.70 (d, J = 3.5 Hz, 2H), 6.60 (d, J = 3.5 Hz, 2H). 13C NMR δ: 148.90, 145.28, 133.37, 127.74, 124.35, 122.83, 107.48, 107.07. HR-MS (ESI) required for C16H10O2S2: 298.0116, found 298.0117 (M+H)+.
5,5’-Bis(2-naphthyl)-2,2’bifuran (4g), pale yellow solid (87 mg, 66%), mp 170-171 oC. Rf 0.32 (PE). 1H NMR δ: 8.52 (d, J = 8.4 Hz, 2H), 7.91 (d, J = 7.6 Hz, 2H), 7.89-7.80 (m, 4H), 7.62-7.51 (m, 6H), 6.86 (dd, J = 10.7, 3.4 Hz, 4H). 13C NMR δ: 152.88, 146.45, 134.07, 130.30, 128.68, 128.16 (s), 126.74, 126.10, 125.45, 111.29, 107.28. HR-MS (ESI) required for C28H18NaO2: 409.1199, found 409.1207 (M+Na)+.
5,5’-Bis(4-phenoxyphenyl)-2,2’bifuran (4h), pale yellow solid (100 mg, 65%), mp 133-134 oC. Rf 0.34 (PE). 1H NMR (500 MHz, CDCl3) δ: 7.71 (dd, J = 15.4, 8.7 Hz, 2H), 7.62 (d, J = 8.6 Hz, 1H), 7.52 (d, J = 8.8 Hz, 1H), 7.38-7.34 (m, 3H), 7.28 (t, J = 8.0 Hz, 1H), 7.16-7.03 (m, 10H), 6.96 (d, J = 8.7 Hz, 1H), 6.76-6.62 (m, 3H). 13C NMR (125 MHz, CDCl3) δ: 195.92, 157.01, 156.92, 151.34, 195.92, 129.96, 195.92, 128.32, 195.92, 125.61, 218.28, 124.98, 123.76, 123.34, 119.28, 118.82, 118.60, 107.31, 106.31. HR-MS (ESI) required for C32H22O4: 470.1513, found 470.1525 (M+H)+.
General procedure for 3,3’,5,5’-tetrakis(aryl)-2,2’-bifuran (5a-h)
A three-necked round flask was charged with 3,3’,5,5’-dibromo-2,2’-bifuran (40 mg, 0.09 mmol) and arylboronic acid (0.36 mmol), Cs2CO3 (293 mg, 0.90 mmol), freshly distilled THF (20 mL) and distilled water (5 mL). The mixture was purged with nitrogen for three times before Pd-132 (1 mg, 0.0014 mmol) was added. The resulting mixture was refluxed for 12 h and the TLC monitoring indicated the reaction was completed. Brine was added to quench the reaction before it was extracted with CH2Cl2 (3 x 50 mL). The organic phase was washed with water (2 x 50 mL) and the solvents were removed under vacuum. The residue was purified by column separation to afford target molecule.
3,3’,5,5’-Tetraphenyl-2,2’-bifuran (5a), yellow solid (35 mg, 90%), mp 77-178 oC. Rf 0.25 (PE). 1H NMR δ: 7.53 (t, J = 7.7 Hz, 8H), 7.32 (dt, J = 28.8, 9.6 Hz, 12H), 6.90 (s, 2H). 13C NMR δ: 153.37, 140.18, 133.18, 130.19, 128.68, 128.26, 127.72, 127.25, 127.01, 123.86, 108.04. HR-MS (ESI) required for C32H22O4: 438.1614, found 438.1623 (M+H)+.
3,3’,5,5’-Tetra(2-methylphenyl)-2,2’-bifuran (5b), pale-green solid (35 mg, 79%), mp 181-182 oC. Rf 0.35 (PE). 1H NMR δ: 7.38-7.26 (m, 8H), 7.11 (dd, J = 22.1, 8.1 Hz, 8H), 6.60 (s, 2H), 2.31 (s, 6H), 2.24 (s, 6H). 13C NMR δ: 152.21, 141.06, 136.87, 134.15, 133.59, 131.12, 130.21, 130.00, 129.32, 127.47, 127.27, 126.33, 125.91, 125.65, 124.39, 112.99, 22.05, 20.26. HR-MS (ESI) required for C36H30O2: 494.2240, found 494.2240 (M+H)+.
