Human Patient Simulation in Pharmacology Graduate Education: Bridging the Bench-to-Bedside Gap
The National Institutes of Health roadmap for medical research places great emphasis on translational medicine. Nonetheless, it remains a difficult proposition for PhD scientists trained to study intracellular and molecular mechanisms to appreciate the bigger picture of how their work relates to human systems biology. This difficulty is compounded by the disparate educational curricula that separate graduate students from medical students, with graduate students rarely experiencing the clinical environment. In effort to expose our PhD graduate students to integrated aspects of human physiology and contextualize drug utilization, we report the use of human patient simulation in our pharmacology curriculum.
The term “translational medicine” is often used to describe the unidirectional transition of in vitro and experimental animal research results into human applications, resulting in new drugs, devices, or novel diagnostic tools. However, translational medicine works best as a two-way street, where the incentive to “cure” is driven by a desire to improve management of human health by understanding diseases and their complexities (1). Thus, one important aspect of translational medicine is going from the bedside back into the laboratory following observations made in human disease management. For success in translational medicine, an investigator must understand not only cellular and molecular mechanisms at the laboratory level, but must also have a thorough knowledge of human biology and pathophysiology. As suggested elsewhere (2), it may be easier to bridge the bench-to-bedside gap by teaching the laboratory-trained PhD about human pathobiology than by moving a chief resident from the clinic into a laboratory.
Pharmacology has long been viewed as the most likely of the basic science disciplines to bridge the translational medicine gap. Not only does the discipline of pharmacology overlap with the basic sciences of physiology, biochemistry, immunology, cell biology, and molecular biology, but it also interfaces with the clinical sciences of neurology, psychology, microbiology and clinical medicine (3). For decades, PhD students in pharmacology learned about the discipline from a clinical perspective, with an emphasis on human biology and pathophysiology, while learning about disease alongside of medical students. However, with the recent reorganization of medical school curricula into problem-based, organ-system components, PhD students have increasingly become excluded from course participation (3). Indeed, there is a pervading belief among many faculty that graduate students should not be taught with medical students, but should receive dedicated problem-solving, literature-based instruction.
A recent survey of current pharmacology PhD programs revealed that approximately two-thirds of respondents are teaching pharmacology courses that are specially designed for graduate students, but which, unfortunately, often lack the focus on human biology and pathophysiology that is characteristic of didactic medical school courses (3, 4). In addition, many basic science departments are opting for interdisciplinary approaches that altogether fail to emphasize traditional pharmacological training techniques (3). Over the past decade, organizational structures that centralize PhD-granting programs into a core curriculum have grown, while physiology and pharmacology programs have met their demise (3, 5). Furthermore, pharmacology programs often struggle to introduce principles of the discipline into core curriculum courses that operate within interdisciplinary umbrella programs (3).
The segregation of MD and PhD students and the resulting lack of exposure of PhD students to clinical medicine and human biology is a reason for concern. The increasing focus of basic scientists on molecular approaches results in a relative neglect of the human system (2), and extrapolation of cellular findings is not always meaningful. It has become increasingly evident that the pharmacological whole is not the sum of the parts, and a workforce that lacks knowledge of pharmacokinetics, systems biology, and the unique interconnectedness of the intact body will ultimately delay the development of therapeutic advances in clinical medicine. Instructional mechanisms must be instituted and maintained in our PhD training programs to impart biomedical knowledge as a continuum from clinical medicine to research and back again (4).
In the absence of direct patient exposure and courses designed specifically for graduate students, novel teaching strategies are necessary to provide systems biology education to PhD students in the life sciences. Simulated scenarios offer one way to accomplish this task. Similar to flight simulators used by the aviation industry, human patient simulators have been proposed to enhance medical training and improve patient safety (6). During the past decade, use of patient simulators has been incorporated into instruction for all types of healthcare workers, including medical students, physicians and physician residents, nurses, and emergency personnel (7–10). However, use of simulated approaches has not been reported for graduate students in the life sciences. Here, we describe the incorporation of simulated patient scenarios and hands-on teaching demonstrations to illustrate physiological and pharmacological principles in the area of general anesthesia for graduate students enrolled in a pharmacology course at our institution.
