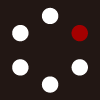
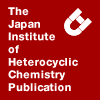
HETEROCYCLES
An International Journal for Reviews and Communications in Heterocyclic ChemistryWeb Edition ISSN: 1881-0942
Published online by The Japan Institute of Heterocyclic Chemistry
e-Journal
Full Text HTML
Received, 10th February, 2016, Accepted, 31st May, 2016, Published online, 13th June, 2016.
DOI: 10.3987/COM-16-13437
■ Preparation and Electrochemical Behavior of N-Substituted Phenothiazine Oxide
Hideki Hayashi* and Take-aki Koizumi
Organic Materials Laboratory, Nagoya Municipal Industrial Research Institute, 3-4-41, Rokuban, Atsuta-ku, Nagoya 456-0058, Japan
Abstract
The chemical properties of promazine oxide (Prom-O) and promazine (Prom) were compared. Cyclic voltammetry (CV) experiments showed a higher oxidation potential of Prom-O compared to that of Prom. The results of CV measurements also suggested the potential applicability of Prom-O as an n-type semiconductor.Polymers containing diphenylamine with a bridging structure, such as O,1,2 S,3-5 SO,4,5, SO2,5 -CH=CH-,2 -SiR2-,6-15 and -SiR2SiR2-,16 often show interesting optical and electrochemical properties, and thus have been widely researched. We previously reported the preparation, properties, and applications of Si17,18 and ethylene19,20 unit-bridged diphenylamine-containing polymers (Scheme 1).
We also reported the preparation of poly(dibenzazepine)s ((PAzep)s) by oxidative polymerization of the corresponding dibenzazepines ((Azep)s) and their use as a fluorescent additive for resins (Scheme 2). However, our efforts to synthesize poly(phenothiazine)s using the same method failed to give the desired polymer. In the case of phenothiazine, we hypothesized that the oxidation of phenothiazine occurred prior to the oxidative polymerization. Therefore, we attempted to analyze the oxidation products derived from phenothiazine.21 Mass and IR spectral data strongly indicated that phenothiazine oxide is produced under the oxidative polymerization conditions, as shown in Scheme 2. Since (PAzep)s are useful as functional additives for resins, the potential applications of the oxidation products of phenothiazine derivatives should also be investigated.
Promazine (N,N-dimethyl-3-(10H-phenothiazin-10-yl)-propan-1-amine, Prom) and its hydrochloride salt (Prom-HCl) are derivatives of phenothiazine that are easily available owing to studying for medical use.22,23 However, the oxidation of Prom and the chemical properties of the resulting products have not been investigated. In the present study, we have compared the optical and electrochemical properties of Prom-O and Prom.
In order to compare the physical and chemical properties of phenothiazine and phenothiazine oxide, it is preferable to introduce the same substituent on the N atom of the diphenylamine unit in the phenothiazine skeleton. From this point of view, we synthesized N-(3-trimethylammoniopropyl)-substituted phenothiazine (Prom-MeI) and its S-oxide derivative (Prom-O-MeI) from the common precursor Prom-HCl (Scheme 3). The oxidation of promazine hydrochloride using m-chloroperbenzoic acid (mCPBA)22 afforded 10-(3-(N,N-dimethylamino)-1-propyl)-10H-phenothiazine-5-oxide (promazine S-oxide, Prom-O). The 3-dimethylaminopropyl group of both Prom and Prom-O was converted ammonium salt to Prom-MeI and Prom-O-MeI, by the reported method.24 Prom-MeI shows low solubility in CHCl3, whereas Prom-O and Prom-O-MeI show good solubility.
The 1H NMR spectra of oxidized and unoxidized products obtained from Prom-HCl are shown in Figure 1. Prom was prepared by treatment of Prom-HCl with NaOH in water. In the case of Prom, quaternization of the alkylamine unit shifts the adjacent protons to a lower magnetic field (Hc, δ 2.4 to δ 3.9, Figures 1(a) and 1(b)). However, the other two signals associated with the alkyl chain do not change so much upon quaternization (Ha: δ 3.9; Hb: δ 1.9, Figure 1(a)). However, the signal assigned to Ar2NCH2CH2CH2NMe2 (δ 2.4, Hc in Figure 1(a)) is shifted to δ 3.8 (Figure 1(b)). Additionally, the quaternization of dimethylalkylamine unit of Prom changes the chemical shift of N–CH3 from δ 2.2 to δ 3.0.
In the case of phenothiazine and phenothiazine oxide, several peaks assigned to the aromatic protons of phenothiazine shifted to a lower magnetic field upon S-oxidation. In Prom-O, the signal assigned to Ha shifted to a lower magnetic field (δ 4.5, Figure 1(c)) as compared to that of Prom (δ 3.9, Figure 1(a)) by the formation of the S-oxide, whereas the chemical shifts of Hb, Hc, and -NMe were unaffected. The lower shift of Ha was promoted by the introduction of the electron-withdrawing S-oxide.
