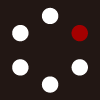
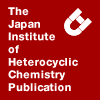
HETEROCYCLES
An International Journal for Reviews and Communications in Heterocyclic ChemistryWeb Edition ISSN: 1881-0942
Published online by The Japan Institute of Heterocyclic Chemistry
e-Journal
Full Text HTML
Received, 25th July, 2016, Accepted, 19th August, 2016, Published online, 25th August, 2016.
■ Synthesis of Nitriles from Aldehydes with Trimethylphenylammonium Tribromide and Ammonium Acetate
Shinsei Sayama*
Natural Sciences (Chemistry), Fukushima Medical University, Hikarigaoka, Fukushima, 960-1295, Japan
Abstract
Various aromatic and heterocyclic aldehydes were easily converted to respective nitriles with the combination of trimethylphenylammonium tribromide and ammonium acetate in good yields at room temperature.Nitriles have been known to be effective for the synthetic intermediates of amides, amines, esters2 and for the preparation of bioactive heterocycles such as imidazoles, tetrazoles, thiazoles.3 Aromatic nitriles from aryl diazonium salts were synthesized by Sandmeyer reaction and other alkyl nitriles were also prepared by the nucleophilic reaction of alkyl halides with inorganic cyanides.
As those methods using a stoichiometric amounts of inorganic cyanides were harmful and hazardous, there have been reported alternative methods such as methylarenes with NBS,4a aryl halides with CuSCN,4b or Zn (CN)2,4c and alkyl halides with TBACN.4d Further, convenient transformation of primary alcohols,5a,5b amines,5b,5c and oxiranes5d to nitriles was also reported respectively.
Moreover transformations of aldehydes to nitriles have been studied independently.6 The methods for producing nitriles via aldehyde derivatives such as aldoximes,7 aldehyde N-tosylimines,8a aldehyde trimethylhydrazonium iodides,8b were also investigated. The Fe3O4-catalyzed one-pot three-component synthesis of -aminonitriles from aldehydes, amines, and TMSCN was reported.9 The simple and economical synthesis of nitriles from aldehydes with hydroxylamine hydrochloride catalyzed by KF/Al2O3 was explored.10 Therefore, there is still considerable interest in investigating an alternative synthesis of various nitriles from aldehydes.
On the other hand, the methods for the oxidation of secondary alcohols to ketones,11a the chemoselective conversion of aromatic epoxide to 1,3-dioxane derivatives,11b and the transformation of alkoxyfurans to 3(2H)-furanones11c,11d were achieved with commercially available trimethylphenylammonium tribromide (phenyltrimethylammonium tribromide, PTAB). The regioselective one-pot synthesis of 6-bromobenzothiazoles form arylaldehydes was also achieved with PTAB-SbBr3.12 The oxidation of carbohydrates to keto-sugars was recently developed with PTAB-K2CO3 in the presence of organotin catalyst.13 Thus, the use of PTAB was expected to be attractive in oxidative organic syntheses. Zhu and Cai reported the synthesis of nitrile with tetrabutylammonium tribromide in aqueous ammonia.14 Therefore, it was seemed to be significant in finding a new oxidative procedure for preparation of nitriles from aldehydes with PTAB. Recently preparation of aromatic nitriles from aldehydes with pentylpridinium tribromide in aqueous NH4OAc was also reported by Bagherzade, Zali, and Sokrolahi.15 As we have also presented preliminary alternative reports for conversion of aldehydes to nitriles, we would like to report on the results of our studies concerning the one-pot synthesis of heterocyclic and aromatic nitriles from aldehydes with PTAB-NH4OAc.1
At first, the reaction of 2-pyridinecarbaldehyde (1), chosen as a representative heterocyclic aldehyde for this study, was carried out with various molar ratios of PTAB and NH4OAc over 1 for obtaining 2-pyridinenitrile (2). The results are summarized in Table 1. At 2.0 molar ratios of PTAB and 10.0 molar ratios of NH4OAc over 1 in CH2Cl2 at room temperature, 2-pyridinenitrile (2) was afforded in good yield (run 1). To examine the optimum amounts of PTAB for the synthesis of nitrile 2, the reaction of 1 with 0.0-1.5 molar ratios of PTAB was carried out in the presence of 10.0 molar ratios of NH4OAc over 1. At 1.5 molar ratios of PTAB, the yield of 2 was 92%, accompanied by small amounts of recovered 1 (run 2). A mixture of nitrile 2 (84%) and recovered 1 (10%) was afforded at 1.0 molar ratio of PTAB over 1 (run 3). A complex mixture was given without PTAB under the same reaction conditions (run 4). Consequently, there is need to use at least more than 1.5 molar equivalents of PTAB over 1 for obtaining nitrile 2 in moderate yield.