Educational Environment
“Pharmacology 502” is a four-credit course required for second-year PhD candidates in the department of pharmacology at Pennsylvania State University College of Medicine. This course follows an integrated set of courses in biochemistry as well as cell and molecular biology (i.e., the “core curriculum” for life science graduate students at our campus). Students enrolled in other programs may also take this course as an elective. Class enrollment typically consists of three to five students per year.
The course is largely focused on teaching students about drugs that affect the nervous system and includes lectures and discussions pertaining to antipsychotics, antidepressants, anxiolytics, drugs of abuse, opioid analgesics, antiepileptic drugs, drugs that impact the sympathetic and parasympathetic systems, as well as general anesthetics. Whereas the vast majority of drug classes covered in the course have a well-defined clinical application associated with pathophysiology that can be “fixed” or “managed” with pharmacotherapeutics, the topic of general anesthetics is a bit more nebulous. When discussing anesthetic drugs, students do not have a “disease framework” in which to contextualize the drugs. As such, it is difficult in a typical didactic environment for graduate students to envision the interplay among anxiolytic drugs, skeletal muscle paralytics, opioid analgesics, and general anesthetic parenteral/gaseous agents that are combined in clinical practice to accomplish the amnesia, hypnosis, immobilization, and analgesia needed during surgery. Thus, we envisioned that a simulation session might provide a more suitable environment for conveying the pharmacology necessary to achieve a balanced anesthetic state.
Prior to the anesthesia session of the course, lectures are presented that pertain to the pharmacology of opioids, benzodiazepines, excitation-contraction coupling, and drugs that block the actions of acetylcholine at the neuromuscular junction. Then, before didactic classroom time is dedicated to general anesthetics, students are asked to review specific physiologic and pharmacologic information about these topics and make a class presentation; they are also given independent reading pertaining to general anesthesia.
In the classroom, a two-hour block of time is designated for faculty-facilitated discussion of general anesthetics. During the discussion, students are encouraged to explore differences between drugs within each of the pharmacologic classes (e.g., onset of action, duration of effect, unique properties or side effects), as well as the pharmacology of reversal agents (e.g., naloxone, flumazenil, and neostigmine). The pharmacology faculty member then provides a lecture in which the terms and concepts associated with “general anesthesia,” “balanced anesthesia”, properties of an ideal anesthetic agent, and the stages of general anesthesia are presented. In addition, current theories regarding mechanisms of action and sites of action are also covered. In this way, students are prepared to study and review the pharmacologic principles and profiles of intravenous and gaseous agents prior to entering the simulation session.
The Simulation Session
The simulation session involves a two-hour block of time in the Penn State Hershey Medical Simulation Center led by an anesthesiologist and guided by the pharmacology instructor. Our 10,000-square-foot Simulation Center comprises several learning spaces equipped with clinical patient monitors and procedural equipment. Manikin-based models, virtual-reality trainers, and partial task–training models are available, along with access to debriefing facilities. An audio-video system is available to record sessions, which aids in debriefing and enables real-time feeds to adjacent rooms for larger group training.
During the anesthesia session with the PhD graduate students, a Human Patient Simulator (HPS version 6D, Medical Education Technologies, Inc., Sarasota, FL) is attached to a Narkomed 6400 anesthesia machine (Drager, Telford, PA) and a Delta Infinity XL patient monitor (Drager, Telford, PA). The patient simulator is controlled via a wireless computer; a control rack contains pieces not housed within the actual manikin body (i.e., bellows for the lungs, the saturation generator, and the gas analyzer for determining the mixture of gas administered). In addition, separate airway intubation trainers (Laerdal Medical, Wappingers Falls, NY) are available to demonstrate intubation and airway maintenance techniques to the PhD students. The facilities and an educational session are depicted in Figure 1.
The human patient simulator is ideal for graduate students as it can simulate multiple clinical characteristics: spontaneous breathing with chest rise and fall; a realistic airway that can be instrumented with standard airway devices; breath and heart sounds that may be auscultated with standard stethoscopes; and scores of normal and abnormal heart rhythms, complete with synchronized, palpable pulses in multiple locations. Student interpretation and intervention is afforded through clinical monitors. Furthermore, the simulator possesses “eyes” that blink and respond to light. Intravenous access is present on the manikin, with attachments that allow simulated drugs to be administered via infusion sites and standard tubing.
