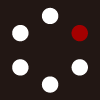
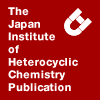
HETEROCYCLES
An International Journal for Reviews and Communications in Heterocyclic ChemistryWeb Edition ISSN: 1881-0942
Published online by The Japan Institute of Heterocyclic Chemistry
e-Journal
Full Text HTML
Received, 8th June, 2016, Accepted, 4th August, 2016, Published online, 18th August, 2016.
■ Oxidative Dearomatic Cyclization of N-Substituted Benzanilide Derivatives: Conformational Effect of Amide Groups on the Reaction
Kousuke Hayashi, Jun Takayama,* Meiyan Xuan, Misaki Suda, Hiroyuki Teramae, and Takeshi Sakamoto*
Faculty of Pharmaceutical Sciences, Josai University, 1-1 Keyakidai, Sakado, Saitama 350-0295, Japan
Abstract
The synthesis of spirooxindoles with a hypervalent iodine reagent depended on N-substituted benzanilide derivatives as starting materials. Reaction yields of benzanilides containing various N-substituents were discovered to relate to the cis and trans conformations of the amide bond by ab initio molecular orbital calculations at the B3LYP/6-31G(d,p) and MP2/6-31G(d,p) levels, including full geometry optimizations. The relationship between the reaction and conformation of the starting material by quantum chemical calculations was applied to the formal synthesis of SR121463.In recent years, many new biologically active spirooxindoles have been discovered.1 The unique core structure and bioactive nature of these compounds have long been a focus of pharmaceutical development. One of these, named SR121463 1,2 is manufactured by Sanofi and is a V2 receptor antagonist (Figure 1).
The synthesis of a SR121463 intermediate 2 with N-methyl and benzyl protecting groups, has also been published using oxidative dearomatic reaction with hypervalent iodine reagent by Yu and co-workers in 2011.3 This intermediate is useful in the synthesis of bioactive natural products such as phenanthridinone derivatives4 using the dienone-phenol rearrangement,5 and synthesis of a hydrocarbazole skeleton6 by ring opening reaction of a lactam followed by aza-Michael addition (Figure 2). However, commercial applications utilizing intermediate 2 are inherently difficult due to issues with scaling up of the synthesis. For example, deprotection of the nitrogen when R is benzyl requires severe conditions such as in the Birch reduction.7 Removal of protecting groups in total syntheses of bioactive natural products should proceed under mild conditions, otherwise the constructed structures will decompose. Therefore, the initial focus of this paper was to explore better protecting groups for intermediate 2. Also, in the course of the screening, we employed computational methods to optimize our synthesis.
Scheme 1 shows the synthesis of spirooxindole derivatives 4 from benzanilide derivatives 3 with hypervalent iodine reagent. Cyclization reaction by phenol oxidation with hypervalent iodine reagent has been proposed via two synthetic pathways, path A and path B.8 However, neither route has been confirmed despite numerous studies.9 In path A, cyclization, dearomatization and elimination reactions take place in order. In path B, on the other hand, phenoxenium cation is generated prior to the electrophilic aromatic substitution reaction. Compound 3 is generated from 4-hydroxybenzoic acid and aniline derivatives. The synthesis is designed and performed as described below.
