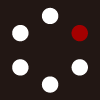
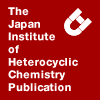
HETEROCYCLES
An International Journal for Reviews and Communications in Heterocyclic ChemistryWeb Edition ISSN: 1881-0942
Published online by The Japan Institute of Heterocyclic Chemistry
e-Journal
Full Text HTML
Received, 21st November, 2015, Accepted, 22nd January, 2016, Published online, 28th January, 2016.
DOI: 10.3987/COM-15-13379
■ Preparation of (+)-Biotin: Process Development and Scale-up
Fei Xiong,* Jin Li, Gen Li, Bo Song, Yu-Xiao Zhou, Fen-Er Chen, and Dan Li
Department of Chemistry, Fudan University, 220 Handan Road, Shanghai 200433, China
Abstract
A practical asymmetric total synthesis of (+)-biotin based upon the chiral bifunctional sulfamide 8-promoted enantioselective anhydride desymmetrization has been achieved via the key chiral intermediacy of (3aS,6aR)-1,3-bis(4-methoxybenzyl)tetrahydro-4H-thieno[3,4-d]imidazole-2,4(1H)-dione (4). In addition, the installation of the 4-carboxybutyl side chain at C-4 position of 4 was efficiently introduced by an improved C4+C1 strategy.The Roche lactone-thiolactone strategy for the synthesis of (+)-biotin originally described by Goldberg and Sternbach in 19511-3 has proven to be the most efficient method so far.4-7 It is thus not surprising that over the past decades, the major interest has been focused on developing commercially viable approach to prepare the optically pure Roche lactone, which has been obtained by various methods involving optical resolution4-7 and more recently, via asymmetric synthesis.8-17 Despite the progress made since the report of the original patent description, there are very few reports that relate to the deprotection (debenzylation) of N-benzylated imidazolido-thiophanes by reaction with hydrobromic acid13 or a mixture of sulfuric and glacial acetic acid10,18 in the final step of the total synthesis. Even then, many of these procedures suffer from one or more disadvantages such as prolonged reaction time, poor yields, and use of environmentally unfriendly reagents. Therefore, the design of an efficient and convenient method for the cleavage of the protective group (usually benzyl) of N,N-diprotected (as dibenzyl) biotin to (+)-biotin or the use of an alternate and more readily removable protecting group instead of the classical benzyl group is still a desirable target for the preparation of (+)-biotin on a large scale.
A complete literature search (Chemical Abstracts) revealed that removal of the N-(p-methoxybenzyl) group could be readily accomplished by treatment with potassium persulfate19 or ceric ammonium nitrate.20 In connection with our sustained interest in the synthesis of (+)-biotin, we recently reported the preparation of cis-1,3-bis(4-methoxybenzyl)tetrahydro-2H-furo[3,4-d]imidazole-2,4,6-trione (1).21 On the basis of the previously reported success of the cleavage of N-(p-methoxybenzyl) groups,19,20 it was anticipated that the use p-methoxybenzyl groups (and presumably their facile removal) in N,N-bis-(p- methoxybenzyl)biotin to (+)-biotin might constitute an improved procedure. We now report the success- ful execution of this new procedure to (+)-biotin, albeit removal of the p-methoxybenzyl group did not occur as anticipated from the literature reports.
