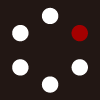
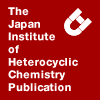
HETEROCYCLES
An International Journal for Reviews and Communications in Heterocyclic ChemistryWeb Edition ISSN: 1881-0942
Published online by The Japan Institute of Heterocyclic Chemistry
e-Journal
Full Text HTML
Received, 27th November, 2015, Accepted, 16th December, 2015, Published online, 24th December, 2015.
■ Experimental Investigation of Tetracyclic Compounds Containing a Nine-Membered Sultam via Cobalt Alkyne Complexes
Kyosuke Kaneda,* Misuzu Fujita, and Risa Naruse
Hokkaido Pharmaceutical University, 7-Jo 15-4-1 Maeda,Teine,Sapporo,Hokkaido 006-8590, Japan
Abstract
The synthesis, reactivity, and stereochemistry of nine-membered sultams fused with cobalt alkyne complexes using sequential Nicholas and Pauson–Khand reactions are reported. Novel tetracyclic compounds containing nine-membered sultam moieties were characterized by NMR spectroscopy and X-ray crystallography.introduction
Dicobalt hexacarbonyl alkyne complex-mediated cyclization reactions are among the methods available to construct medium-sized rings and bicyclic systems.1 The Nicholas2 and Pauson–Khand (PK) reactions3 have been extensively studied and remain of great importance in this respect, with applications in the synthesis of structurally complex molecules, including bioactive natural products.4 In addition, studies on the combined use of the Nicholas and PK reactions have been attempted by several groups.5 The Shea group reported the scope and limitations of this approach for the synthesis of medium-sized heterocyclic ring systems, wherein they demonstrated difficulty in constructing a nitrogen-embedded, nine-membered ring framework.5a Recently, our group successfully constructed a sulfonamide-embedded, nine-membered ring compound fused with dicobalt hexacarbonyl moiety B, which was synthesized from dicobalt alkyne complex A by a BF3-induced Nicholas reaction.6 As a result of the bent and strained structure of B, a subsequent intramolecular PK reaction occurred upon heating without the addition of any reagents to afford tetracycle C in sufficient yield (Scheme 1). Encouraged by this novel synthetic route and the reactivity of these medium-sized sultams (B and C), which are synthetically important building blocks in drug discovery,7 we decided to investigate their chemical properties. Herein, we report the detailed syntheses of tetracyclic compounds containing a nine-membered sultam via sequential cobalt alkyne complex-mediated cyclization reactions.
RESULTS AND DISCUSSION
The preparation of the various cobalt precursors was based on a previously established, diverse synthetic route.6 First, enyne-sulfonamides 2a–c were prepared from sulfonamide 1 with one of three alcohols, but-3-en-1-ol, cis-pent-3-en-1-ol, or 2-methylbut-3-en-1-ol, in the presence of di-2-methoxyethyl azodicarboxylate (DMEAD) and PPh3. DEMAD, as reported by the Sugimura group,8 is a useful reagent for the Mitsunobu9 and Fukuyama o-nitrobenzenesulfonamide (nosylamide) protocol,10 allowing for a facile purification process. Compounds 2a–c were readily produced in 85%–93% yields by adopting this protocol (Scheme 2).
To test the reactivity of the propargyl group, the tert-butyldimethylsilyl (TBS) group was transformed into an acetyl (Ac) group, as the acetoxyl moiety is an excellent leaving group for the Nicholas reaction.2 The TBS groups were removed from 2a and 2b by adding tetrabutylammonium fluoride (TBAF) in tetrahydrofuran (THF) at 0 °C to give corresponding propargyl alcohols 3a and 3b. Compounds 3a and 3b were then converted into 4a and 4b in sufficient yields using anhydrous acetic acid with pyridine in CH2Cl2 at 0 °C (Scheme 3).
Chemoselective reduction of the nitro groups on 2a–c, 3a, and 4a,b was achieved using Fe in the presence of NH4Cl in aqueous ethanol, a process developed by the Ramadas group11a and Xiao group,11b to give aniline derivatives 5a–f in 88%–95% yields without any intermediates or side products. Subsequent dicobalt alkyne complexation of 5a–f was performed under simple conditions, mixing with a stoichiometric amount of dicobalt octacarbonyl [Co2(CO)8] in dichloromethane (DCM) or 1,2-dichloroethane (DCE) at ambient temperature for 2 h. Cobalt alkyne complexes 6a–f could all be isolated in excellent yields using silica gel chromatography; however, the PK cyclization gradually occurred in the warm water bath during evaporation (Scheme 4).
Once we obtained 6a–f, we began investigating the formation of nine-membered sultams with the cobalt alkyne complexes through an endo-type Nicholas cyclization reaction.2 The reaction conditions were found to be optimized when 2.4 equiv of boron trifluoride diethyl etherate in dilute DCE was used. Use of 1.2 equiv of BF3 required a longer reaction time, most likely due to the existence of the amino groups in 6a–f. Subsequently, 6a–f were converted into nine-membered sultams 7a–f using the optimized reaction conditions, i.e., BF3 (2.4 equiv) in DCE (0.005M) at 0 °C for 30 min. Interestingly, there was no difference in whether OTBS (6a), OH (6d), or OAc (6e) was used as the leaving group at the propargylic position, affording 7a, 7d, and 7e, respectively. Thus, we demonstrated that the Nicholas reaction could proceed even in the presence of the OTBS moiety as a protecting group. Therefore, there is no longer a need to exchange TBS for Ac, as in Scheme 3 (Scheme 5).
Next, we investigated the reactivity of the cobalt alkyne moiety with alkenes on 7a–c under intramolecular PK cyclization conditions.3 First, terminal alkene 7a was successfully converted to cyclopentenone 8a in 90% yield by simply heating at 60 °C for 30 min. In contrast, the PK cyclization of internal alkene 7b required reaction at 80 °C for 1 h to completely consume the cobalt complex and afford 8b as a single isomer in 61% yield. Thus, the methyl group on the alkene inhibited access between the alkene and cobalt moieties. Moreover, we previously reported that a methyl group at the allylic position affected the PK cyclization process, resulting in two diastereoisomers.12 Therefore, the attempted PK cyclization of 7c gave two isomers (8c and 8d) in about 10:1 ratio, demonstrating that repulsion between the methyl group and alkene moiety influenced the cyclization process (Scheme 6).
The stereochemistry and conformations of 8b and 8c were confirmed using single crystal X-ray analysis, as illustrated in Figure 1.13 The results indicated that the piperidine and aromatic rings were in close proximity due to the bent nine-membered ring conformation. The corresponding NMR spectroscopic data supported this observation. For example, the corresponding methyl peak in 8d (α-isomer) was observed at −0.17 ppm (d, J = 7.6 Hz, 3H) in the 1H NMR spectrum, as a result of the ring current effect.