To clarify the optimum amounts of NH4OAc for conversion of 1 to nitrile 2, the reaction of 1 with 1.0-8.0 molar ratios of NH4OAc was also carried out in the presence of 2.0 molar ratios of PTAB over 1 respectively. At 8.0 molar ratios of NH4OAc over 1, the reaction of 1 with PTAB gave nitrile 2 in 95% yield(run 5). At 4.0-6.0 molar ratios of NH4OAc over 1, nitrile 2 was afforded in 84-87% yields, accompanied by 8-9% recovered 1 (runs 6, 7). The yields of 2 were not fully satisfactory, accompanied by recovered 1 (29-48%) at 1.0-2.0 molar ratios of NH4OAc in the presence of 2.0 molar ratios of PTAB over 1 (runs 8, 9). The satisfactory yield of nitrile 2 was not observed at 1.0 molar ratio of PTAB and 6.0 molar ratio of NH4OAc (run 10). In the present experiments, there is need to use at least 6.0-10.0 equivalents of NH4OAc in the presence of 2.0 equivalents of PTAB over 1 for producing nitriles 2 quantitatively. Further, the reaction of 1 with ammonium oxalate or NH4Cl instead of NH4OAc was carried out to examine the effect of NH4OAc in this method. At 6.0 molar ratios of ammonium oxalate in the presence of 2.0 molar ratios of PTAB, the yield of nitrile 2 was not fully satisfactory accompanied by recovered 1 (run 11). At 10.0 molar ratios of NH4Cl, aldehyde 1 was recovered unchanged under the same reaction conditions (run 12). Accordingly, this one-pot synthesis of 2-pyridinenitrile 2 from
2-pyridinecarbaldehyde 1 was suggested to rest on the complemental function of PTAB and NH4OAc.
To test the suitable solvents in this method, the conversion of 1 to 2 was carried out with PTAB-NH4OAc in various solvents such as MeOH, MeCN, hexane, benzene, H2O under the same reaction conditions. In MeOH, MeCN, hexane, 1 was converted to 2 in good yields respectively (runs 13-15). A mixture of nitrile 2 (48%) and recovered aldehyde 1 (47%) was afforded in benzene (run 16). H2O was also found to be appropriate solvent for conversion of 1 to 2 (run 17). Consequently, it was found that the preparation of nitrile 2 from aldehyde 1 by PTAB-NH4OAc was not rest on solvents excepting for benzene.
To examine the effect of ammonium tribromides, the conversion of 1 to 2 was carried out with pyridinium hydrobromide perbromide(PHPB) instead of PTAB in the presence of NH4OAc in various solvents. Nitrile 2 was respectively given with PHPB in 92-94% yields in CH2Cl2, MeOH, MeCN, hexane, H2O under the same reaction conditions (runs 18-22). Accordingly, the combination of ammonium tribromides, PTAB or PHPB and NH4OAc was confirmed to be alternative convenient one-pot procedure for conversion of 2-pyridinecarbaldehyde 1 to 2-pyridinenitrile 2.
To elucidate the limitations for this conversion of heterocyclic aldehydes to nitriles, the reaction of various heterocyclic aldehydes was examined with PTAB-NH4OAc. The results of the reaction of heterocyclic aldehydes are shown in Table 2. The reaction of 3-pyridinecarbaldehyde (3), 4-pyridinecarbaldehyde (5), and 6-methyl-2-pyridinecarbaldehyde (7) took place to give corresponding nitriles (4), (6), (8) respectively (runs 2-4). 2,6-Pyridinedicarbaldehyde (9) was similarly converted to dinitrile(10) in good yield (run 5).
The reaction of quinolinecarbaldehydes, 2-formylthiazole was also carried out with PTAB-NH4OAc to clarify the chemoselectivity for conversion of heterocyclic aldehydes to nitriles. The reaction of 2-, 3-, 4-, and 8-quinolinecarbaldehydes (11), (13), (15), (17) also took place to give corresponding nitriles (12), (14), (16), (18) in good yields (runs 6-9). 2-Formylthiazole (19) was converted to nitrile (20)(run 10).
A variety of heterocyclic nitriles were found to be easily prepared from respective aldehydes with PTAB-NH4OAc.