Our improved process started with the generation of chiral building block (4S,5R)-hemiester 2, the direct precursor to (3aS,6aR)-lactone 3, via the asymmetric alcoholysis of cis-1,3-bis-(p-methoxy- benzyl)tetrahydro-2H-furo[3,4-d]imidazole-2,4,6-trione (1) (Scheme 1). The use of the chiral amines (Cinchona alkaloids)-based sulfonamide12 as the organocatalyst to mediate the nucleophilic ring-opening of the prochiral cyclic anhydride facilitated the highly enantioselective desymmetrization of 1 with cinnamyl alcohol compared to existing methods9-13,15-18 in terms of enantioselectivity, rapid reaction times and the practicality on large-scale production. Inspired by this potential approach and our continued interest to discover other chiral amine scaffolds, we first investigated the nucleophilic opening of 1 in the presence of alkamine-derived bifunctional sulfamide 8 (110 mol%), which was synthesized from a novel chiral source (1S,2S)-2-amino-1-(p-nitrophenyl)propane-1,3-diol,22 a by-product in the industrial production of chloramphenicol. As expected, the asymmetric desymmetrization reaction proceeded readily in methyl tert-butyl ether (MTBE) at room temperature to afford the desired (4S,5R)-hemiester 2 in nearly quantitative yield in 80% ee after 5 min (Table 1, entry 1). Encouraged by these promising results, a range of various reaction conditions (solvent, catalyst loading and concentration) were then screened in the presence of this chiral sulfamide catalyst 8, and the results are illustrated in Table 1.
A number of solvents were examined (Table 1, entries 1-4), the optimum solvent with high level of enantiocontrol was aprotic, H-bonding accepting solvent dioxane. Furthermore, it was found that decrease the catalyst loading had a significant negative influence on the enantioselectivity (Table 1, entries 3, 5-7). In contrast to the remarkable catalyst loading effect, the change of the reaction concentration of 1 in the dioxane (from 0.15 to 0.05 M) led only to subtle variation in the enantioselectivities (Table 1, entries 3, 8-9). It is also noteworthy that chiral bifunctional sulfamide catalyst 8 was easily recovered quantitatively by a simple 2M HCl extraction followed by basification of its aqueous hydrochloride salt solution and it could be reused without purification.
Reduction of the crude 2 to the sodium salt of the corresponding (4S,5R)-hydroxy acid (after alkaline saponification with 10% aqueous sodium carbonate) followed by lactonization using 5% hydrochloric acid gave 3 in 99% yield and 91% ee. The enantiomeric purity of 3 was easily improved to 98% by one recrystallization from ethanol. Subsequently (3aS,6aR)-lactone 3 was subjected to thiolactonization by treatment with potassium thioacetate in refluxing dimethylformamide for 40 min under argon atmosphere to provide the desired intermediate (3aS,6aR)-thiolactone 4 in 87% yield. It was also rewarding that cinnamyl alcohol was easily recovered quantitatively by evaporation of the separated organic phase at the stage of alkaline treatment with 10% aqueous sodium carbonate.
Although our earlier procedure involving the generation of the di-Grignard from 1,4-dibromobutane and carboxylation with carbon dioxide18 to install the ω-carboxybutyl side-chain at the C-4 position of the chiral thiolactone 4, under these reaction conditions, only a 75% conversion to the hydroxy acid was observed possibly because of the low solubility of Grignard reagent in THF at the cryogenic temperature (-25 oC). A significant improvement in the conversion 4 to the hydroxy acid (100%) was achieved when toluene was added as a co-solvent to the reaction mixture. Dehydration of the hydroxy acid was performed using 30% H2SO4 in THF at reflux to afford pentanoic acid 5, which upon catalytic hydrogenation over 10% Pd/C in 2-propanol at 120 oC and 60 atm (882 psi), gave N,N-bis(p-methoxybenzyl)biotin (6). Surprisingly, attempts to deprotect 6 with potassium persulfate or ceric ammonium nitrate under the reported conditions19,20 gave none of the expected (+)-biotin (7). Fortunately, the target molecule [(+)-biotin (7)] was obtained via a one-pot simultaneous deprotection and ring-opening of 6 using 47% aqueous HBr followed by in situ treatment of the resulting dihydrobromide salt of diamine with triphosgene in 83% yield after recrystallization from deionized water. The NMR and mass spectra for 7 were in agreement with those of authentic (+)-biotin.18
In summary, we have developed an improved and commercially scalable process for the stereocontrolled total synthesis of (+)-biotin from the known cis-1,3-bis(p-methoxybenzyl)tetrahydro-2H-furo[3,4-d]- imidazole-2,4,6-trione (1) in high purity and satisfactory overall yield (54%) from 1. Although we have successfully carried out this sequence on a kilogram scale (e. g. starting with 1.39 kg of 1), this article describes only the laboratory scale preparation of (+)-biotin starting with about 10 g of 1.