To compare the diastereoselectivity of 8c:8d = ca. 10:1, we attempted the PK reaction of acyclic, alkyne cobalt complex 6c. Under the same reaction conditions, the major product, 9, was obtained in 67% yield with trace amounts of a minor product (over 50:1 ratio). The stereochemistry of 9 was confirmed by NMR spectroscopic measurements and was also supported by our previously reported compounds.12 These results indicate that diastereoselectivity generation is influenced by the nine-membered ring conformation throughout the PK reaction process (Scheme 7).
We also explored a one-pot procedure from 6a to 8a. After several attempts, we found that adding saturated aqueous NaHCO3 after the completion of the Nicholas reaction followed by heating afforded 8a in 87% yield without isolation and purification of 7a. When the NaHCO3 and heating were removed, the yield of 8a decreased to below 40%. Therefore, we demonstrated that the PK step from 7a proceeds smoothly under basic aqueous conditions, which avoid the decomposition of 7a (Scheme 8).
In conclusion, we investigated the combined use of the Nicholas and PK reactions to synthesize unique tetracyclic molecules 8a–d. In the Nicholas reaction, OTBS, OH, and OAc groups could function as leaving groups. Through the PK reaction, the diastereoselectivity of nine-membered ring fused cobalt complex 7c was different from that of acyclic 6c. We also successfully demonstrated a one-pot procedure for sequential cobalt reactions. We expect that these results will allow for the enhancement of the chemistry toward medium-sized heterocyclic sultams in drug discovery.
EXPERIMENTAL
General. Melting points were measured using a Yanaco micro-melting point apparatus. IR spectra were obtained using a PerkinElmer Spectrum 100 FT-IR spectrometer. 1H and 13C NMR spectra were recorded using JEOL JNM-ECA-600 spectrometer (1H: 600 MHz; 13C: 150 MHz) in CDCl3. The chemical shifts were reported in ppm (δ) using tetramethylsilane (TMS) as an internal standard. Coupling constants (J) were reported in hertz (Hz) using the following abbreviations for the multiplicity: s, singlet; d, doublet; t, triplet; m, multiplet; comp, complex, i.e., overlapping multiplets of magnetically nonequivalent protons; br, broad. Mass spectra were measured using a JEOL JMS-600 spectrometer.
N-[4-(tert-Butyldimethylsilyloxy)-but-2-ynyl]-2-nitrobenzenesulfonamide (1) Following the procedure used in our previous experiments,6 1 was prepared through a four-step sequence from 2-nitrobenznenesulfonamide and obtained as a colorless oil. The structure was confirmed by comparison to an authentic sample.6 1H NMR: δ 8.19–8.15 (m, 1H), 7.91–7.87 (m, 1H), 7.76–7.71 (comp, 2H), 5.62 (t, J = 6.0 Hz, 1H), 4.02 (dt, J = 6.0, 1.7 Hz, 2H), 3.96 (t, J = 1.7 Hz, 2H), 0.82 (s, 9H), 0.00 (s, 6H).
General procedure for synthesis of enyne-amides (2a–c)
To a stirred solution of 1 (1 equiv), alcohol (1.1–1.3 equiv), and PPh3 (1.2 equiv) in anhydrous toluene (5 mL per 1 mmol) was slowly added DMEAD (1.2 equiv) at 0 °C. After stirring for 24 h at ambient temperature, the reaction mixture was treated with H2O. The aqueous layer was separated from the organic layer and extracted with Et2O. The combined organic layers were dried with MgSO4 and concentrated under reduced pressure. The residue was purified by silica gel column chromatography by eluting with hexane:EtOAc (6:1 to 3:1) to give the corresponding enyne-amide (2a–c).
N-(But-3-en-1-yl)-N-[4-(tert-butyldimethylsilyloxy)-but-2-yn-1-yl]-2-nitrobenzenesulfonamide (2a):6 Obtained in 93% yield as a colorless oil. 1H NMR: δ 8.03 (dd, J = 7.6, 1.4 Hz, 1H), 7.70–7.64 (comp, 2H), 7.62 (dd, J = 7.6, 1.4 Hz, 1H), 5.70 (ddt, J = 17.2, 10.3, 6.9 Hz, 1H), 5.08 (dd, J = 17.2, 1.4 Hz, 1H), 5.02 (dd, J = 10.3, 1.4 Hz, 1H), 4.23 (s, 2H), 4.17 (s, 2H), 3.46 (t, J = 7.2 Hz, 2H), 2.34 (dt, J = 7.2, 6.9 Hz, 2H), 0.86 (s, 9H), 0.04 (s, 6H).
(Z)-N-[4-(tert-Butyldimethylsilyloxy)-but-2-yn-1-yl]-2-nitro-N-(pent-3-en-1-yl)benzenesulfonamide (2b): Obtained in 85% yield as a yellow oil. ¹H NMR: δ 8.02 (dd, J = 7.2, 1.8 Hz, 1H), 7.70–7.64 (comp, 2H), 7.62 (dd, J = 6.6, 1.8 Hz, 1H), 5.56–5.48 (m, 1H), 5.33–5.26 (m, 1H), 4.25 (s, 2H), 4.18 (s, 2H), 3.41 (t, J = 1.8 Hz, 2H), 2.34 (q, J = 7.2 Hz, 2H), 1.59 (d, J = 6.0 Hz, 3H), 0.86 (s, 9H), 0.05 (s, 6H). 13C NMR: δ 148.3, 133.6, 133.2, 131.7, 130.9, 127.2, 125.5, 124.3, 84.5, 77.8, 51.6, 46.3, 36.9, 25.8 (3C), 25.6, 18.3, 12.7, -5.2 (2C). IR (neat): 2929, 2857, 1544, 1471, 1439, 1359, 1254, 1164 cm-1. CIMS m/z: [M + H]+ calcd for C21H33N2O5SiS, 453.1879; found, 453.1906.
N-[4-(tert-Butyldimethylsilyloxy)-but-2-yn-1-yl]-N-(2-methylbut-3-en-1-yl)-2-nitrobenzenesulfon-
amide (2c): Obtained in 91% yield as a colorless oil. 1H NMR: δ 8.02 (dd, J = 7.6, 1.4 Hz, 1H), 7.69–7.64 (comp, 2H), 7.61 (dd, J = 6.9, 2.1 Hz, 1H), 5.65 (ddd, J = 17.5, 10.3, 7.6 Hz, 1H), 5.06 (d, J = 17.5 Hz, 1H), 4.98 (d, J = 10.3, 1H), 4.23 (t, J = 2.1 Hz, 2H), 4.14 (t, J = 2.1 Hz, 2H), 3.31 (d, J = 7.6 Hz, 2H), 2.51 (dtq, J = 7.6, 7.6, 6.6 Hz, 1H), 1.00 (d, J = 6.6 Hz, 3H), 0.86 (s, 9H), 0.04 (s, 6H). 13C NMR: δ 148.4, 140.5, 133.6, 133.1, 131.6, 131.0, 124.2, 115.5, 84.6, 77.4, 52.0, 51.5, 37.1, 36.3, 25.8 (3C), 18.3, 17.5, -5.1 (2C). IR (neat): 2957, 2930, 2858, 1545, 1463, 1359, 1254, 1164 cm-1. CIMS m/z: [M + H]+ calcd for C21H33N2O5SiS, 453.1879; found, 453.1885.