To test the application for other aldehydes by this PTAB-NH4OAc system, the reaction of various aromatic aldehydes was carried out under the same reaction conditions. The results of aromatic aldehydes are shown in Table 3. Benzaldehyde (21) was converted to benzonitrile (22) (run 1). The reaction of o-, m-, and p-tolualdehydes (23), (25), (27) took place to give corresponding nitriles (24), (26), (28) in 64-73% yields, accompanied by respective recovered aldehydes (runs 2-4). o-, and p-Nitrobenzaldehydes
(29) (31) were converted to nitriles (30), (32) (runs 5,6). o-, m-, and p-Chlorobenzaldehydes (33), (35), (37) were converted to corresponding nitriles (34), (36), (38). o-, m-, and p-Bromobenzaldehydes (39), (41), (43) were also converted to respective nitriles (40), (42), (44) in good yields (runs 7-12). Further, 2-phenylpropionaldehyde (45) and 3-phenylpropionaldehyde (47) were similarly converted to nitriles (46), (48) (runs 13, 14). Thus, the conversion of aromatic aldehydes to nitriles with PTAB-NH4OAc was not rested on the substituents of aromatic ring.
The PTAB-NH4OAc system was confirmed to be useful for conversion of heterocyclic and aromatic aldehydes to corresponding nitriles in various solvents.1
Since aromatic and heterocyclic nitriles are of particular interest as key intermediates in the syntheses of biologically active compounds by amidation and ester exchange reactions, the combination of ammonium tribromides, PTAB or PHPB and NH4OAc provides a significant alternative method for the synthesis of various nitriles from aldehydes.1,16,17
References
01. Preliminary reports were presented by S. Sayama at the 94th Spring Annual Meeting of Chemical Society of Japan, Nagoya, March, 2014 (ab., IV, p. 1580) and the 44th Congress of Heterocyclic Chemistry, Sapporo, Japan, September, 2014 (ab., p. 67).
02. (a) S.-i. Murahashi, Synthesis from Nitriles with Retention of the Cyano Group, In Science of Synthesis, Vol. 19, ed. by S.-i. Murahashi, George Thieme Verlag, Stuttgart, 2004, p. 345;; (b) S. J. Collier and P. Langer, Application of Nitriles as Reagents for Organic Synthesis with Loss of the Nitriles Functionality (Including Cycloaddition Reactions), In Science of Synthesis, Vol. 19, ed. by S.-i. Murahashi, George Thieme Verlag, Stuttgart, 2004, p. 403;; (c) Comprehensive Organic Transformation, 2nd Ed., by R. C. Larock, Wiley-VCH, New York, 1999, p. 1986.
03. (a) M. E. Fabiani, Drug News Perspect, 1999, 12, 207;; (b) M. Chichiro, H. Nagamoto, I. Takemura, K. Kitano, H. Komatsu, K. Sekiguchi, F. Tabusa, T. Mori, M. Tominaga, and Y. Yabuuchi, J. Med. Chem., 1995, 38, 353;; CrossRef (c) G. D. Diana, D. Cutcliffe, D. L. Volkots, J. P. Mallamo, T. R. Bailey, N. Vescio, R. C. Oglesby, T. J. Nitz, J. Wetzel, V. Giranda, D. C. Pevear, and F. J. Dutko, J. Med. Chem., 1993, 36, 3240;; CrossRef (d) J. B. Medwid, R. Paul, J. S. Baker, J. A. Brockman, M. T. Du, W. A. Hallet, J. W. Hanifin, R. A. Hardy, M. E. Tarrant, L. W. Torley, and S. J. Wrenn, J. Med. Chem., 1990, 33, 1230. CrossRef
04. (a) D. Tsuchiya, Y. Kawagoe, K. Moriyama, and H. Togo, Org. Lett., 2013, 15, 4194;; CrossRef (b) G.-Y. Zhang, J.-T. Yu, M.-L. Hu, and J. Cheng, J. Org. Chem., 2013, 78, 2710;; CrossRef (c) M. Hatsuda and M. Seki, Tetrahedron Lett., 2005, 46, 1849;; CrossRef (d) T. S. Ratani, S. Bachman, G. C. Fu, and J. C. Peters, J. Am. Chem. Soc., 2015, 137, 13902. CrossRef
05. (a) T. Oishi, K.Yamaguchi, and N. Mizuno, Angew. Chem. Int. Ed., 2009, 48, 6286;; CrossRef (b) S. Iida and H. Togo, Tetrahedron, 2007, 63, 8274. CrossRef