Sophisticated computer modeling of normal and abnormal human physiology allows the simulator to represent and mimic changes in the cardiovascular and respiratory systems that occur following drug administration. Examples of simulated physiologic parameters that are altered in response to drug delivery include: uptake and distribution of respiratory gases; pharmacokinetics and pharmacodynamics of inhaled and intravenous drugs; and physiological control mechanisms such as baroreceptor reflexes and hypercarbic/hypoxic drive. These computer models respond to drug delivery, provide simulated responses, and transmit physiologic information to the clinical monitoring instruments.
To orient students to the simulator at the beginning of the session, they are provided with an opportunity to listen to heart and lung sounds, palpate peripheral pulses, observe electrical stimulation of the ulnar nerve via neuromuscular monitoring, and view pupillary responses to light. Students are also familiarized with the clinical monitor, so that they are prepared to observe changes in electrocardiogram, heart rate, blood pressure, and oxygen saturation. Finally, students are taught to intubate the human patient simulator and use the anesthesia machine to administer intravenous and gaseous agents appropriately. Once oriented to the simulator and its capabilities, students are presented with five specific clinical scenarios (Table 1) and are required to consider agents appropriate for: simple gas induction; simple mask anesthetic with muscle relaxant; short induction using an intravenous agent; medium-length anesthetic with rapid induction; and longer anesthetic with long-acting muscle relaxant.
Clinical Scenarios to Demonstrate Pharmacology Principles of General Anesthesia
For each scenario, students are engaged in decision making and active hands-on learning by choosing and administering an appropriate combination of pharmacologic agents to provide balanced anesthesia. To accomplish this, students must consider the depth of anesthesia required and the duration of the procedure that is to be performed. Students must select drugs with appropriate pharmacologic and pharmacokinetic properties for each case. In addition, students must be prepared to administer appropriate pharmacologic agents to reverse the administered drugs at the conclusion of the “procedure” or in the event of serious adverse events. Each time a decision is made to administer a drug, students are asked to describe two “good things” and two “bad things” about each drug they choose. Good things are typically described in terms of why the drug selected is especially appropriate for the specific clinical scenario (e.g., propofol for a patient who has previously suffered from nausea and vomiting during recovery), and bad things are typically described in terms of common or severe side effects (e.g., emesis with etomidate).
During the simulation session, students have an opportunity to explore therapeutic uses as well as physiologic and pharmacologic effects of general anesthetic agents and pharmacologic adjuncts. The patient-simulated scenarios allow the students to compare and contrast commonly used agents; discuss distribution, metabolism, and duration of action; and consider how pharmacokinetic properties of drugs differ with single doses versus continuous drug administration. The concepts of minimal alveolar concentration (MAC) and “second gas effect” are also discussed and their relevance correlated with anesthetic gases. Finally, the relative advantages and disadvantages of each anesthetic agent are discussed in the context of actual clinical scenarios. Educational objectives for the session are listed in Table 2.
Educational Objectives for Students at Conclusion of the Simulation Session
Student Responses
At the conclusion of each simulation session, learners complete an evaluation of the session to provide feedback to the instructors. The generation of data that resulted from these evaluations was reviewed by the Institutional Review Board (IRB) at the Penn State Hershey College of Medicine and found to be exempt from IRB oversight, as the research involves instructional techniques taking place in common educational settings (Protocol # 32482EM). Student responses were based upon a five-point Likert scale in which 1 represented an undesirable experience and 5 represented an excellent experience. Results of student s’ evaluation of the anesthesia component of Pharmacology 502 are provided in Table 3 and include simulation sessions that occurred in 2005, 2007, 2008, and 2009. Due to curriculum changes, no anesthesiology simulation session was offered in 2006. Questions 1, 2, 4, and 5 explored student perception of the quality and content of the lecture and simulation session, the explanations and responses provided throughout the session, and the extent to which the course goals were met. Question 3 evaluated effectiveness of the technology in simulating a real-life situation.
Overall, students ranked the simulation session highly, with an average of 4.76 on the five-point scale (n=17), and gave positive ratings to all parameters assessed. In 2009, three additional questions were added to the evaluation to assess student impressions of the simulation setting for learning, and all received an enthusiastic response from the five students in attendance at this session. The data indicate that students learned new information from the simulation experience and would welcome more hands-on learning opportunities in their education. A breakdown of learner comments is provided in Table 4.