First, possible candidates for the starting material, 3a-3f (Table 1), with various N-substituents were selected. N-Methyl and benzyl compounds, 3a-3d, were prepared following published procedures.3 Reported compounds 3a-3d were synthesized to identify correlations between yield of cyclization reaction and amide conformation. The remaining two starting materials, N-methoxybenzanilide derivative 3e10 and N-phthaloylbenzanilide derivative 3f,10 were generated from 4-acetoxybenzoic acid11 in 3 steps including arylation via nitrenium ion12 with hypervalent iodine reagent. We also included two N-protecting groups reported in the literature: N-methoxy and N-phthaloyl groups. It was reported that N-methoxy group was easily deprotected under mild conditions such as hydrogenation,13 and N-phthaloyl group was removed in two steps, hydrolysis with hydrazine followed by reduction.14
Second, the oxidative dearomatization reaction of the benzanilides was screened using 3a-3c, 3e and 3f in 4 different solvents, (CF3)2CHOH, CF3CH2OH, MeOH, and CH2Cl2. Compound 3d was also investigated in MeCN as a solvent. The yield of products 4a-4d in Table 1 shows that phenol oxidation with 3a-3d afforded the desired spiro compounds 4a-4d with excellent yields (entries 1-16), whereas the yield of the cyclization reaction with N-methoxy compound 3e was much lower (entries 17-20). The amide 3f produced spirooxindole 4f10 in moderate yield (entries 21-24). PhI(OAc)2 (PIDA) was evaluated but it was found to be less effective than PhI(OCOCF3)2 (PIFA) (entry 15, 16). The result of the cyclization reactions showed that the differences between the yields of spirooxindole 4a-4f depended on the various N-substituents in the starting materials. N-Methyl and benzyl compounds (3a-3d) afforded the best yields. However, it was not possible to predict which N-substituent would work better for the cyclization reactions.
In order to build a better predictive model, the impact of amide conformations (Figure 3) in the cyclization reactions was investigated. It was reported that cis N-methylbenzanilide is the predominant conformer according to NMR analysis.15
The cis-trans conformations of each candidate material (3a-3f) were determined by employing computational methods, specifically quantum chemical calculations. Both ab initio DFT molecular orbital calculations at the B3LYP/6-31G(d,p) level and ab initio molecular orbital calculations at the MP2/6-31G(d,p) level using the Gaussian09 program package16 can estimate the energetics of each conformation to determine the most stable form, and both tools were used to identify the cis-trans conformations of all starting materials (3a-3f). Note that the complete analysis for all conformations, including methoxy groups, were not performed at this time. One of the optimized structures of cis and trans conformers for each compound were calculated. The energetics would vary a little if complete calculations were performed on the most stable cis and trans conformers. Such calculations using Hamiltonian algorithm17 is in progress and will be published elsewhere. The trans-cis ratio was estimated by the Boltzmann distribution law at 300 K (kT product is 0.596 kcal/mol at 300 K). The calculated results were compared to the yields of synthetic products 4a-4f (Table 2).
The result of the calculations correlates very closely with the yields of the cyclization reaction. According to the data, amides 3a-d, which are predominantly cis compounds, gave high yields of the products 4a-d. On the other hand, benzamide 3e with the N-methoxy group provided a low yield of 4e. The dipole moments of the carbonyl and methoxy groups are one of the possible factors contributing to the trans-conformation of N-methoxyamide 3e (Figure 4). In addition, steric hindrance of the methoxy group is smaller than the methyl group allowing easier cis to trans conformational change. The calculated results for N-phthaloylamide 3f show that the trans-conformation is predominant, probably due to both dipole moments and steric hindrance.
These calculations provide a practical way to identify cis-trans conformations of compounds to find better starting materials to generate products with high yields, however no one has yet reported utilizing quantum chemical calculations for cis-trans conformations of amide bonds. Such calculations are useful, since the reaction points in the cis form of benzanilide derivatives are closer than those in the trans form, hence the higher yields.
Although the starting materials that showed high yields of the products so far were 3a-3d, they are not actually ideal compounds since it is known that the N-methyl group cannot be removed and deprotecting the N-benzyl group requires severe conditions. Therefore, more effective N-protecting groups were investigated as the next step. Synthesis of a spirooxindole with the N-methoxymethyl (MOM) group, which can be deprotected using acid hydrolysis, was evaluated. Initial screening was performed using ab initio DFT molecular orbital calculations on N-methoxymethylbenzanilide derivatives to find possible compounds with the cis form, and two candidates, 3g and 3h, were selected (Table 3).