General procedure for synthesis of alcohols (3a, 3b)
To a stirred solution of silyl ether (2a or 2b) in THF (4 mL per 1 mmol) was slowly added TBAF (1 M in THF, 1.2 equiv) at 0 °C. The mixture was stirred for 0.5–1 h at room temperature and treated with H2O. The aqueous layer was extracted with EtOAc and the combined organic layers were washed with brine, dried with MgSO4, and concentrated under reduced pressure. The residue was purified by silica gel column chromatography by eluting with hexane:EtOAc (1:2) to give the corresponding alcohol (3a or 3b).
N-(But-3-en-1-yl)-N-(4-hydroxybut-2-yn-1-yl)-2-nitrobenzenesulfonamide (3a):6 Obtained in 85% yield as a colorless oil. 1H NMR: δ 8.06 (dd, J = 7.6, 2.1 Hz, 1H), 7.72–7.66 (comp, 2H), 7.64 (dd, J = 7.6, 2.1 Hz, 1H), 5.70 (ddt, J = 17.2, 10.3, 6.9 Hz, 1H), 5.09 (ddt, J = 17.2, 3.4, 1.4 Hz, 1H), 5.03 (dd, J = 10.3, 1.4 Hz, 1H), 4.22 (s, 2H), 4.13 (d, J = 5.5 Hz, 2H), 3.46 (t, J = 7.6 Hz, 2H), 2.34 (dt, J = 7.6, 6.9 Hz, 2H), 1.55–1.43 (br s, 1H).
(Z)-N-(4-Hydroxybut-2-yn-1-yl)-2-nitro-N-(pent-3-en-1-yl)benzenesulfonamide (3b): Obtained in 97% yield as a colorless oil. ¹H NMR: δ 8.05–7.97 (m, 1H), 7.71–7.65 (m, 2H), 7.64–7.59 (m, 1H), 5.52–5.44 (m, 1H), 5.30–5.21 (m, 1H), 4.19 (s, 2H), 4.13–4.07 (m, 2H), 3.36 (t, J = 7.2 Hz, 2H), 2.30 (dt, J = 6.6, 6.6 Hz, 2H), 1.54 (dd, J = 7.2, 2.4 Hz, 3H). 13C NMR: δ 148.2, 133.9, 132.8, 131.9, 130.9, 127.2, 125.5, 124.3, 84.1, 78.7, 50.7, 46.5, 36.9, 25.6, 12.9. IR (neat): 3414, 2920, 1729, 1535, 1439, 1351, 1161 cm-1. CIMS m/z: [M + H]+ calcd for C15H19N2O5S, 339.1014; found, 339.0997.
General procedure for synthesis of acetates (4a, b)
To a stirred solution of alcohol (3a or 3b) in the presence of pyridine (1.5–2.0 equiv) in anhydrous CH2Cl2 (4 mL per 1 mmol) was slowly added Ac2O (1.2–1.5 equiv) at 0 °C. The mixture was stirred for 24 h at room temperature and treated with saturated aqueous NaHCO3. The aqueous layer was separated from the CH2Cl2 layer and extracted with additional CH2Cl2. The combined organic layers were washed with 1 M aqueous HCl, washed with brine, dried with MgSO4, and concentrated under reduced pressure. The residue was purified by silica gel column chromatography by eluting with hexane:EtOAc (2:1) to give the corresponding acetate (4a or 4b).
4-[N-(But-3-en-1-yl)-2-nitrophenylsulfonamide]-but-2-yn-1-yl acetate (4a):6 Obtained in 93% yield as a colorless oil. 1H NMR: δ 8.04–7.99 (m, 1H), 7.72–7.66 (comp, 2H), 7.65–7.60 (m, 1H), 5.69 (ddt, J = 17.2, 10.3, 6.9 Hz, 1H), 5.08 (dd, J = 17.2, 1.4 Hz, 1H), 5.02 (dd, J = 10.3, 1.4 Hz, 1H), 4.51 (s, 2H), 4.23 (s, 2H), 3.44 (t, J = 6.9 Hz, 2H), 2.33 (dt, J = 7.6, 6.9 Hz, 2H), 2.04 (s, 3H).
(Z)-4-[2-Nitro-N-(pent-3-en-1-yl)phenylsulfonamide]-but-2-yn-1-yl acetate (4b): Obtained in 82% yield as a colorless oil. ¹H NMR: δ 8.04–8.00 (m, 1H), 7.71–7.66 (comp, 2H), 7.65–7.61 (m, 1H), 5.55–5.48 (m, 1H), 5.32–5.25 (m, 1H), 4.52 (t, J = 1.8 Hz, 2H), 4.24 (t, J = 1.8 Hz, 2H), 3.38 (t, J = 6.9 Hz, 2H), 2.33 (dt, J = 7.6, 6.9 Hz, 2H), 2.04 (s, 3H), 1.58 (d, J = 6.9 Hz, 3H). 13C NMR: δ 170.1, 148.3, 133.7, 133.0, 131.8, 130.9, 127.2, 125.4, 124.3, 80.0, 79.8, 51.9, 46.5, 36.9, 25.6, 20.8, 12.9. IR (neat): 2937, 1741, 1550, 1438, 1356, 1222, 1163 cm-1. CIMS m/z: [M + H]+ calcd for C17H21N2O6S, 381.1120; found, 381.1106.
General procedure for synthesis of aniline derivatives (5a–f)
To a stirred suspension of a 2-nitrobenzene derivative (2a–c, 3a, 4a,b) in the presence of NH4Cl (10 equiv) in EtOH:H2O (1:1, 20 mL per 1 mmol) was added iron powder (10 equiv) at room temperature. The reaction mixture was stirred vigorously for 24 h and filtered through a Celite pad with EtOAc. After removal of EtOH and EtOAc from the filtrate under reduced pressure, the water layer was extracted with EtOAc. The organic layer was dried with MgSO4 and concentrated under reduced pressure to give the corresponding aniline derivative (5a–f).