5. (c) S. Iida and H. Togo, Synlett, 2006, 2633. CrossRef
05. (d) R. R. Jadhav and K. G. Akamanchi, Chem. Lett., 2013, 42, 162. CrossRef
06. Comprehensive Organic Transformation, 2nd Ed., by R. C. Larock, Wiley-VCH, New York, 1999, p. 1658.
07. (a) H. S. Kim, S, H. Kim, and J. N. Kim, Tetrahedron Lett., 2009, 50, 1717;; CrossRef (b) M. H. Sarvari, Synthesis, 2005, 787;; CrossRef (c) B. Movassagh and S. Shokri, Synth. Commun., 2005, 35, 887;; CrossRef (d) S. H. Yang and S. Chang, Org. Lett., 2001, 3, 4209;; CrossRef (e) D. G. Desai, S. S. Swami, and G. D. Mahale, Synth. Commun., 2000, 30, 1623;; CrossRef (f) H. G. Thomas and H. D. Greyn, Synthesis, 1990, 129;; CrossRef (g) J. Streith and C. Fizet, Helv. Chim. Acta, 1976, 59, 2786;; CrossRef (h) M. J. Miller and G. M. Loudon, J. Org. Chem., 1975, 40, 126. CrossRef
08. (a) R. S. Glass and R. C. Hoy, Tetrahedron Lett., 1976, 1781;; CrossRef (b) R. F. Smith and L. E. Walker, J. Org. Chem., 1962, 27, 4372. CrossRef
09. M. M. Mojtahedi, M. S. Abaee, and T. Alishiri, Tetrahedron Lett., 2009, 50, 2322. CrossRef
10. B. Movassagh and S. Shokri, Tetrahedron Lett., 2005, 46, 6923. CrossRef
11. (a) S. Sayama and T. Onami, Synlett, 2004, 2369;; CrossRef (b) S. Sayama, Tetrahedron Lett., 2006, 47, 4001;; CrossRef (c) S. Sayama, Heterocycles, 2005, 65, 1347;; CrossRef (d) S. Sayama, Synth. Commun., 2007, 37, 3067. CrossRef
12. S. Sayama, Heterocycles, 2011, 83, 1267. CrossRef
13. W. Muramatsu, Org. Lett., 2014, 16, 4846. CrossRef
14. Y.-Z. Zhu and C. Cai, Monatsh. Chem., 2010, 141, 637. CrossRef
15. G. Bagherzade, A. Zali, and A. Shokrolahi, Chinese Chem. Lett., 2015, 26, 603. CrossRef
16. Typical procedure: To a solution of 2-pyridinecarbaldehyde 1 (54 mg, 0.5 mmol) and ammonium acetate (385 mg, 5.0 mmol) in MeCN (6 mL), was added trimethylphenylammonium tribromide (376 mg, 1.0 mmol) at room temperature. After stirring for 21 h at rt, the reaction mixture was treated with 0.5 M aq. Na2S2O3(10 mL), 1.0 M NaHCO3 (15 mL) and extracted with EtOAc (60 mL). The organic layer was washed with 0.5 M Na2S2O3 and successively washed with saturated aq. NaCl, and dried over MgSO4. After removal of solvent in vacuo, the residue was purified by column chromatography on silica gel (Wako C-200) with CCl4, CCl4-CHCl3 (2:1 v/v). 2-Pyridinenitrile 2 (49 mg, 0.47 mmol) was obtained in 95% yield. 2: IR (neat, cm-1) 3059, 3021. 2926, 2236, 1597, 1581, 1571, 1462, 1432, 1287, 1248, 1217, 1154, 1091, 1045, 992, 780, 756, 667, 631. 1H NMR (CDCl3) δ 7.53-7.58(1H, m), 7.73(1H, d, J=8.1 Hz), 7.88(1H, dd, J=8.1, 2.7 Hz), 8.75(1H, d, J=2.7 Hz). 13C NMR (CDCl3) δ 117.16, 126.89, 128.49, 133.93, 136.99, 151.08.
17. The reaction of 2-pyridinecarbaldehyde 1 (10 mmol, 1.07 g) was carried out as follows: To a solution of 2-pyridinecarbaldehyde (1.07 g) in MeCN (50 mL) were added NH4OAc (7.70 g, 0.1 mol) and PTAB (7.53 g, 20 mmol). After stirring for 18 h at rt, the precipitated trimethylphenyl- ammonium bromide (ca. 8.32 g) was filtered off, washed with MeCN (15 mL). The combined filtrate was treated with 0.5 M aq. Na2S2O3 (30 mL), 1.0 M aq. NaHCO3 (60 mL) and extracted with EtOAc (100 mL) The organic layer was washed with 0.5 M aq. Na2S2O3 (30 mL), successively saturated aq. NaCl (50 mL), and dried over MgSO4. After removal of solvent in vacuo, the residue was purified by column chromatography( φ 20 mm, L 140 mm) on silica gel (Merck, Kieselgel Type 60, 230-400 mesh) with CCl4, CCl4 and CHCl3(2:1 v/v). 2-Pyridinenitrile 2 (852 mg) was obtained.