N-Methoxymethyl compounds (3g, 3h) were obtained from 4-benzyloxybenzanilide derivatives in 2 steps following literature procedures.18 After the oxidative dearomatization reaction of compounds 3g, 3h, spirooxindole derivatives 4g, 4h were obtained in excellent yields (Table 4).
The oxidative dearomatization reaction of N-methoxymethylbenzanilide 3h was applied to the synthesis of SR121463 intermediate 6 (Scheme 2). Catalytic hydrogenation of spirodienone 4h in ethyl acetate at room temperature produced spirocyclohexanone 5 in >99% yield. The deprotection of the methoxymethyl group was carried out by treating 5 with trimethylsilyl iodide in acetonitrile at ambient temperature and triethylamine in methanol at 55 °C to give intermediate 6.19 The structure of 620 was confirmed by spectroscopic data, which was identical to reported data by Curran and co-workers.21 Therefore, the synthesis afforded 6 under mild conditions.
In summary, we presented a concise synthesis of a SR121463 intermediate under mild conditions by oxidative dearomatization cyclization with hypervalent iodine reagent as a key step. It is proposed that N-MOM will be a better protecting group, not only for synthesis of the intermediate 2, but perhaps also for other bioactive compounds since the deprotection can be performed under mild conditions. Hypervalent iodine reagent could also react with the nitrogen of the amide, however, we believe N-Me, Bn and MOM substituted amides were sufficiently protected, allowing the reaction to occur on the hydroxy group in the spirooxindole synthesis. In addition, we established a practical way to identify cis-trans conformations of amides to screen for better reactive compounds to give higher yields. We identified a correlation between experimental data and ab initio DFT molecular orbital calculations, and successfully identified better benzamide derivatives for the target compound. This correlation suggests more applicability of these calculations to screen compounds for improved reactivity. This is also the first report to demonstrate that the cis-trans conformations of amide bonds can have an effect on the yield of oxidative dearomatization cyclizations.
ACKNOWLEDGEMENTS
We would like to thank Dr. Jeffrey Stewart and Dr. Aeri Park for assistance with preparation of the manuscript.
References
01. (a) C. Marti and E. M. Carreira, Eur. J. Org. Chem., 2003, 2209; CrossRef (b) C. V. Galliford and K. A. Scheidt, Angew. Chem. Int. Ed., 2007, 46, 8748; CrossRef (c) L. Hong and R. Wang, Adv. Synth. Catal., 2013, 355, 1023. CrossRef
02. C. Serradeil-Le Gal, C. Lacour, G. Valette, G. Garcia, L. Foulon, G. Galindo, L. Bankir, B. Pouzet, G. Guillon, C. Barberis, D. Chicot, S. Jard, P. Vilain, C. Garcia, E. Marty, D. Raufaste, G. Brossard, D. Nisato, J. P. Maffrand, and G. LeFur, J. Clin. Invest., 1996, 98, 2729. CrossRef