2-Amino-N-but-3-enyl-N-[4-(tert-butyldimethylsilyloxy)-but-2-ynyl]benzenesulfonamide (5a): Obtained in 96% yield as a colorless oil. 1H NMR: δ 7.60 (dd, J = 7.6, 1.4 Hz, 1H), 7.27–7.23 (m, 1H), 6.71 (dd, J = 8.3, 6.9 Hz, 1H), 6.67 (d, J = 8.3 Hz, 1H), 5.72 (ddt, J = 17.2, 10.3, 6.9 Hz, 1H), 5.07 (d, J = 17.2 Hz, 1H), 5.04–5.00 (comp, 3H), 4.13 (s, 2H), 4.13 (s, 2H), 3.33 (t, J = 7.6 Hz, 2H), 2.30 (dt, J = 7.6, 6.9 Hz, 2H), 0.86 (s, 9H), 0.05 (s, 6H). 13C NMR: δ 146.0, 134.6, 134.0, 130.2, 120.4, 117.6, 117.2, 117.1, 83.9, 77.6, 51.5, 45.8, 36.7, 32.1, 25.8 (3C), 18.2, -5.2 (2C). IR (neat): 3483, 3380, 2929, 2857, 1617, 1483, 1453, 1340, 1320, 1139 cm-1. CIMS m/z: [M + H]+ calcd for C20H33N2O3SiS, 409.1981; found, 409.1954.
(Z)-2-Amino-N-[4-(tert-butyldimethylsilyloxy)-but-2-yn-1-yl]-N-(pent-3-en-1-yl)benzenesulfonamide (5b): Obtained in 92% yield as a yellow oil. ¹H NMR: δ 7.60 (dd, J = 8.3, 1.4 Hz, 1H), 7.24 (ddd, J = 8.3, 6.9, 1.4 Hz, 1H), 6.70 (ddd, J = 8.3, 6.9, 1.4 Hz, 1H), 6.67 (dd, J = 8.3, 1.4 Hz, 1H), 5.56–5.49 (m, 1H), 5.34–5.28 (m, 1H), 5.02 (s, 2H), 4.14 (t, J = 1.7 Hz, 2H), 4.12 (t, J = 1.7 Hz, 2H), 3.28 (t, J = 7.6 Hz, 2H), 2.30 (dt, J = 7.6, 7.2 Hz, 2H), 1.59 (d, J = 6.9 Hz, 3H), 0.86 (s, 9H), 0.05 (s, 6H). 13C NMR: δ 146.2, 134.1, 130.2, 126.7, 126.0, 120.5, 117.6, 117.3, 83.9, 77.8, 51.6, 46.0, 36.8, 25.9 (3C), 25.6, 18.3, 13.0, -5.1 (2C). IR: 3479, 3379, 2929, 2857, 1617, 1566, 1483, 1453, 1339, 1320, 1255, 1143, 1079 cm-1. CIMS m/z: [M + H]+ calcd for C21H35N2O3SiS, 423.2137; found, 423.2145.
2-Amino-N-[4-(tert-butyldimethylsilyloxy)-but-2-yn-1-yl]-N-(2-methylbut-3-en-1-yl)- benzenesulfonamide (5c): Obtained in 96% yield as a yellow oil. 1H NMR: δ 7.60 (dd, J = 8.3, 1.4 Hz, 1H), 7.25 (ddd, J = 8.3, 7.3, 1.4 Hz, 1H), 6.71 (dd, J = 8.3, 7.3 Hz, 1H), 6.66 (d, J = 8.3 Hz, 1H), 5.68 (ddd, J = 18.4, 10.3, 7.6 Hz, 1H), 5.04 (d, J =18.4 Hz, 1H), 5.03 (s, 2H), 4.99 (d, J = 10.3 Hz, 1H), 4.13–4.08 (comp, 4H), 3.19–3.12 (m, 2H), 2.47 (dtq, J = 7.6, 7.6, 6.9 Hz, 1H), 0.99 (d, J = 6.9 Hz, 3H), 0.86 (s, 9H), 0.05 (s, 6H). 13C NMR: δ 146.2, 141.0, 134.1, 130.3, 120.5, 117.6, 117.2, 115.0, 84.0, 77.6, 51.8, 51.6, 37.2, 36.2, 25.9 (3C), 18.3, 17.5, -5.1 (2C). IR (neat): 3479, 3380, 2957, 2929, 2858, 1617, 1484, 1454, 1341, 1321, 1254, 1137, 1077 cm-1. CIMS m/z: [M + H]+ calcd for C21H35N2O3SiS, 423.2137; found 423.2165.
2-Amino-N-(but-3-en-1-yl)-N-(4-hydroxybut-2-yn-1-yl)benzenesulfonamide (5d): Obtained in 88% yield as a colorless oil. 1H NMR: δ 7.61 (d, J = 8.3, 1H), 7.30–7.24 (m, 1H), 6.72 (d, J = 8.3, 6.9 Hz, 1H), 6.69 (d, J = 8.3 Hz, 1H), 5.72 (ddt, J = 17.2, 10.3, 6.9 Hz, 1H), 5.20–4.92 (comp, 4H), 4.11 (s, 2H), 4.04 (s, 2H), 3.34 (t, J = 7.3 Hz, 2H), 2.29 (dt, J = 7.3, 6.9 Hz, 2H), 1.93–1.57 (br s, 1H). 13C NMR: δ 146.3, 134.6, 134.2, 130.5, 120.4, 117.7, 117.3, 117.2, 83.6, 78.8, 50.9, 46.1, 36.7, 32.2. IR (neat): 3482, 3379, 2927, 1617, 1484, 1453, 1319, 1139 cm-1. CIMS m/z: [M + H]+ calcd for C14H19N2O3S, 295.1116; found, 295.1123.
4-[(2-Aminobenzenesulfonyl)-but-3-enylamino]but-2-ynyl acetate (5e):6 Obtained in 93% yield as a colorless oil. 1H NMR: δ 7.59 (dd, J = 8.4, 1.4 Hz, 1H), 7.27–7.22 (m, 1H), 6.73–6.65 (comp, 2H), 5.71 (ddt, J = 17.2, 10.7, 6.9 Hz, 1H), 5.07 (dd, J = 17.2, 1.4 Hz, 1H), 5.05 (br s, 2H), 5.01 (d, J = 10.7 Hz, 1H), 4.45 (t, J = 2.1 Hz, 2H), 4.12 (t, J = 2.1 Hz, 2H), 3.31 (t, J = 6.9 Hz, 2H), 2.29 (dt, J = 6.9, 6.9 Hz, 2H), 2.04 (s, 3H).