EXPERIMENTAL
Melting points were determined with a WRS-1B digital melting point apparatus and are uncorrected. NMR spectra were recorded on a Bruker Avance 400 spectrometer using TMS as an internal standard. Chemical shifts (δ) are expressed in ppm. Optical rotations were measured with a JASCO P1020 digital automatic polarimeter. HPLC analysis conditions: mobile phase A: 85% H3PO4 (0.85 mL) and K2HPO4 (2.7 g) dissolved in 1000 mL H2O; mobile phase B: acetonitrile; gradient elution. Unless otherwise noted, all the reagents were obtained from commercial sources and used as received.
(3aS,6aR)-1,3-bis(4-Methoxybenzyl)tetrahydro-4H-furo[3,4-d]imidazole-2,4(1H)-dione (3). trans-Cinnamyl alcohol (17.0 g, 16.2 mL, 126.5 mmol) was added dropwise to a suspension of 1 (10.0 g, 25.3 mmol) and chiral bifunctional sulfonamide catalyst 8 (21.0 g, 27.8 mmol) in dioxane (253 mL) under nitrogen atmosphere at 25 oC, and the mixture was stirred at this temperature for 5 min. Removal of the solvent in vacuo gave a residue which was dissolved in EtOAc (100 mL) and washed with 2M HCl (3 × 50 mL). The separated organic phase was washed with brine, dried over MgSO4 and evaporated to give the crude (4S,5R)-hemiester 2, which was used in the next step without purification.
To recover the chiral bifunctional sulfonamide catalyst 8, the collected acidic aqueous phase was basified with 2 M NaOH and extracted with CH2Cl2 (3 × 50 mL). The combined organic layers were dried over MgSO4 and evaporated to give the organocatalyst in quantitative yield, which could be reused directly without purification.
To a solution of 2 (13.4 g, 25.3 mmol) and anhydrous powdered CaCl2 (2.8 g, 25.3 mmol) in EtOH (130 mL) was added KBH4 (4.1 g, 75.9 mmol) in three portions at 0 oC and the reaction mixture was then allowed to warm up to 25 oC. After stirring for 18 h, the resulting mixture was concentrated and the residue was dissolved in EtOAc (80 mL). The solution was then extracted with 10% aqueous Na2CO3 (3 × 50 mL) and the combined aqueous layers were treated with 5% HCl (106 mL) at 60 oC for 30 min. After cooling, the mixture was extracted with CH2Cl2 (3 × 80 mL). The combined organic layers were washed with H2O (80 mL), dried over MgSO4, and the solvent was evaporated to give the crude product which was then purified by recrystallization from EtOH to afford the pure 3 (8.91 g, 92%) as a white solid in 99% purity, mp 110-111 oC (lit.21 mp 110.6-110.9 oC).
1H NMR (CDCl3): δ 7.346-7.368 (d, 2H, J = 8.8 Hz), 7.207-7.209 (d, 2H, J = 8.8 Hz), 6.907-6.928 (d, 4H, J = 8.4 Hz), 5.002-5.038 (d, 1H, J = 14.4 Hz), 4.574-4.612 (d, 1H, J = 15.2 Hz), 4.323-4.360 (d, 2H, J = 14.8 Hz), 4.116-4.193 (m, 3H), 3.926-3.948 (d, 1H, J = 8.8 Hz), 3.847 (s, 3H), 3.844 (s, 3H). 13C NMR (CDCl3): δ 172.401, 158.907, 129.195, 127.469, 113.697, 76.603, 76.282, 69.897, 45.987, 44.264. IR (KBr): 3525, 2954, 2832, 1770, 1699, 1687, 1513, 1237, 1253, 1030, 818 cm-1. MS (ESI): m/z = 405.0 (M+Na+).