The electrochemical behavior of Prom-MeI and Prom-O-MeI was studied using cyclic voltammetry (CV). The redox responses of the compounds are shown in Figure 2. In the oxidative region, the CV of Prom-MeI and Prom-OMeI showed complicated redox wave. Because these compounds contain I- as a counter anion, the redox process of I-/I2 also appears at this region (cf. E1/2(I-/I2) = ca. +0.4 V vs AgCl/Ag in teraalkylammonium iodide).25 On the other hand, in contrast to Prom-MeI, Prom-O-MeI shows an irreversible reduction peak in the negative region at Epc = -2.77 V (vs. Fc+/Fc, Figure 2 (c)). Electrochemical properties of phenothiazine and phenothiazine oxide have been reported previously, however only the oxidation properties of the compounds have been described in the articles.5,26 We tried to investigate the reduction behavior of Prom-MeI and Prom-O-MeI. The results are shown in Figures 2(b) and 2(d). As shown in Figure 2(d), not only whole scan (Figure 2 (c)) but also negative scan show an irreversible reduction peak at Epc = -2.77 V (vs. Fc+/Fc) although Prom-MeI shows no reduction peak in the reduced region (Figure 2(b)). These results suggest that phenothiazine oxides have potential applications as n-type semiconducting materials.
The absorption spectra of Prom-O-MeI and Prom-MeI are shown in Figure 3, and the data are summarized in Table 1. Usually, N-substituted diphenylamines show absorption λmax corresponding to the π-π* transition at ca. 300 nm, for example, diphenylamine (λmax = 297 - 305 nm)27 and phenazasiline (λmax = 295 nm).28 In agreement with the literature, the π-π* transition of Prom-MeI is observed at λmax = 307 nm. However, the π-π* transition in Prom-O-MeI is red-shifted by ca. 35 nm (λmax = 341 nm) due to the narrow π-π* gap resulting from the S-oxide. Comparison of CV between Prom-MeI and Prom-O-MeI also agreed with the result.
Prom-O-MeI shows fluorescence at 369 nm in CHCl3 (Figure 3c), although Prom-MeI shows no fluorescence under the same conditions. Generally, an iodine ion works as a fluorescence quencher,29,30 therefore, fluorescent spectra of Prom and Prom-O were also measured. As shown in Table 1, Prom shows no fluorescence whereas Prom-O shows fluorescence at same wavelength with Prom-O-MeI. These results suggest that the difference of fluorescence properties between Prom and Prom-O comes from not existence of I- but formation of S-oxide mainly.
In summary, we have reported that diphenylamine with a bridged structure can be functionalized by the introduction of appropriate substituent(s) on the N atom.18 Furthermore, we found that the S atom of the phenothiazine unit can be functionalized to give the S-oxide using the mCPBA oxidation protocol. Since the S-containing π-conjugated system, thiophene, has been functionalized by oxidation to form thiophene S-oxide,31 functionalization of phenothiazine derivatives using the S-oxidation method and applications of the derivatives in are also expected.
EXPERIMENTAL
Prom-HCl was purchased from SIGMA. Other reagents were commercially available from either Tokyo Chemical Industry or Kanto Chemical Industry and used as received.
Measurement
NMR and IR spectra were recorded on Varian INOVA400 and JASCO FT/IR-410 spectrometers, respectively. Absorption and fluorescence spectra were recorded on JASCO UV-570 and Hitachi F 4010 spectrometers, respectively. Cyclic voltammetry (CV) was performed using a Hokuto Denko HSV-100 automatic polarization system.
Preparation of 10-(3-(trimethylammonio)-1-propyl)-10H-phenothiazine iodide (Prom-MeI)
Iodomethane (3 mL) was added to a benzene solution (10 mL) of Prom, which was prepared by the neutralization of Prom-HCl (1.08 g, 3.4 mmol) using sodium hydroxide, and stirred for 24 h to yield a yellow precipitate. The precipitate was separated by filtration, washed with hexane, and dried in vacuo to afford Prom-MeI as a yellow powder in 91% yield (1.31 g, 3.1 mmol, from Prom-HCl). Mp 241-244 °C (decomp.)
1H NMR (DMSO-d6) δ 7.15-7.40 (m, 4H, Ar), 7.05-7.15 (m, 2H, Ar), 6.90-7.05 (m, 2H, Ar), 3.94 (t, 2H, J = 7.0 Hz, ArN-CH2-), 3.40-3.60 (m, 2H, -CH2-NMe3), 3.02 (s, 9H, -NMe3), 2.00-2.20 (m, 2H, -CH2-CH2-CH2-).