(Z)-4-[2-Amino-N-(pent-3-en-1-yl)phenylsulfonamide]-but-2-yn-1-yl acetate (5f): Obtained in 95% yield as a light yellow oil. ¹H NMR: δ 7.61 (dd, J = 8.0, 1.4 Hz, 1H), 7.26 (ddd, J = 8.5, 7.6, 1.4 Hz, 1H), 6.72 (dd, J = 8.0, 7.6 Hz, 1H), 6.68 (d, J = 8.5 Hz, 1H), 5.56–5.49 (m, 1H), 5.35–5.28 (m, 1H), 5.03 (br s, 2H), 4.47 (t, J = 2.1 Hz, 2H), 4.15 (t, J = 2.1 Hz, 2H), 3.26 (t, J = 7.6 Hz, 2H), 2.29 (dt, J = 7.6, 7.6 Hz, 2H), 2.05 (s, 3H), 1.59 (d, J = 6.9 Hz, 3H). 13C NMR: δ 170.2, 146.2, 134.2, 130.3, 126.5, 125.9, 120.4, 117.7, 117.3, 80.0, 79.2, 52.1, 46.2, 36.8, 25.6, 20.8, 12.9. IR (neat): 3481, 3380, 3017, 2936, 1736, 1616, 1483, 1453, 1320, 1220, 1145 cm-1. CIMS m/z: [M + H]+ calcd for C17H23N2O4S, 351.1378; found, 351.1353.
General procedure for synthesis of cobalt complexes (6a–f)
To a stirred solution of an alkyne derivative (5a–f) in anhydrous CH2Cl2 or ClCH2CH2Cl (10 mL per 1 mmol) was added dicobalt octacarbonyl (1.05 equiv) at room temperature under an Ar atmosphere. After stirring for 2 h, the solvent was removed under reduced pressure. The residue was purified by silica gel column chromatography by eluting with CHCl3 or hexane:EtOAc (20:1 to 6:1) to give the corresponding cobalt complex (6a–f).
Cobalt complex with 5a (6a): Obtained in 98% yield as a dark red oil. 1H NMR: δ 7.67 (d, J = 8.3 Hz, 1H), 7.30 (dd, J = 8.3, 7.3 Hz, 1H), 6.76 (dd, J = 7.6, 7.3 Hz, 1H), 6.71 (d, J = 7.6 Hz, 1H), 5.59 (ddt, J = 16.5, 9.6, 6.9 Hz, 1H), 5.03–4.93 (comp, 4H), 4.82 (s, 2H), 4.65 (s, 2H), 3.44 (t, J = 7.6 Hz, 2H), 2.20 (dt, J = 7.6, 6.9 Hz, 2H), 0.93 (s, 9H), 0.12 (s, 6H). 13C NMR: δ 199.4 (6C), 145.7, 134.3, 134.1, 130.0, 121.7, 117.8, 117.6, 117.3, 98.3, 89.7, 63.7, 49.5, 46.4, 31.6, 25.9 (3C), 18.4, -5.6 (2C). IR (neat): 3482, 3379, 2929, 2857, 2093, 2051, 2018, 1617, 1483, 1454, 1337, 1143 cm-1. The corresponding molecular ion was not detected using either EIMS or CIMS methods.
Cobalt complex with 5b (6b): Obtained in 99% yield as a dark red oil. 1H NMR: δ 7.73–7.63 (m, 1H), 7.33–7.20 (m, 1H), 6.80–6.68 (comp, 2H), 5.51–5.42 (m, 1H), 5.22–5.13 (m, 1H), 5.00 (br s, 2H), 4.82 (br s, 2H), 4.67 (br s, 2H), 3.42–3.32 (m, 2H), 2.24–2.14 (m, 2H), 1.57–1.45 (m, 3H), 0.92 (br s, 9H), 0.11 (br s, 6H). 13C NMR: δ 199.5 (6C), 145.8, 134.2, 130.0, 126.9, 125.7, 121.9, 117.8, 117.7, 98.5, 89.9, 63.8, 49.5, 46.6, 25.9 (3C), 25.0, 18.5, 12.8, -5.5 (2C). IR: 3485, 3380, 2930, 2859, 2093, 2050, 2005, 1617, 1569, 1483, 1454, 1336, 1320, 1258, 1142 cm-1. The corresponding molecular ion was not detected using either EIMS or CIMS methods.
Cobalt complex with 5c (6c): Obtained in 93% yield as a dark red oil. ¹H NMR: δ 7.67 (d, J = 7.3 Hz, 1H), 7.33–7.23 (m, 1H), 6.75 (dd, J = 7.3, 6.2 Hz, 1H), 6.69 (d, J = 7.6 Hz, 1H), 5.46 (ddd, J = 17.2, 9.6, 8.3 Hz, 1H), 4.97 (br s, 2H), 4.91–4.67 (comp, 6H), 3.35–3.17 (m, 2H), 2.39–2.28 (m, 1H), 0.93 (s, 9H), 0.82 (d, J = 6.2 Hz, 3H), 0.11 (s, 6H). 13C NMR: δ 199.5 (6C), 145.8, 140.7, 134.2, 130.2, 122.0, 117.7, 117.6, 115.3, 98.7, 89.1, 63.8, 51.8, 50.0, 36.4, 26.0 (3C), 18.5, 17.5, -5.5 (2C). IR (neat): 3486, 3382, 2955, 2931, 2858, 2092, 2048, 2009, 1857, 1618, 1485, 1455, 1335, 1320, 1257, 1140, 1066 cm-1. The corresponding molecular ion was not detected using either EIMS or CIMS methods.
Cobalt complex with 5d (6d): Obtained in 88% yield as a dark red oil. 1H NMR: δ 7.66 (d, J = 7.6 Hz, 1H), 7.37–7.28 (m, 1H), 6.82–6.74 (m, 1H), 6.73 (d, J = 7.6 Hz, 1H), 5.61–5.47 (m, 1H), 5.10–4.80 (comp, 6H), 4.69 (s, 2H), 3.45–3.30 (comp, 3H), 2.18–2.08 (m, 2H). 13C NMR: δ 199.3 (6C), 145.9, 134.7, 134.0, 130.1, 120.8, 118.0, 117.9, 117.7, 97.2, 90.4, 63.7, 50.1, 47.1, 31.8. IR (neat): 3481, 3384, 2934, 2093, 2050, 2002, 1617, 1483, 1454, 1319, 1139 cm-1. The corresponding molecular ion was not detected using either EIMS or CIMS methods.
Cobalt complex with 5e (6e):6 Obtained in 96% yield as a dark red oil. 1H NMR: δ 7.65 (d, J = 7.9 Hz, 1H), 7.30 (dd, J = 8.3, 7.6 Hz, 1H), 6.77 (dd, J = 7.9, 7.6 Hz, 1H), 6.72 (d, J = 8.3 Hz, 1H), 5.62–5.53 (m, 1H), 5.34 (s, 2H), 5.03–4.93 (comp, 4H), 4.64 (s, 2H), 3.40 (t, J = 7.6 Hz, 2H), 2.19 (dt, J = 8.3, 7.6 Hz, 2H), 2.12 (s, 3H).