03. Z. Yu, X. Ju, J. Wang, and W. Yu, Synthesis, 2011, 860. CrossRef
04. (a) D. Weltin, V. Picard, K. Aupeix, M. Varin, D. Oth, J. Marchal, P. Dufour, and P. Bischoff, Int. J. Immunopharmacol., 1995, 17, 265; CrossRef (b) T. Harayama, H. Akamatsu, K. Okamura, T. Miyagoe, T. Akiyama, H. Abe, and Y. Takeuchi, J. Chem. Soc., Perkin Trans. 1, 2001, 523; CrossRef (c) J. Karthikeyan and C.-H. Cheng, Angew. Chem. Int. Ed., 2011, 50, 9880; CrossRef (d) S. Mishra, S. De, B. N. Kakde, D. Dey, and A. Bisai, Indian J. Chem., 2013, 52A, 1093; (e) K. Tanimoto, N. Nakagawa, K. Takeda, M. Kirihata, and S. Tanimori, Tetrahedron Lett., 2013, 54, 3712; CrossRef (f) Z. Liang, J. Zhang, Z. Liu, K. Wang, and Y. Zhang, Tetrahedron, 2013, 69, 6519; CrossRef (g) M. Yuan, L. Chen, J. Wang, S. Chen, K. Wang, Y. Xue, G. Yao, Z. Luo, and Y. Zhang, Org. Lett., 2015, 17, 346; CrossRef (h) M. Feng, B. Tang, N. Wang, H.-X. Xu, and X. Jiang, Angew. Chem. Int. Ed., 2015, 54, 14960. CrossRef
05. (a) S. Bo, M. Deng, and Q. Wang, Org. Lett., 2013, 15, 1606; CrossRef (b) M. Yoshida, T. Nozaki, T. Nemoto, and Y. Hamada, Tetrahedron, 2013, 69, 9609. CrossRef
06. J.-Y. Du, C. Zeng, X.-J. Han, H. Qu, X.-H. Zhao, X.-T. An, and C.-A. Fan, J. Am. Chem. Soc., 2015, 137, 4267. CrossRef
07. (a) H. Venkatesan, M. C. Davis, Y. Altas, J. P. Snyder, and D. C. Liotta, J. Org. Chem., 2001, 66, 3653; CrossRef (b) T. Ohgi and S. M. Hecht, J. Org. Chem., 1981, 46, 1232; CrossRef (c) M. Y. Kim, J. E. Starrett, Jr., and S. M. Weinreb, J. Org. Chem., 1981, 46, 5383; CrossRef (d) S. Sugasawa and T. Fujii, Chem. Pharm. Bull., 1958, 6, 587; CrossRef (e) P. A. Jacobi, H. L. Brielmann, and S. I. Hauck, Tetrahedron Lett., 1995, 36, 1193; CrossRef (f) R. M. Williams and E. Kwast, Tetrahedron Lett., 1989, 30, 451; CrossRef (g) M. Barbier, Heterocycles, 1985, 23, 345; CrossRef (h) J. E. Semple, P. C. Wang, Z. Lysenko, and M. M. Joullié, J. Am. Chem. Soc., 1980, 102, 7505; CrossRef (i) E. G. Baggiolini, H. L. Lee, G. Pizzolato, and M. R. Uskokovic, J. Am. Chem. Soc., 1982, 104, 6460; CrossRef (j) G. F. Field, W. J. Zally, L. H. Sternbach, and J. F. Blount, J. Org. Chem., 1976, 41, 3853. CrossRef
08. (a) J. S. Swenton, A. Callinan, Y. Chen, J. J. Rohde, M. L. Kerns, and G. W. Morrow, J. Org. Chem., 1996, 61, 1267; CrossRef (b) Y. Kita, M. Arisawa, M. Gyoten, M. Nakajima, R. Hamada, H. Tohma, and T. Takada, J. Org. Chem., 1998, 63, 6625; CrossRef (c) Y. Kita, YAKUGAKU ZASSHI, 2002, 122, 1011. CrossRef
8. (d) A. M. Harned, Tetrahedron Lett., 2014, 55, 4681. CrossRef