To recover the trans-cinnamyl alcohol, the collected EtOAc layer was dried over MgSO4 and evaporated to give the trans-cinnamyl alcohol in quantitative yield.
(3aS,6aR)-1,3-bis(4-Methoxybenzyl)tetrahydro-4H-thieno[3,4-d]imidazole-2,4(1H)-dione (4). (3aS,6aR)-Lactone 3 (8.91 g, 23.3 mmol), potassium thioacetate (3.1 g, 26.8 mmol) were added to DMF (10 mL) at 25 oC under a nitrogen atmosphere. The reaction mixture heated to 150 oC and stirred for 45 min. After completion of the reaction as monitored by TLC, the resulting mixture was poured into H2O (250 mL) and extracted with CH2Cl2 (3 × 70 mL). The combined organic layers were then washed with H2O (200 mL), dried over MgSO4, and concentrated under reduced pressure to give the crude product, which was purified by recrystallization from 2-propanol to afford product 4 (8.07 g, 87%) as an off-white crystalline powder, mp 126-127 oC (lit.21 mp 125-126 oC).
1H NMR (CDCl3): δ 7.308-7.330 (d, 2H, J = 8.8 Hz), 7.247-7.269 (d, 2H, J = 8.8 Hz), 6.906-6.928 (d, 2H, J = 8.4 Hz), 6.890-6.912 (d, 2H, J = 8.8 Hz), 4.989-5.025 (d, 1H, J = 14.4 Hz), 4.649-4.686 (d, 1H, J = 14.8 Hz), 4.294-4.349 (m, 2H), 4.118-4.157 (m, 1H), 3.819-3.849 (m, 1H), 3.849 (s, 3H), 3.839 (s, 3H), 3.308-3.430 (m, 2H). 13C NMR (CDCl3): δ 203.145, 157.804, 127.779, 113.610, 76.280, 61.635, 54.861, 44.263, 32.747. IR (KBr): 3361, 2932, 2832, 1692, 1510, 1448, 1245, 1213, 1029, 824 cm-1.
MS (ESI): m/z = 421.1 (M+Na+).
(3aS,6aR)-[1,3-bis(4-Methoxybenzyl)tetrahydro-2(3H)-oxo-1H-thieno[3,4-d]imidazol-4-yl]pentanoic Acid (5). Under the atmosphere of argon, 1,4-dibromobutane (0.4 mL, 3.5 mmol) was added into the suspension of magnesium (5.6 g, 232.7 mmol) in anhydrous THF (48 mL) at 35 oC. After the reaction had started, the remaining 1,4-dibromobutane (13.0 mL, 110.0 mmol) in THF (60 mL) was added dropwise over a period of 1 h and stirred at reflux for another 2 h. The solution of 4 (8.07 g, 20.3 mmol) in anhydrous THF (25 mL) and toluene (50 mL) was then added dropwise into the freshly prepared di-Grignard reagent at -25 oC within 30 min followed by stirring at this temperature for 3 h. Then dry gaseous carbon dioxide was bubbled into the resulting mixture for 3 h at -25 oC. After being allowed to warm up to room temperature, the mixture was quenched with saturated aqueous NH4Cl (150 mL) and the mixture was extracted with toluene (3 × 100 mL). The combined organic extracts were washed with brine (80 mL) and concentrated in vacuo to afford a yellow oily residue, which was subjected to dehydration by treatment with 30% H2SO4 (40 mL) in refluxing THF (20 mL). After being stirred under reflux for 0.5 h, the reaction mixture was extracted with EtOAc (80 mL) and the separated organic layer was concentrated under reduced pressure. The residue was neutralized with 10% aqueous Na2CO3 (80 mL) and extracted with EtOAc (3 × 80 mL). The pH of the aqueous phase to 5.5 by addition of 2 M HCl then extracted with EtOAc (3 × 80 mL). The combined organic extracts were washed successively with brine (50 mL) and H2O (50 mL), dried over MgSO4, and concentrated in vacuo to afford 5 (7.93 g, 81%, 99% pure by HPLC) as a yellow oil.