3,3’,5,5’-Tetra(4-methylphenyl)-2,2’-bifuran (5c), pale-green solid (41 mg, 93%), mp 195-196 oC. Rf 0.32 (PE). 1H NMR δ: 7.45 (d, J = 8.1 Hz, 4H), 7.40 (d, J = 8.0 Hz, 4H), 7.17-7.11 (m, 8H), 6.84 (s, 2H), 2.36 (d, J = 4.3 Hz, 12H). 13C NMR δ: 153.45, 139.72, 137.50, 136.82, 130.31, 129.33, 128.87, 128.03, 127.67, 126.88, 123.86, 107.17, 21.28. HR-MS (ESI) required for C36H30O2: 494.224, found 494.2260 (M+H)+.
3,3’,5,5’-Tetra(2-chlorophenyl)-2,2’-bifuran (5d), pale green solid (20 mg, 39%), mp 188-189 oC. Rf 0.27 (PE:EtOAc = 20:1). 1H NMR δ: 7.48 (dd, J = 10.5, 4.1 Hz, 4H), 7.40-7.27 (m, 8H), 7.19 (dd, J = 6.7, 2.5 Hz, 2H), 7.15-7.09 (m, 4H). 13C NMR δ: 149.20, 141.47, 133.88, 132.59, 131.83, 130.66, 129.90, 129.66, 128.80, 128.31, 128.10, 127.39, 126.65, 122.99, 115.15. MS (EI) required for C32H18Cl4O2: 576, found 575 (M-H)+ .
3,3’,5,5’-Tetra(4-butylphenyl)-2,2’-bifuran (5e), pale-green solid (30 mg, 50%), mp 201-202 oC. Rf 0.33 (PE). 1H NMR δ: 7.65 (dd, J = 8.1, 5.1 Hz, 6H), 7.23 (dd, J = 10.8, 8.2 Hz, 6H), 6.72-6.65 (m, 6H), 2.66 (dt, J = 25.3, 7.7 Hz, 7H), 1.66-1.59 (m, 6H), 1.40-1.34 (m, 7H), 0.94 (t, J = 7.4 Hz, 6H), 0.88 (dd, J = 16.3, 9.4 Hz, 10H). 13C NMR δ: 153.47, 145.77, 143.72, 142.40, 128.78, 128.32, 127.98, 123.78, 107.19, 106.31, 35.47, 33.56, 31.95, 29.72, 29.38, 28.71, 22.71, 22.35, 15.53, 14.14, 13.97. MS (EI) required for C48H54O2: 663, found 664 (M+H)+.
3,3’,5,5’-Tetra(2-thienyl)-2,2’-bifuran (5f), pale-yellow solid (30 mg, 73%), mp 103-104 oC. Rf 0.40 (PE). 1H NMR δ: 7.35 (d, J = 2.0 Hz, 2H), 7.29 (d, J = 4.7 Hz, 3H), 7.23 (d, J = 4.7 Hz, 2H), 7.11-7.00 (m, 5H), 6.84 (s, 2H). 13C NMR δ: 149.85, 137.60, 133.98, 132.75, 127.83, 127.32, 125.69, 125.22, 123.79, 122.67, 106.97. HR-MS (ESI) required for C24H14NaO2S4: 484.9769, found 484.9778 (M+Na)+.
3,3’,5,5’-Tetra(2-naphthyl)-2,2’bifuran (5g), pale-green solid (24 mg, 42%), mp 231-232 oC. Rf 0.18 (PE:EtOAc=10:1). 1H NMR δ: 8.26 (d, J = 8.6 Hz, 1H), 7.98 (d, J = 8.3 Hz, 1H), 7.83 (dd, J = 12.1, 8.1 Hz, 2H), 7.75 (d, J = 8.2 Hz, 1H), 7.69 (d, J = 8.2 Hz, 1H), 7.53-7.39 (m, 5H), 7.33 (dd, J = 15.5, 8.2 Hz, 3H), 7.17 (d, J = 7.2 Hz, 1H), 6.94 (s, 1H). 13C NMR δ: 152.74, 142.22, 133.90, 133.64, 131.99, 131.09, 129.67, 128.53, 128.24, 127.74, 127.45, 126.65, 125.85, 125.41, 125.06, 124.02, 114.39. HR-MS (ESI) required for C48H30O2: 638.2240, found 638.2255 (M+H)+.