13C NMR (DMSO-d6) δ 144.39, 127.76, 127.32, 123.87, 122.88, 115.90, 63.15, 52.32, 43.48, 20.21. IR (ATR) 3001, 2957, 2870, 1591, 1566, 1487, 1455, 1420, 1353, 1340, 1311, 1283, 1253, 1235, 1197, 1155, 1139, 1123, 1104, 1051, 1032, 962, 940, 917, 858, 759, 752, 728, 694, 672, 618 cm-1
Preparation of 10-(3-(N,N-dimethylamino)-1-propyl)-10H-phenothiazine-5-oxide (Prom-O)
Under N2 atmosphere, 0.69 g (2.15 mmol) of Prom-HCl was dissolved in 10 mL of CHCl3; then, 0.53 g (3.07 mmol) of m-chloroperbenzoic acid was added to the solution at 0 °C and stirred overnight. The reaction was stopped by the addition of aqueous NaOH, and the solution was extracted by CHCl3. The organic phase was dried over MgSO4, then the solvent was removed by evaporation. The residue was purified by alumina column chromatography (eluent: CHCl3) and dried in vacuo to afford Prom-O as a pale yellow powder in 83% yield (0.53 g, 1.8 mmol). Mp 114.5-116.0 °C.
1H NMR (CDCl3) δ:7.90-8.00 (m, 2H, Ar), 7.55-7.70 (m, 2H, Ar), 7.40-7.55 (m, 2H, Ar), 7.20-7.30 (m, 2H, Ar), 4.33 (t, 2H, J = 7.8 Hz,, ArN-CH2-), 2.47 (t, 2H, J = 6.8 Hz, -CH2-NMe2), 2.29 (s, 6H, -NMe2), 2.00-2.20 (m, 2H, -CH2-CH2-CH2-).
13C NMR (CDCl3) δ:138.35, 132.84, 131.69, 124.12, 121.72, 115.76, 56.65, 45.96, 45.69, 24.59.
IR (ATR) 2924, 2856, 2809, 2789, 2755, 1584, 1572, 1482, 1456, 1375, 1364, 1250, 1225, 1165, 1152, 1095, 1043, 1019, 967, 955, 897, 858, 826, 779, 764, 742, 706, 668, 634, 604 cm-1.
Preparation of 10-(3-(trimethylammonio)-1-propyl)-10H-phenothiazine-5-oxide iodide (Prom-O-MeI)
Iodomethane (0.2 ml) was added to a benzene solution (2.5 mL) of Prom-O (87.5 mg, 0.29 mmol) and stirred for 24 h to yield a colorless precipitate. The precipitate was separated by filtration, washed with hexane, and dried in vacuo to afford Prom-O-MeI as a colorless powder in 96% yield (123.4 mg, 0.28 mmol). Mp 245-247 °C (decomp.).
1H NMR (CDCl3) δ:7.80-7.90 (m, 2H, Ar), 7.60-7.80 (m, 4H, Ar), 7.20-7.35 (m, 2H, Ar), 4.66 (t, 2H, J = 5.7 Hz, ArN-CH2-), 3.60-3.75 (m, 2H, -CH2-NMe2), 3.02 (s, 9H, -NMe3), 2.30-2.50 (m, 2H, -CH2-CH2-CH2-).
13C NMR (CDCl3) δ:138.86, 133.40, 130.26, 127.23, 122.77, 117.95, 62.62, 53.65, 43.40, 22.04.
IR (ATR) 3006, 2957, 1586, 1575, 1489, 1462, 1380, 1254, 1200, 1178, 1148, 1102, 1053, 1023, 999, 971, 922, 907, 853, 786, 748, 708, 669, 627, 606 cm-1.
Electrochemical measurements
A conventional three-electrode configuration was used, with a glassy carbon electrode (BAS PFCE 3 carbon electrode), a platinum wire auxiliary electrode (Tokuriki, special order), and 0.1 M AgNO3/Ag reference (BAS RE-5). Cyclic voltammograms were recorded at a scan rate of 50 mV s-1. The sample concentration was 1 mM in DMF containing 0.1 M n-Bu4NBF4 as the supporting electrolyte.
ACKNOWLEDGEMENTS
A part of this research was performed under the Cooperative Research Program of "Network Joint Research Center for Materials and Devices."