Anal. Calcd for C26H30N2O5S: C, 64.71; H, 6.27; N, 5.80; S, 6.64. Found: C, 64.67; H, 6.34; N, 5.74; S, 6.63. 1H NMR (CDCl3): δ 7.264-7.286 (d, 2H, J = 8.8 Hz), 7.200-7.222 (d, 2H, J = 8.8 Hz), 6.905-6.927 (d, 2H, J = 8.8 Hz), 6.901-6.923 (d, 2H, J = 8.8 Hz), 5.468-5.503 (t, 1H, J = 7.2 Hz), 4.921-4.959 (d, 1H, J = 15.2 Hz), 4.746-4.784 (d, 1H, J = 15.2 Hz), 4.188-4.297 (m, 2H), 4.059-4.101 (m, 1H), 3.977-4.016 (d, 1H, J = 15.6 Hz), 3.849 (s, 3H), 3.843 (s, 3H), 2.950-3.033 (m, 2H), 2.353-2.391 (t, 2H, J = 7.6 Hz), 2.111-2.228 (m, 2H), 1.721-1.793 (m, 2H). 13C NMR (CDCl3): δ 177.502, 158.485, 137.828, 128.566, 124.840, 113.650, 76.279, 64.038, 58.454, 54.860, 45.499, 43.857, 36.670, 32.788, 30.588, 23.577. IR (KBr): 3433, 2938, 1717, 1652, 1515, 1477, 1258, 1240, 1172 cm-1. MS (ESI): m/z = 505.1 (M+Na+).
(+)-Biotin.- A suspension of 10% Pd/C (1.2 g), 5 (7.93 g, 16.5 mmol), and H2O (7 mL) in 2-propanol (38 mL) was stirred at 120 oC under hydrogen pressure of 60 atm (882 psi) for 20 h then filtered. The filtrate was concentrated under reduced pressure to give the product 6, which was subjected to next step without further purification.
A mixture of 6 (7.99 g, 16.5 mmol) and 47% HBr (53 mL) was heated to reflux for 36 h then extracted with toluene (3 × 10 mL). The aqueous phase was concentrated under reduced pressure. After dissolution of the residue in water (26 mL), a solution of triphosgene (14.7 g, 49.5 mmol) in anisole (100 mL) was added dropwise at 35 oC over a period of 2 h. The pH of the reaction mixture was maintained at 10 by simultaneous addition of 40% aqueous KOH. After 12 h at this temperature, the separated aqueous phase was acidified to pH1 with conc. HCl and cooled to 0 oC. The precipitated crystals were collected and recrystallized from deionized water to give pure (+)-biotin (3.35 g, 83%) as colorless crystals, mp 232-233 oC ([α]D22.1 +91.2 (c 1.0, 0.1 N NaOH); lit.18 mp 230-232 oC, [α]D22 +91.1 (c 1.0, 0.1 N NaOH)).
Anal. Calcd for C10H16N2O3S: C, 49.16; H, 6.60; N, 11.47; S, 13.12. Found: C, 49.01; H, 6.55; N, 11.55; S, 13.20. 1H NMR (CDCl3): δ 11.9 (s, 1H), 6.40 (s, 1H), 6.33 (s, 1H), 4.30 (dd, 1H, J = 7.6, 5.2 Hz), 4.11-4.15 (m, 1H), 3.07-3.12 (m, 1H), 2.82 (dd, 1H, J = 12.4, 5.2 Hz), 2.57 (d, 1H, J = 12.4 Hz), 2.20 (t, 2H, J = 7.6 Hz), 1.41-1.62 (m, 4H), 1.30-1.37 (m, 2H). 13C NMR (CDCl3): δ 174.8, 163.1, 61.5, 59.6, 55.8, 40.0, 33.9, 28.57, 28.52, 25.0. IR (KBr): 3359, 3308, 2961, 2469, 1941, 1707, 1480, 1318, 1270, 1154, 1015, 842, 753, 651 cm-1. MS (ESI): m/z = 283.2 (M+K+).