3,3’,5,5’-Tetrakis(4-phenoxyphenyl)-2,2’-bifuran (5h), yellow solid (36 mg, 64% from 0.07 mmol 3 and 0.20 mmol 4-phenoxyphenylboronic acid), mp 141-142 oC. Rf 0.37 (PE:EtOAc = 10:1). 1H NMR δ: 7.55 (d, J = 7.8 Hz, 4H), 7.47 (d, J = 7.6 Hz, 4H), 7.40-7.28 (m, 8H), 7.16-6.96 (m, 20H), 6.79 (s, 2H). 13C NMR δ: 157.29, 156.66, 152.80, 152.46, 145.77, 140.27, 130.28, 129.84, 128.24, 125.72, 125.51, 125.12, 123.89, 123.51, 119.07, 118.61 (s), 108.93, 108.19, 106.08. HR-MS (ESI) or EI could not be obtained.
ACKNOWLEDGEMENTS
We thank the “Daxia” fund for undergraduate student training program of ECNU(2014DX-265), the national innovative program for undergraduate student (201510269036) and the large instrumental open foundation of East China Normal University for financial support.
References
1. (a) A. Facchetti, Chem. Mater., 2011, 23, 733; CrossRef (b) A. R. Murphy and J. M. J. Frechet, Chem. Rev., 2007, 107, 1066; CrossRef (c) “Organic Field-Effect Transistors”, ed. by Z. Bao and J. Locklin, CRC Press: Boca Raton, FL, 2007; CrossRef (d) “Organic Electronics, Manufacturing and Applications”, ed. by H. Klauk, Wiley-VCH: Weinheim, Germany, 2006. CrossRef
2. (a) O. Gidron and M. Bendikov, Angew. Chem. Int. Ed., 2014, 53, 2546; CrossRef (b) U. H. F. Bunz, Angew. Chem. Int. Ed., 2010, 49, 5037. CrossRef
3. T. Kauffmann and H. Lexy, Chem. Ber., 1981, 114, 3667. CrossRef
4. (a) O. Gidron, Y. Diskin-Posner, and M. Bendikov, J. Am. Chem. Soc., 2010, 132, 2148; CrossRef (b) Y. Miyata, T. Nishinaga, and K. Komatsu, J. Org. Chem., 2005, 70, 1147; CrossRef (c) Y. Miyata, M. Terayama, T. Minari, T. Nishinaga, T. Nemoto, S. Isoda, and K. Komatsu, Chem. Asian J., 2007, 2, 1492; CrossRef (d) F.-T. Luo and A. C. Bajji, J. Chin. Chem. Soc., 2000, 47, 257. CrossRef
5. J. M. Kauffman and G. Moyna, J. Heterocycl. Chem., 2002, 39, 981. CrossRef
6. H. Ishida, K. Yui, Y. Aso, T. Otsubo, and F. Ogura, Bull. Chem. Soc. Jpn., 1990, 63, 2828. CrossRef
7. G. Distefano, D. Jones, M. Guerra, L. Favaretto, A. Modelli, and G. Mengoli, J. Phys. Chem., 1991, 95, 9746. CrossRef
8. X. Cui, X. Xu, L. Wojtas, M. M. Kim, and X. P. Zhang, J. Am. Chem. Soc., 2012, 134, 19981. CrossRef
9. (a) S.-K. Kang, E.-Y. Namkoong, and T. Yamaguchi, Synth. Commun., 1997, 27, 641; CrossRef (b) J. P. Parrish, V. L. Flanders, R. J. Floyd, and K. W. Jung, Tetrahedron Lett., 2001, 42, 7229; CrossRef (c) S.-K. Kang, T.-G. Baik, X. H. Jiao, and Y.-T. Le, Tetrahedron Lett., 1999, 40, 2383. CrossRef
10. (a) O. Gidron, N. Varsano, L. J. W. Shimon, G. Leitus, and M. Bendikov, Chem. Commun., 2013, 49, 6256; CrossRef (b) O. Gidron, A. Dadvand, Y. Sheynin, W. Bendikov, and D. F. Perepichka, Chem. Commun., 2011, 47, 1976; CrossRef (c) S. Zhen, B. Lu, J. Xu, S. Zhang, and Y. Li, RSC Adv., 2014, 4, 14001; CrossRef (d) X.-H. Jin, D. Sheberla, L. J. W. Shimon, and M. Bendikov, J. Am. Chem. Soc., 2014, 136, 2592. CrossRef
11. (a) Kauffmann, B. Greving, R. Kriegesmann, A. Mitschker, and A. Woltermann, Chem. Ber., 1978 111, 1330; CrossRef (b) C.-S. Chang and W. H. Hwang, J. Chin. Chem. Soc., 1989, 36, 251. CrossRef
12. H. Ge and Q. Liu, Heterocycles, 2015, 91, 1001. CrossRef