09. (a) L. Kürti, P. Herczegh, J. Visy, M. Simonyi, S. Antus, and A. Pelter, J. Chem. Soc., Perkin Trans. 1, 1999, 379;; CrossRef (b) Y.-T. Wang, K. J. Jin, S. H. Leopold, J. Wang, H.-L. Peng, M. S. Platz, J. Xue, D. L. Phillips, S. A. Glover, and M. Novak, J. Am. Chem. Soc., 2008, 130, 16021;; CrossRef (c) P. J. Hanway and A. H. Winter, J. Am. Chem. Soc., 2011, 133, 5086;; CrossRef (d) P. J. Hanway, J. Xue, U. Bhattacharjee, M. J. Milot, Z. Ruixue, D. L. Phillips, and A. H. Winter, J. Am. Chem. Soc., 2013, 135, 9078. CrossRef
10. 3e: mp 166-168 ºC; 1H NMR (400 MHz, CD3OD) δ 7.40 (d, 2H, J = 8.8 Hz), 7.22 (d, 2H, J = 8.8 Hz), 6.90 (d, 2H, J = 8.8 Hz), 6.68 (d, 2H, J = 8.8 Hz), 4.02 (q, 2H, J = 7.0 Hz), 3.70 (s, 3H), 1.37 (t, 3H, J = 7.0 Hz); 13C NMR (100 MHz, CD3OD) δ 170.0, 161.5, 160.2, 133.7, 132.1, 129.5, 125.9, 115.9, 115.7, 64.8, 61.4, 15.0; IR (KBr) 3224, 2939, 1635, 1597, 1512, 1442, 1350, 1257, 1226, 1165 cm-1; HRMS (EI) Calcd for C16H17NO4 287.1158, Found 287.1157; Anal. Calcd for C16H17NO4: C, 66.89; H, 5.96; N, 4.88. Found: C, 66.87; H, 5.94; N, 4.85. 3f: mp 139-142 ºC; 1H NMR (400 MHz, CDCl3) δ 7.86 (d, 2H, J = 3.2 Hz), 7.77-7.74 (m, 2H), 7.44-7.40 (m, 2H), 7.36 (d, 2H, J = 8.6 Hz), 6.89-6.77 (m, 3H), 6.59 (d, 2H, J = 8.6 Hz), 3.97 (q, 2H, J = 7.0 Hz), 1.37 (t, 3H, J = 7.0 Hz); 13C NMR (100 MHz, CDCl3) δ 165.1, 158.9, 158.6, 134.8, 131.7, 129.9, 129.7, 129.2, 124.0, 123.8, 120.6, 115.0, 113.0, 63.7, 14.6; IR (KBr) 3317, 2985, 1735, 1651, 1604, 1512, 1327, 1280, 1242, 1172, 1111 cm-1; HRMS (EI) Calcd for C23H18N2O5 402.1216, Found 402.1199; Anal. Calcd for C23H18N2O5: C, 68.65; H, 4.51; N, 6.96. Found: C, 68.36; H, 4.79; N, 6.68. 4e: mp 99-102 ºC; 1H NMR (400 MHz, CD3OD) δ 7.11 (d, 1H, J = 8.4 Hz), 7.00 (dd, 1H, J = 8.8 Hz and 2.4 Hz), 6.79 (d, 2H, J = 9.8 Hz), 6.69 (d, 1H, J = 2.4 Hz), 6.53 (d, 2H, J = 9.8 Hz), 4.04 (s, 3H), 3.97 (q, 2H, J = 7.0 Hz), 1.34 (t, 3H, J = 7.0 Hz); 13C NMR (100 MHz, CD3OD) δ 187.1, 167.8, 158.2, 145.4, 134.3, 132.3, 125.4, 116.7, 113.3, 110.5, 65.3, 64.4, 56.2, 15.1; IR (KBr) 3070, 2985, 2939, 2877, 1743, 1666, 1604, 1473, 1404, 1280, 1211, 1072 cm-1; HRMS (EI) Calcd for C16H15NO4 285.1001, Found 285.1029; Anal. Calcd for C16H15NO4: C, 67.36; H, 5.30; N, 4.91. Found: C, 67.23; H, 5.31; N, 4.86. 4f: mp 219-220 ºC; 1H NMR (400 MHz, CDCl3) δ 8.02-7.99 (m, 2H), 7.90-7.88 (m, 2H), 6.87 (dd, 1H, J = 8.4 Hz and 2.4 Hz), 6.84 (d, 2H, J = 10.0 Hz), 6.77 (d, 1H, J = 8.4 Hz), 6.69 (d, 1H, J = 2.4 Hz), 6.61 (d, 2H, J = 10.0 Hz), 3.97 (q, 2H, J = 7.0 Hz), 1.39 (t, 3H, J = 7.0 Hz); 13C NMR (100 MHz, CDCl3) δ 184.7, 168.9, 163.2, 156.9, 142.5, 135.3, 133.8, 131.6, 129.6, 124.7, 124.4, 115.6, 112.0, 109.6, 64.1, 54.3, 14.6; IR (KBr) 2978, 1797, 1743, 1666, 1496, 1458, 1273, 1165 cm-1; HRMS (EI) Calcd for C23H16N2O5 400.1059, Found 400.1039; Anal. Calcd for C23H16N2O5 ;C, 69.00; H, 4.03; N, 7.00. Found: C, 68.72; H, 4.19; N, 6.83.