ACKNOWLEDGEMENTS
The authors gratefully acknowledge the financial support of the Shanghai Municipal Education Commission (No. SLG14033); the research project of teachers’ teaching development of University of Shanghai for Science and Technology for the year of 2016 and the open project program of Hubei Key Laboratory of Drug Synthesis and Optimization, JingChu University of Technology (No. OPP2014ZD01).
References
1. M. W. Goldberg and L. H. Sternbach, US Pat 2489232, 1949 (Chem. Abstr., 1951, 45, 184).
2. M. W. Goldberg and L. H. Sternbach, US Pat 2489235, 1949 (Chem. Abstr., 1951, 45, 186s).
3. M. W. Goldberg and L. H. Sternbach, US Pat 2489235, 1949 (Chem. Abstr., 1951, 45, 186g).
4. P. J. De Clercq, Chem. Rev., 1997, 97, 1755. CrossRef
5. M. Seki, Med. Res. Rev., 2006, 26, 434. CrossRef
6. B. Xie and F. E. Chen, Chin. J. Med. Chem., 2008, 18, 468 (Chem. Abstr., 2009, 153, 313361).
7. Z. Zhong, X. F. Wu, and F. E. Chen, Chin. J. Org. Chem., 2012, 32, 1792 (Chem. Abstr., 2012, 158, 272707). CrossRef
8. C. Choi, S. K. Tian, and L. Deng, Synthesis, 2001, 1737. CrossRef
9. J. Huang, F. Xiong, and F. E. Chen, Tetrahedron: Asymmetry, 2008, 19, 1436. CrossRef
10. H. F. Dai, W. X. Chen, L. Zhao, F. Xiong, H. Sheng, and F. E. Chen, Adv. Synth. Catal., 2008, 350, 1635. CrossRef
11. S. X. Wang and F. E. Chen, Adv. Synth. Catal., 2009, 351, 547. CrossRef
12. F. Xiong, X. X. Chen, and F. E. Chen, Tetrahedron: Asymmetry, 2010, 21, 665. CrossRef
13. X. X. Chen, F. Xiong, H. Fu, Z. Q. Liu, and F. E. Chen, Chem. Pharm. Bull., 2011, 59, 488. CrossRef
14. M. Yoshimura, K. Tsuda, H. Nakatsuka, and T. Yamamura, Tetrahedron, 2011, 67, 10006. CrossRef
15. F. Xiong, F. J. Xiong, W. X. Chen, H. Q. Jia, and F. E. Chen, J. Heterocycl. Chem., 2013, 50, 1078.
16. F. Xiong, X. K. Li, M. Xu, S. P. Zhang, F. E. Chen, B. Liao, H. J. Hua, and J. H. He, Asian J. Chem., 2014, 26, 3659.
17. F. Xiong, Z. Y. Shen, X. K. Li, Y. N. Peng, H. Y. Zhu, S. P. Zhang, Y. Y. Song, and J. L. Du, Org. Prep. Proced. Int., 2015, 47, 242. CrossRef
18. F. E. Chen, H. F. Dai, Y. Y. Kuang, and H. Q. Jia, Tetrahedron: Asymmetry, 2003, 14, 3667. CrossRef
19. G. Gerona-Navarro, M. A. Bonache, R. Herranz, M. T. Garcia-Lopez, and R. Gonzalez-Muniz, J. Org. Chem., 2001, 66, 3538. CrossRef
20. H. Ishibashi, C. Kameoka, H. Iriyama, K. Kodama, T. Sato, and M. Ikeda, J. Org. Chem., 1995, 60, 1276. CrossRef
21. D. Li, F. J. Xiong, F. Xiong, and F. E. Chen, Chin. J. Pharm., 2014, 45, 919 (Chem. Abstr., 2015, 180854).
22. H. J. Yang, F. J. Xiong, J. Li, and F. E. Chen, Chin. Chem. Lett., 2013, 24, 553. CrossRef