Cobalt complex with 5f (6f): Obtained in 99% yield as a dark red oil. ¹H NMR: δ 7.67 (d, J = 8.3 Hz, 1H), 7.29 (dd, J = 8.3, 7.6 Hz, 1H), 6.77 (dd, J = 8.3, 7.6 Hz, 1H), 6.71 (d, J = 8.3 Hz, 1H), 5.51–5.44 (m, 1H), 5.35 (s, 2H), 5.20–5.13 (m, 1H), 5.00 (br s, 2H), 4.67 (s, 2H), 3.33 (t, J = 8.0 Hz, 2H), 2.18 (dt, J = 8.0, 7.6 Hz, 2H), 2.12 (s, 3H), 1.50 (d, J = 6.2 Hz, 3H). 13C NMR: δ 199.0 (6C), 170.7, 145.9, 134.3, 130.0, 127.1, 125.4, 121.6, 117.9, 117.8, 91.4, 90.7, 64.9, 49.5, 47.0, 25.1, 20.6, 12.8. IR (neat): 3480, 3383, 2096, 2056, 2023, 1740, 1617, 1483, 1454, 1374, 1336, 1264, 1220, 1143 cm-1. The corresponding molecular ion was not detected using either EIMS or CIMS methods.
General procedure for synthesis of nine-membered ring products (7a–c)
To a stirred solution of a cobalt alkyne complex (6a–f) in anhydrous CH2Cl2 or ClCH2CH2Cl (200 mL per 1 mmol) was slowly added BF3·Et2O (2.4 equiv) at 0 °C. The mixture was stirred for 0.5–2 h to consume the starting materials, as monitored by TLC, and was quenched with saturated aqueous NaHCO3 at 0 °C. The organic layer was separated from the aqueous layer, dried with MgSO4, and concentrated under reduced pressure. The residue was purified by silica gel column chromatography by eluting with hexane:EtOAc (10:1) or CH2Cl2 to give the corresponding nine-membered ring derivative (7a–c).
Nine-membered sultam with cobalt complex (7a):6 Obtained in 82% yield from 6a, 69% yield from 6d, and 72% yield from 6e as a dark red oil. 1H NMR: δ 7.77 (d, J = 7.9 Hz, 1H), 7.43 (dd, J = 7.7, 7.6 Hz, 1H), 7.19 (d, J = 7.7 Hz, 1H), 6.90 (dd, J = 7.9, 7.6 Hz, 1H), 6.16 (dd, J = 6.9, 6.9 Hz, 1H), 5.80 (ddt, J = 17.2, 10.3, 6.9 Hz, 1H), 5.17 (d, J = 17.2 Hz, 1H), 5.11 (d, J = 10.3 Hz, 1H), 4.90–4.60 (comp, 3H), 3.09–3.01 (br s, 2H), 2.53 (dt, J = 7.6, 6.9 Hz, 2H).
Nine-membered sultam with cobalt complex (7b): Obtained in 84% yield from 6b and 77% yield from 6f as a dark red oil. ¹H NMR: δ 7.78 (d, J = 7.6 Hz, 1H), 7.42 (dd, J = 8.0, 7.6 Hz, 1H), 7.18 (d, J = 8.0 Hz, 1H), 6.90 (dd, J = 7.6, 7.6 Hz, 1H), 6.17 (t, J = 7.1 Hz, 1H), 5.68–5.56 (m, 1H), 5.44–5.34 (m, 1H), 5.00–4.60 (comp, 4H), 3.09–2.92 (m, 2H), 2.58–2.50 (m, 2H), 1.69 (d, J = 6.2 Hz, 3H). 13C NMR: δ 198.6 (6C), 146.5, 134.4, 130.1, 129.5, 127.6, 125.7, 122.5, 120.9, 96.0, 89.5, 53.9, 48.8, 47.2, 27.3, 12.9. IR (neat): 3370, 2928, 2092, 2051, 2005, 1594, 1578, 1503, 1462, 1336, 1148 cm-1. The corresponding molecular ion was not detected using either EIMS or CIMS methods.
Nine-membered sultam with cobalt complex (7c): Obtained in 72% yield from 6c as a dark red oil. 1H NMR: δ 7.76 (d, J = 8.0 Hz, 1H), 7.43 (dd, J = 8.0, 7.3 Hz, 1H), 7.19 (d, J = 8.0 Hz, 1H), 6.91 (dd, J = 8.0, 7.3 Hz, 1H), 6.17 (s, 1H), 5.74 (ddd, J = 17.6, 9.6, 8.3 Hz, 1H), 5.17 (d, J = 17.6 Hz, 1H), 5.09 (d, J = 9.6 Hz, 1H), 5.00–4.54 (comp, 4H), 2.96–2.83 (m, 2H), 2.82–2.71 (m, 1H), 1.07 (d, J = 6.9 Hz, 3H). 13C NMR: δ 198.6 (6C), 146.6, 140.8, 134.5, 130.4, 129.4, 122.7, 121.0, 115.8, 95.8, 88.9, 54.1, 52.5, 49.2, 37.9, 17.7. IR (neat): 3374, 2972, 2930, 2092, 2052, 2017, 1594, 1463, 1336, 1149 cm-1. The corresponding molecular ion was not detected using either EIMS or CIMS methods.
General procedure for synthesis of tetracycles (8a–d)
A solution of sultam (7a–c) in anhydrous DCE (200 mL per 1 mmol) was heated to either 60 °C or 80 °C and stirred for 0.5–1 h. The reaction mixture was cooled to room temperature and filtered through a filter paper to remove the insoluble black materials. The filtrate was concentrated under reduced pressure. The residue was purified by silica gel column chromatography by eluting with hexane:EtOAc (1:1 to 0:1) to give the corresponding tetracycle (8a–d).
(E)-2,3,11,12-Tetrahydro-3,5-ethanobenzo[h]cyclopenta[d][1,2,7]thiadiazonin-1-(4H)-one 6,6-dioxide (8a):6 Obtained in 90% yield as a white solid. mp 255 °C dec. 1H NMR: δ 7.71 (dd, J = 7.8, 1.4 Hz, 1H), 7.35 (ddd, J = 7.9, 7.0, 1.4 Hz, 1H), 7.10 (d, J = 7.9 Hz, 1H), 6.91 (dd, J = 7.8, 7.0 Hz, 1H), 5.57 (dd, J = 11.0, 6.2 Hz, 1H), 5.05 (dd, J = 15.1, 2.1 Hz, 1H), 4.44 (dd, J = 16.1, 11.0 Hz, 1H), 3.95–3.90 (m, 1H), 3.83 (dd, J = 16.1, 6.2 Hz, 1H), 3.79 (d, J =15.1 Hz, 1H), 3.29 (ddd, J = 15.5, 13.1, 2.8 Hz, 1H), 2.71–2.66 (m, 1H), 2.44 (dd, J = 19.4, 6.2 Hz, 1H), 1.83 (dddd, J = 13.1, 4.8, 2.8, 2.1 Hz, 1H), 1.54 (d, J = 19.4 Hz, 1H), 0.13 (dddd, J = 15.3, 13.1, 13.1, 4.5 Hz, 1H).