11. C. H. R. M. Wilsens, B. A. J. Noordover, and S. Rastogi, Polymer, 2014, 55, 2432. CrossRef
12. (a) Y. Kikugawa and M. Kawase, Chem. Lett., 1990, 581; CrossRef (b) R. Samanta, J. O. Bauer, C. Strohmann, and A. P. Antonchick, Org. Lett., 2012, 14, 5518;; CrossRef (c) M. Kawase, T. Kitamura, and Y. Kikugawa, J. Org. Chem., 1989, 54, 3394; CrossRef (d) K. Murata, M. Tsukamoto, T. Sakamoto, S. Saito, and Y. Kikugawa, Synthesis, 2008, 32. CrossRef
13. X. Zhou, T. Xiao, Y. Iwama, and Y. Qin, Angew. Chem. Int. Ed., 2012, 51, 4909. CrossRef
14. Y. Kikugawa, A. Nagashima, T. Sakamoto, E. Miyazawa, and M. Shiiya, J. Org. Chem., 2003, 68, 6739. CrossRef
15. (a) I. Azumaya, K. Yamaguchi, H. Kagechika, S. Saito, A. Itai, and K. Shudo, YAKUGAKU ZASSHI, 1994, 114, 414;; (b) I. Azumaya, YAKUGAKU ZASSHI, 2001, 121, 117;; CrossRef (c) R. Yamasaki, A. Tanatani, I. Azumaya, H. Masu, K. Yamaguchi, and H. Kagechika, Cryst. Growth Des., 2006, 6, 2007. CrossRef
16. Gaussian 09, Revision C.01, M. J. Frisch, G. W. Trucks, H. B. Schlegel, G. E. Scuseria, M. A. Robb, J. R. Cheeseman, G. Scalmani, V. Barone, B. Mennucci, G. A. Petersson, H. Nakatsuji, M. Caricato, X. Li, H. P. Hratchian, A. F. Izmaylov, J. Bloino, G. Zheng, J. L. Sonnenberg, M. Hada, M. Ehara, K. Toyota, R. Fukuda, J. Hasegawa, M. Ishida, T. Nakajima, Y. Honda, O. Kitao, H. Nakai, T. Vreven, J. A. Montgomery Jr., J. E. Peralta, F. Ogliaro, M. Bearpark, J. J. Heyd, E. Brothers, K. N. Kudin, V. N. Staroverov, R. Kobayashi, J. Normand, K. Raghavachari, A. Rendell, J. C. Burant, S. S. Iyengar, J. Tomasi, M. Cossi, N. Rega, M. J. Millam, M. Klene, J. E. Knox, J. B. Cross, V. Bakken, C. Adamo, J. Jaramillo, R. Gomperts, R. E. Stratmann, O. Yazyev, A. J. Austin, R. Cammi, C. Pomelli, J. W. Ochterski, R. L. Martin, K. Morokuma, V. G. Zakrzewski, G. A. Voth, P. Salvador, J. J. Dannenberg, S. Dapprich, A. D. Daniels, Ö. Farkas, J. B. Foresman, J. V. Ortiz, J. Cioslowski, and D. J. Fox, Gaussian, Inc., Wallingford CT, 2009.