Student Evaluation of Anesthesia Simulation Session
Collated and Summarized Student Comments
Conclusion
Without instructing life science trainees in a holistic manner, such that they have exposure to integrative physiology, organ systems, and the intact body (2), PhD graduate students may be hindered from seeing the “big picture;” their research at the lab bench lacks a context of medical physiology and human disease. Unfortunately, many medical school curricula have become unable to provide PhD students with opportunities to engage in active and experiential learning in pharmacology with a patient-disease focus. Certainly, patient safety and privacy concerns must limit the participation of novice students in clinical care settings. Even if graduate students were regularly able to “shadow” physicians, physician tolerance and encouragement of novice participation is variable (11). The current lack of graduate student exposure to human physiology and medical pharmacology threatens the future of pharmacological discovery and research. In an age of biomedical “translation” research, understanding the dynamics and implications of human biology and disease is a necessary prerequisite for researchers to turn innovative ideas into tangible therapeutics and diagnostics. Pharmacology is uniquely situated to bridge the bench-to-bedside gap. However, the positioning of this scientific discipline also presents unique challenges when assembling curricula, especially during recent years, as translational research has become a recurring theme and universities struggle to strengthen the relevance of graduate student research to clinical medicine (12).
Here, we have presented the application of simulated and hands-on patient-based learning scenarios for PhD graduate students in the life sciences. We believe that the use of human patient simulators conveys physiologic and pharmacologic concepts to our pharmacology graduate students, providing an ideal mechanism for approaching the challenges of translational research. Based upon overwhelmingly positive student feedback, the simulated participatory anesthetic session appears to empower students to integrate and apply their knowledge of drugs, in a non-threatening, translational environment, that recapitulates “real-life” patient-centered scenarios. We believe that active, hands-on student engagement, as opposed to two-dimensional diagrams, has enhanced student knowledge and confidence in understanding physiology and drug action.
The topics we discuss in the classroom and the simulation session are in agreement with knowledge objectives that have been outlined by the American Medical School Pharmacology Chairs (www.amspc.org/Knowledge_Objectives_2008/index.htm). Students are informed in advance that the success and satisfaction they will experience in the simulation center is largely dependent upon their prior knowledge. This provides them with motivation to prepare and learn for a reason other than an end-of-course examination. Students are aware that simulation sessions will demonstrate the gaps in their knowledge, thereby facilitating expansion of their knowledge in a risk-free “real life” context.
There are several positive features of the simulation experience provided to our graduate students. The simulations reinforce book-learned concepts and clearly illustrate to students knowledge areas they must acquire prior to the final course examination (11). In most cases, hands-on experience promotes learning to an extent not achievable by classroom lectures and reading assignments alone. In addition, simulation-enhanced learning allows the training agenda to be modified for the needs of each learner; students can learn from their mistakes in a small-group environment would be unfathomable and unsafe in actual clinical scenarios. Active learning, which tends to be preferred over passive learning by adult learners (13), is the basis of the simulation experience. Furthermore, our approach makes use of a variety of teaching methods (e.g., readings, lectures, student presentations, and simulation session); the utilization of multiple teaching methods fosters knowledge (14). Finally, we found the simulated sessions to promote an interactive mode of instruction in physiology and pharmacology without the cost and ethical concerns associated with using humans or live animals in research (7).
Admittedly, the costs involved with running the simulation session are not trivial. Two professors (an anesthesiologist and a basic scientist) and a technician are utilized at the simulation session; collaboration between departments has thus been critical to successful incorporation of simulation into our curriculum. The simulation session is technologically demanding, requiring a complex human patient simulator and personnel trained to effectively operate the computer. Nonetheless, with more than one-third of all medical schools having access to human patient simulators, we believe that integration of simulation into graduate education could be feasible at many institutions (7). With the ever increasing need for PhDs to think as translational scientists, pharmacology faculty must be mindful of opportunities to integrate human patient simulators into graduate student education. Although the feedback and responses from students have been overwhelmingly positive to the simulation experience, we are limited by lack of a comparator group to truly assess the impact that the hands-on session provides. There are no students in our curriculum who receive only a didactic lecture on the concepts of general anesthesia.