(2R/S,3S/R,E)-2-Methyl-2,3,11,12-tetrahydro-3,5-ethanobenzo[h]cyclopenta[d][1,2,7]thiadiazonin-1-(4H)-one 6,6-dioxide (8b): Obtained in 61% yield as a white solid. mp 260 °C dec. ¹H NMR: δ 7.70 (dd, J = 7.9, 1.7 Hz, 1H), 7.33 (ddd, J = 8.1, 7.9, 1.7 Hz, 1H), 7.11 (dd, J = 8.3, 1.4 Hz, 1H), 6.90 (dd, J = 7.6, 6.9 Hz, 1H), 5.59 (br s, 1H), 5.05 (dd, J = 15.2, 2.1 Hz, 1H), 4.45 (dd, J = 15.8, 11.0 Hz, 1H), 3.95 (ddd, J = 15.8, 2.8, 2.8 Hz, 1H), 3.84 (dd, J = 15.8, 6.2 Hz, 1H), 3.79 (d, J = 15.2 Hz, 1H), 3.28 (ddd, J = 15.8, 13.1, 2.8 Hz, 1H), 2.75 (ddd, J = 11.4, 6.5, 5.5 Hz, 1H), 2.40 (ddd, J = 15.1, 7.6, 7.6 Hz, 1H), 1.64–1.56 (m, 1H), 0.67 (d, J = 7.6 Hz, 3H), 0.12–0.03 (m, 1H). 13C NMR: δ 209.1, 168.7, 147.0, 138.1, 134.2, 131.5, 128.8, 124.0, 121.7, 46.9, 46.3, 43.3, 42.5, 39.4, 32.2, 8.9. IR (neat): 3392, 2928, 1700, 1463, 1333, 1149 cm-1. CIMS m/z: [M + H]+ calcd for C16H19N2O3S, 319.1116; found, 319.1118.
(3R/S,14R/S,E)-14-Methyl-2,3,11,12-tetrahydro-3,5-ethanobenzo[h]cyclopenta[d][1,2,7]thiadiazonin-1-(4H)-one 6,6-dioxide (8c): Obtained in 67% yield as a white solid. mp 212–213 °C. 1H NMR: δ 7.68 (dd, J = 8.0, 1.6 Hz, 1H), 7.34 (ddd, J = 8.6, 6.9, 1.6 Hz, 1H), 7.08 (d, J = 8.6 Hz, 1H), 6.90 (dd, J = 8.0, 6.9 Hz, 1H), 5.56–5.48 (m, 1H), 5.02 (dd, J = 15.2, 2.1 Hz, 1H), 4.42 (dd, J = 16.5, 11.0 Hz, 1H), 3.86–3.79 (comp, 2H), 3.74 (d, J = 15.1 Hz, 1H), 2.93 (dd, J = 15.1, 12.4 Hz, 1H), 2.40 (dd, J = 19.3, 6.2 Hz, 1H), 2.24 (dd, J = 10.3, 6.2 Hz, 1H), 1.61 (d, J = 18.6 Hz, 1H), 0.78 (d, J = 6.9 Hz, 3H), 0.28–0.18 (m, 1H). 13C NMR: δ 206.5, 168.6, 147.0, 138.7, 134.4, 131.4, 128.7, 123.9, 121.8, 52.7, 46.4, 45.8, 42.4, 39.6, 39.1, 17.5. IR (neat): 3399, 2968, 2950, 2888, 1698, 1650, 1595, 1460, 1328, 1130 cm-1. CIMS m/z: [M + H]+ calcd for C16H19N2O3S, 319.1116; found, 319.1138.
(3R/S,14S/R,E)-14-Methyl-2,3,11,12-tetrahydro-3,5-ethanobenzo[h]cyclopenta[d][1,2,7]thiadiazonin-1-(4H)-one 6,6-dioxide (8d): Obtained in 7% yield from 7c as a white solid. mp 215–216 °C. 1H NMR: δ 7.73 (dd, J = 8.5, 1.4 Hz, 1H), 7.35 (ddd, J = 8.0, 7.3, 1.4 Hz, 1H), 7.19 (d, J = 8.0 Hz, 1H), 6.89 (dd, J = 8.5, 7.3 Hz, 1H), 5.26–5.19 (m, 1H), 5.13 (d, J =14.4 Hz, 1H), 4.50 (dd, J =16.5, 11.0 Hz, 1H), 3.90 (d, J = 17.2 Hz, 1H), 3.76 (d, J = 14.4 Hz, 1H), 3.52–3.44 (comp, 2H), 3.04 (dd, J = 6.9, 6.9 Hz, 1H), 2.31–2.22 (comp, 2H), 1.98 (d, J =18.6 Hz, 1H), -0.17 (d, J = 7.6 Hz, 3H). 13C NMR: δ 206.9, 167.1, 146.6, 138.5, 134.3, 131.8, 130.4, 122.9, 121.2, 52.3, 46.9, 41.8, 40.2, 37.6, 36.1, 12.4. IR (neat): 3399, 2968, 2888, 1699, 1651, 1460, 1328, 1143 cm-1. CIMS m/z: [M + H]+ calcd for C16H19N2O3S, 319.1116; found, 319.1122.
(4R/S,4aR/S)-2-(2-Aminophenylsulfonyl)-7-[(tert-butyldimethylsilyloxy)methyl]-4-methyl-1,2,3,4,4a,5-hexahydro-6H-cyclopenta[c]pyridin-6-one (9): Cobalt complex 6c (134 mg, 0.189 mmol) was dissolved in ClCH2CH2Cl (38 mL) and stirred at 80 °C for 30 min. The reaction mixture was filtered through a Celite pad with CHCl3 and the filtrate was evaporated under reduced pressure. The residue was purified by silica gel column chromatography by eluting with hexane:EtOAc (2:1 to 1:1) to give cyclopentenone 9 (57 mg) in 67% yield as a white solid. mp 150–151 °C. 1H NMR: δ 7.58 (d, J = 8.0 Hz, 1H), 7.29 (dd, J = 8.3, 7.1 Hz, 1H), 6.74 (dd, J = 8.0, 7.1 Hz, 1H), 6.71 (d, J = 8.3 Hz, 1H), 5.29 (d, J = 14.4 Hz, 1H), 5.03 (s, 2H), 4.43 (d, J = 14.4 Hz, 1H), 4.36 (d, J = 14.4 Hz, 1H), 3.86–3.81 (m, 1H), 3.33 (d, J = 14.4 Hz, 1H), 2.54 (dd, J = 18.6, 6.2 Hz, 1H), 2.40 (dd, J = 11.0, 11.0 Hz, 1H), 2.20–2.13 (m, 1H), 1.97 (dd, J = 18.6, 2.8 Hz, 1H), 1.58–1.48 (m, 1H), 0.96 (d, J = 6.2 Hz, 3H), 0.91 (s, 9H), 0.12 (s, 3H), 0.07 (s, 3H). 13C NMR: δ 206.0, 166.6, 146.3, 138.2, 134.5, 130.4, 118.3, 117.8, 117.4, 56.9, 51.9, 45.41, 45.40, 39.5, 37.8, 26.0 (3C), 18.4, 17.7, -5.4, -5.5. IR (neat): 3483, 3376, 2928, 2859, 1697, 1663, 1633, 1484, 1454, 1289, 1131 cm-1. CIMS m/z: [M + H]+ calcd for C22H35N2O4SiS, 451.2087; found, 451.2074.