17. K. Ohtawara and H. Teramae, Chem. Phys. Lett., 2004, 390, 84. CrossRef
18. A. Bodzioch, M. Zheng, P. Kaszynski, and G. Utecht, J. Org. Chem., 2014, 79, 7294. CrossRef
19. S. Yokoshima, H. Tokuyama, and T. Fukuyama, Angew. Chem. Int. Ed., 2000, 39, 4073. CrossRef
20. 4h: mp 110-112 °C; 1H NMR (400 MHz, CDCl3) δ 7.04 (d, 1H, J = 8.8 Hz), 6.89 (dd, 1H, J = 8.8 Hz and 2.8 Hz), 6.62 (d, 1H, J = 2.8 Hz), 6.62 (d, 2H, J = 10.2 Hz), 6.53 (d, 2H, J = 10.2 Hz), 5.15 (s, 2H), 3.96 (q, 2H, J = 6.9 Hz), 3.35 (s, 3H), 1.37 (t, 3H, J = 6.9 Hz); 13C NMR (100 MHz, CDCl3) δ 185.1, 171.6, 156.3, 143.3, 135.1, 131.4, 126.8, 115.6, 111.8, 111.2, 72.2, 64.2, 56.5, 56.4, 14.7; IR (KBr) 2939, 1728, 1666, 1496, 1327, 1273, 1188, 1087 cm-1; HRMS (EI) Calcd for C17H17NO4 299.1158, Found 299.1176; Anal. Calcd for C17H17NO4: C, 68.22; H, 5.72; N, 4.68. Found: C, 68.28; H, 5.62; N, 4.73. 5: mp 115-116 °C; 1H NMR (400 MHz, CDCl3) δ 6.98 (d, 1H, J = 8.6 Hz), 6.85 (d, 1H, J = 2.4 Hz), 6.82 (dd, 1H, J = 8.6 Hz and 2.4 Hz), 5.13 (s, 2H), 4.00 (q, 2H, J = 7.2 Hz), 3.34 (s, 3H), 3.19-3.11 (m, 2H), 2.49 (dt, 2H, J = 15.2 Hz and 5.4 Hz), 2.19-2.14 (m, 4H), 1.41 (t, 3H, J = 7.2 Hz); 13C NMR (100 MHz, CDCl3) δ 210.4, 179.6, 155.7, 134.2, 133.9, 113.0, 111.0, 110.1, 71.2, 64.1, 56.2, 46.1, 36.8, 33.8, 14.9; IR (KBr) 2939, 1705, 1597, 1489, 1450, 1342, 1327, 1188, 1095 cm-1; HRMS (EI) Calcd for C17H21NO4 303.1471, Found 303.1474; Anal. Calcd for C17H21NO4: C, 67.31; H, 6.98; N, 4.62. Found: C, 67.19; H, 6.97; N, 4.65. 6: mp 192-194 °C; 1H NMR (400 MHz, CDCl3) δ 8.36 (s, 1H), 6.86 (d, 1H, J = 8.4 Hz), 6.83 (d, 1H, J = 2.4 Hz), 6.77 (dd, 1H, J = 8.4 Hz and 2.4 Hz), 4.00 (q, 2H, J = 7.0 Hz), 3.20-3.12 (m, 2H), 2.50 (dt, 2H, J = 15.2 Hz and 5.5 Hz), 2.25-2.11 (m, 4H), 1.41 (t, 3H, J = 7.0 Hz); 13C NMR (100 MHz, CDCl3) δ 210.7, 181.8, 155.2, 135.0, 133.2, 112.9, 111.1, 110.3, 64.1, 46.3, 36.8, 33.5, 14.8; IR (KBr) 3163, 3047, 2901, 1712, 1697, 1604, 1489, 1465, 1303, 1203, 1049; HRMS (EI) Calcd for C15H17NO3 259.1208, Found 259.1212; Anal. Calcd for C15H17NO3: C, 69.48; H, 6.61; N, 5.40. Found: C, 69.32; H, 6.46; N, 5.38.
21. F. González-López de Turiso and D. P. Curran, Org. Lett., 2005, 7, 151. CrossRef