As a result of our initial success in simulation, hands-on training sessions have extended to other areas of graduate education curricula. One includes a simulated “patient” session that focuses on scenarios that reinforce concepts in cardiovascular physiology and pharmacology. In another simulation, students are provided with the opportunity to experience “asthma” through use of placebo metered-dose inhalers, dry powder inhaler demonstrators, and peak flow meters. Additionally, students are given the chance to practice “a day in the life of a diabetic” through utilization of a glucose meter to test their blood sugar (e.g., before and after eating a donut). Many students have indicated that they learn more by “doing” in the simulation experience than they would through reading a text and attending a traditional lecture. We have found patient simulation and hands-on demonstrations to be valuable components of our pharmacology graduate educational programs, and we continue to explore ways to incorporate additional simulated sessions within our curriculum.
- Copyright © 2010
References
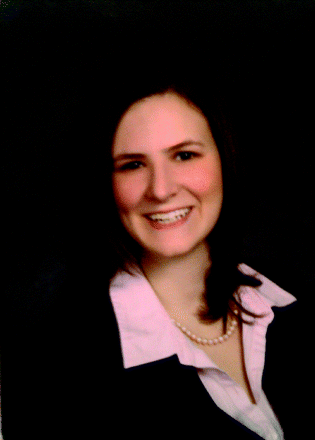
Melissa Fritz (left) and Maura Schwab are recent graduates of Duquesne University. Both women chose to complete pharmacy rotations with Dr Karpa owing to their interest in academia and non-traditional instructional methods. They assisted Dr Karpa with this simulation endeavor.
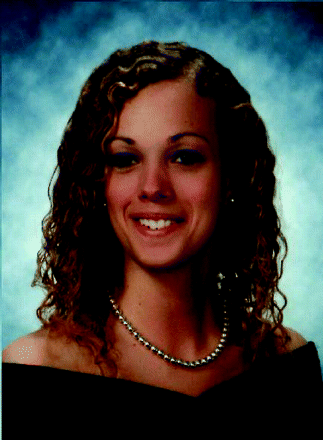
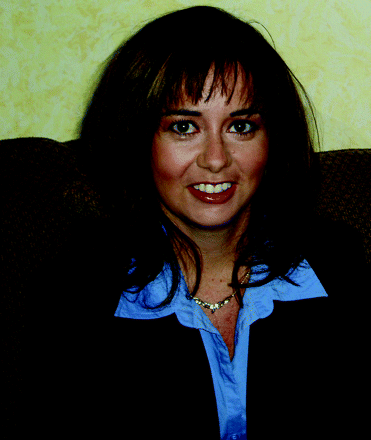
Kelly Karpa, PhD, RPh, is director of medical pharmacology instruction at Penn State University College of Medicine. She brings hands-on learning experiences into her classrooms whenever possible. Send correspondence to KDK. E-mail kjd136{at}psu.edu; fax 717-533-2006.
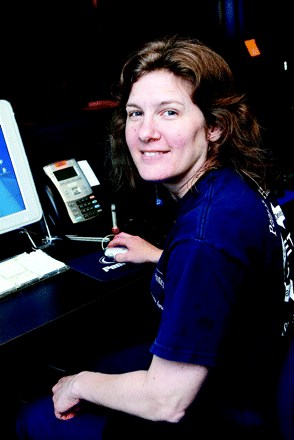
Jody Wood, BS, is a coordinator for the Simulation Center. Among her many roles, she maintains and repairs the equipment, assists with curriculum development, and maintains the informational management system for data collection and report creation.
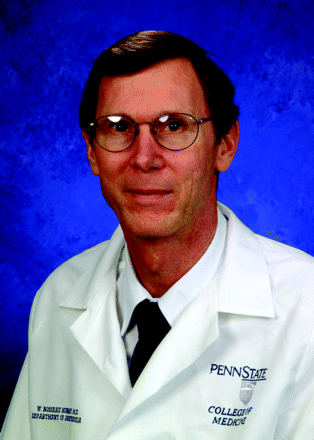
W Bosseau Murray, MD, has been developing innovative teaching sessions and curricula in the Simulation Center at Penn State University for almost two decades. His emphasis is in multi-disciplinary and large group simulation sessions using a wide variety of simulators.