One-pot procedure for the synthesis of 8a from 6a
To a stirred solution of 6a (100 mg, 0.14 mmol) in DCE (29 mL) was added BF3·Et2O (44 μL, 0.35 mmol) at 0 °C. After stirring for 30 min, saturated aqueous NaHCO3 was added to the mixture and stirred for 1 h at room temperature. The reaction mixture was then heated to 60 °C for 3 h. The organic layer and aqueous layer were separated and the organic layer was dried with MgSO4 and concentrated under reduced pressure. The residue was purified by silica gel column chromatography by eluting with CHCl3 to afford 8a (38 mg) in 87% yield.
ACKNOWLEDGEMENTS
The financial support from Hokkaido Pharmaceutical University is greatly helpful to our research. We thank Prof. Dr. Tsubuki (Hoshi University, Japan) and Dr. Kasai (Hoshi University, Japan) for their assistance with the mass spectrometric measurements and Dr. Hiroto (Nagoya University, Japan) for helping with the X-ray crystarographic measurements. We thank Enago (www.enago.jp) for the English language review. This work is partly supported by Nanotechnology Platform Program (Molecule and Material Synthesis) of the Ministry of Education, Culture, Sports, Science and Technology (MEXT), Japan.
References
1. (a) I. Omae, Appl. Organometal. Chem., 2007, 21, 318; CrossRef (b) J. Pérez-Castells, 'Cascade Reactions Involving Pauson-Khand and Related Processes', In Metal Catalyzed Cascade Reactions, Springer Berlin Heidelberg. 2006, pp. 207–257. CrossRef
2. (a) N. Kann, Curr. Org. Chem., 2012, 16, 322; CrossRef (b) B. J. Teobald, Tetrahedron, 2002, 58, 4133; CrossRef (c) J. R. Green, Curr. Org. Chem., 2001, 5, 809. CrossRef
3. (a) 'The Pauson-Khand Reaction Scope, Variation and Applications', ed. by R. R. Torres, John Wiley & Sons Ltd: UK, 2012, pp. 1–305; (b) H. W. Lee and F. Y. Kwong, Eur. J. Org. Chem., 2010, 5, 789; CrossRef (c) B. E. Moulton, 'The Pauson-Khand Reaction', In Organometallic Chemistry, ed. by I. Fairlamb and J. Lynam, RCS, London, 2010; Vol. 36, pp. 93–120. CrossRef
4. (a) R. Ni, N. Mitsuda, T. Kashiwagi, K. Igawa, and K. Tomooka, Angew. Chem. Int. Ed., 2015, 54, 1190; CrossRef (b) J. Huang, L. Fang, J. Gong, C. Li, and Z. Yang, Tetrahedron, 2015, 71, 3720; CrossRef (c) J. R. López, N. Ortega, V. S. Martin, and T. Martin, Chem. Commun., 2014, 50, 3685; CrossRef (d) S. J. McKerrall, L. Jørgensen, C. A. Kuttruff, F. Ungeheuer, and P. S. Baran, J. Am. Chem. Soc., 2014, 136, 5799. CrossRef
5. (a) K. D. Closser, M. M. Quintal, and K. M. Shea, J. Org. Chem., 2009, 74, 3680; CrossRef (b) D. G. J. Young, J. A. Burlison, and U. Peters, J. Org. Chem., 2003, 68. 3494; CrossRef (c) T. F. Jamison, S. Shambayati, W. E. Crowe, and S. L. Schreiber, J. Am. Chem. Soc., 1997, 119, 4353; CrossRef (d) N. Jeong, S. Yoo, S. J. Lee, S. H. Lee, and Y. K. Chung. Tetrahedron Lett., 1991, 32, 2137. CrossRef
6. T. Asamizu, R. Naruse, G. Yongxue, and K. Kaneda, Tetrahedron Lett., 2015, 56, 4674. CrossRef
7. (a) J. K. Loh, N. Asad, T. B. Samarakoon, and P. R. Hanson, J. Org. Chem., 2015, 80, 9926; CrossRef (b) B. Niu, P. Xie, M. Wing, Y. Wang, W. Zhao, C. U. Pittman, Jr., and A. Zhou, Mol Divers., 2015, 19, 447; CrossRef (c) B. Gerard, J. R. Duvall, J. T. Lowe, T. Murillo, J. Wei, L. B. Akella, and L. A. Marcaurelle, ACS Comb. Sci., 2011, 13, 365. CrossRef
8. (a) K. Hagiya, N. Muramoto, T. Misaki, and T. Sugimura, Tetrahedron, 2009, 65, 6109; CrossRef (b) T. Sugimura and K. Hagiya, Chem. Lett., 2007, 36, 566. CrossRef
9. (a) O. Mitsunobu, Synthesis, 1981, 1, 1; CrossRef (b) K. C. K. Swamy, N. N. B. Kumar, E. Balaraman, and K. V. P. Kumar, Chem. Rev., 2009, 109, 2551. CrossRef
10. (a) T. Kan and T. Fukuyama, Chem. Commun., 2004, 353; CrossRef (b) T. Fukuyama, M. Cheung, and T. Kan, Synlett, 1999, 1301. CrossRef
11. (a) K. Ramadas and N. Srinivasan, Synth. Commun., 1992, 22, 3189; CrossRef (b) Z.-P. Xiao, Y.-C. Wnag, G.-Y. Du, J. Wu, T. Luo, and S.-F. Yi, Synth. Commun., 2010, 40, 661. CrossRef
12. K. Kaneda and T. Honda, Tetrahedron, 2008, 64, 11589. CrossRef
13. Deposition number CCDC-1433846 for 8b and 1433878 for 8c. (http://www.ccdc.cam.ac.uk/conts/retrieving.html)