References
1. Y. Ito, T. Shimada, J. Ha, M. Vacha, and H. Sato, J. Polym. Sci. A Polym. Chem., 2006, 44, 4338. CrossRef
2. T. Kanbara, Y. Yokokawa, and K. Hasegawa, J. Polym. Sci. A Polym. Chem., 2000, 38, 28. CrossRef
3. X. Kong, A. P. Kulkarni, and S. A. Jenekhe, Macromolecules, 2003, 36, 8992. CrossRef
4. L. Y. Yang, L. Q. Li, C. Wang, F. J. Zhu, Y. Lu, S. Janietz, A. Wedel, Y. L. Hua, and S. G. Yin, J. Lumin., 2007, 122-123, 714. CrossRef
5. L. Y. Yang, C. Wang, L. Q. Li, S. Janietz, A. Wedel, Y. L. Hua, and S. G. Yin, J. Polym. Sci. A Polym. Chem., 2007, 45, 4291. CrossRef
6. H. Kondo, M. Sato, and M. Yokoyama, J. Polym. Sci., Polym. Chem. Ed., 1983, 21, 165. CrossRef
7. G. Casalbore-Miceli, G. Beggiato, S. S. Emmi, A. Geri, S. Daolio, L. Favaretto, and D. Pietropaolo, J. Appl. Electrochem., 1990, 20, 989. CrossRef
8. G. Casalbore-Miceli, A. Geri, G. Giro, S. Daolio, and G. Zotti, Synth. Met., 1991, 40, 317. CrossRef
9. G. Casalbore-Miceli, G. Beggiato, N. Camainoi, L. Favaretto, D. Pietropaolo, and G. Poggi, Ann. Chim. (Rome), 1992, 82, 161.
10. M. Mastragostino, A. Zanelli, G. Casalbore-Miceli, and A. Geri, Synth. Met., 1995, 68, 157. CrossRef
11. K. Ono, T. Kobayashi, Y. Sato, K. Eguchi, S. Kato, N. Kishi, and T. Soga, Heterocycles, 2011, 83, 1977. CrossRef
12. Y. Fujinami, J. Kuwabara, W. Lu, H. Hayashi, and T. Kanbara, ACS Macro Lett., 2012, 1,67. CrossRef
13. W. Lu, J. Kuwabara, T. Iijima, H. Higashimura, H. Hayashi, and T. Kanbara, Macromolecules, 2012, 45, 4128. CrossRef
14. H. Nakao, H. Hayashi, T. Yoshino, S. Sugiyama, K. Otobe, and T. Ohtani, Nano Lett., 2000, 2, 475. CrossRef
15. H. Nakao, H. Hayashi, F. Iwata, H. Karasawa, K. Hirano, S. Sugiyama, and T. Ohtani, Langmuir, 2005, 21, 7945. CrossRef
16. K. Otobe, H. Nakao, H. Hayashi, F. Nihei, M. Yudasaka, and S. Iijima, Nano Lett., 2002, 2, 1157. CrossRef
17. H. Hayashi, H. Nakao, and K. Iida, Bunseki Kagaku, 2011, 60, 215. CrossRef
18. H. Hayashi, Koubunshi Ronbunsyu, 2006, 63. 529. CrossRef
19. H. Hayashi, K. Murakami, H. Inoue, H. Hirota, M. Harada, K. Iida, Y. Onouchi, and K. Hirano, Curr. Trends Polym. Sci., 2010, 14, 11.
20. H. Hayashi, Y. Ishigaki, J. Kuno, T. Naruo, H. Hirota, T. Ogawa, H. Hattori, H. Seno, Y. Onouchi, and M. Oda, J. Adhes. Soc. Jpn., 2015, 51, 298. CrossRef
21. H. Hayashi, H. Inoue, H. Nakao, H. Hattori, And Y. Onouchi, Int. J. Polym. Anal. Charact., 2012, 17, 189. CrossRef
22. T. Kanamori, K. Kuwayama, K. Tsujikawa, H. Miyaguchi, Y. Iwata, H. Inoue, and T. Kishi, Jpn. J. Forensic Sci. Tech., 2007, 12, 237. CrossRef
23. R. Aoki, T. Arinobu, T. Kumazawa, H. Hattori, and H. Noguchi, Forensic Toxicol., 2007, 25, 8. CrossRef
24. K. Hirano, H. Fukuda, and S. L. Regan, Langmuir, 1991, 7, 1045. CrossRef
25. I. S. El-Hallag, J. Chil. Chem. Soc., 2010, 55, 67.
26. K. D. Theriault and T. C. Sutherland, Phys. Chem. Chem. Phys., 2014, 16, 12266. CrossRef
27. T. Yamamoto, S.-B. Kim, and T.-a. Koizumi, Polym. J., 2009, 41, 810. CrossRef
28. H. Hayashi, M. Murase, T.-a. Koizumi, K. Ohara, T. Miyabayashi, and M. Kojima, Bull. Chem. Soc. Jpn., 2010, 83, 1282. CrossRef
29. T. Asakawa, K. Iriyama, A. Ohta, and S. Miyagishi, J. Oreo. Sci., 2004, 53, 445.
30. A. Chmyrov, T. Sandén, and J. Widengren, J. Phys. Chem. B, 2010, 114, 11282. CrossRef
31. T. Yamamoto, I. Nurulla, H. Hayashi, and H. Koinuma, Synth. Met., 1999, 107, 137. CrossRef