Determining the Destiny of NF-κ B after TCR Ligation: It’s CARMA1
The nuclear factor-κ B (NF-κ B) family of transcription factors consists of RelA (p65), RelB, and c-Rel, which possess transactivating domains, and NF-κ B1 (p50) and NF-κ B2 (p52), which do not [reviewed in (1, 2) ]. Canonically, transcriptionally competent NF-κ B dimers are retained in the cytoplasm by the inhibitor of NF-κ B (Iκ B). In response to external stimuli, Iκ B is first phosphorylated by an Iκ B-kinase (IKK) and then targeted for proteasomal degradation by a ubiquitin-ligase. The degradation of Iκ B releases functional NF-κ B dimers that translocate to the nucleus, where they bind to response elements in the promoters of dozens of genes, including those of cytokines, chemokines, adhesion molecules, anti-apoptotic factors, and regulators of proliferation (3) . The anticipated role of NF-κ B in the generation of adaptive immune responses has been confirmed in multiple genetic models (4) . In T lymphocytes, NF-κ B is most strikingly required for proliferation and the production of cytokines in response to T cell activation (5, 6) .
Recently, several proteins linking the T cell receptor (TCR) to NF-κ B activation have been identified. Much excitement has focused on protein kinase C ?? (PKC?? ), a “novel” PKC isoform primarily expressed in T lymphocytes and uniquely recruited to the “immune synapses,” which form during T cell activation in vivo (7, 8) . Genetic disruption of the PKC?? gene dramatically impairs TCR-induced proliferation and NF-κ B activation without perturbing nuclear factor of activated T lymphocytes (NF-AT) activation (9) . Strikingly, the activation of NF-κ B in the absence of PKC?? is specifically impaired in response to TCR ligation, but it is normal in response to TNFα treatment. Subsequent studies established that the activation of PKC?? and NF-κ B by the TCR and the costimulatory molecule CD28 requires receptor-proximal proteins including the tyrosine kinase ZAP-70 and the adaptor SLP-76 (10) . PKC?? activation is also contingent on its translocation to lipid rafts in the plasma membrane, in response to receptor ligation. The redistribution of PKC?? depends on the activity of the Src-family kinase Lck, phosphatidylinositol 3’-kinase (PI3K), and the guanosine triphosphatase (GTPase) exchange factor Vav (11–14) . Although these observations clearly link PKC?? activation to events driven by the TCR and CD28, the molecular interactions that localize and activate PKC?? have not been precisely defined (15, 16) .
Three recent reports demonstrate that CARMA1 [CA spase R ecruitment Domain-containing MA GUK (membrane-associated guanylate kinase) protein-1] bridges the gap between PKC?? and the IKK-dependent activation of NF-κ B in T cells. Wang et al. have shown that a T cell line lacking CARMA1 is unable to activate NF-κ B in response to either the coligation of TCR and CD28 or the overexpression of a constitutively active PKC?? (17) . CARMA1-deficiency also results in the inability to activate IKK, degrade Iκ B, or produce interleukin-2 (IL-2) in response to receptor engagement. In complementary experiments, both Gaide et al. and Pomerantz et al. used a dominant-negative mutant of CARMA1 to demonstrate that wild-type CARMA1 is required for the activation NF-κ B in response to TCR crosslinking (18, 19) . In all three cases, NF-κ B activation in response to TNFα was normal, as was observed in PKC?? -deficient T cells. These data establish CARMA1 as a critical linker specifically joining the TCR and PKC?? to NF-κ B activation through the IKKs.
CARMA1 coprecipitates with the TCR, colocalizes with capped TCR, and is recruited to lipid rafts enriched in PKC?? (18) . These interactions are most likely mediated by the C-terminal MAGUK (membrane-associated guanylate kinase) homology region in CARMA1. This region, defined by the presence of a PDZ (PSD-95/Dlg/ZO-1 homology) domain, a SH3 (Src homology 3) domain, and a GUK (guanylate-kinase homology) domain, is commonly involved in both grouping transmembrane proteins into functional complexes and in tethering membrane proteins to the cytoskeleton (20) . Therefore, it will be essential to define the interactions that link CARMA1 to TCR-proximal signaling complexes and to PKC?? . Direct interactions between MAGUK proteins and receptor tyrosine kinases are not unprecedented, and PDZ-containing proteins might govern the localization of critical signaling proteins in T cells (21, 22) . Thus, CARMA1 may prove to be an integral component of the “immune synapse” with a prominent role in facilitating interactions between PKC?? and its substrates.
Figure 1. A Model of TCR/CD28-Induced NF-κB Regulation.
The coligation of the TCR with CD28 results in the activation of tyrosine kinases and the recruitment of lipid rafts to the aggregated TCR. Several receptor proximal tyrosine kinases, adaptors, and effectors, including Lck, ZAP-70, SLP-76, and Vav, have been implicated in the regulation of NF-κB and the recruitment of PKC?? to lipid rafts. CARMA1, specifically required for the activation of NF-κB by the TCR and PKC??<links raft-associated receptor complexes to Bcl-10 through the heterodimerization of the CARMA1 and Bcl-10 CARD domains. CARMA1 membrane association is most likely driven by the MAGUK homology region, which consists of a PDZ domain, a SH3 domain, and a guanylate kinase (GUK) homology domain. The recruitment of Bcl-10 results in its phosphorylation, with unknown consequences. Bcl-10 directs the multimerization of the human paracaspase MALT1, which interacts with Bcl-10 through its immunoglobulin-like (Ig) domains. Multimerization of the MALT1 paracaspase domain is sufficient to activate NF-κB by unknown mechanisms. Ultimately, NF-κB activation is triggered by the induced phosphorylation, ubiquitinylation, and proteasomal degradation of IκB. The canonical IκB kinase (IKK) complex—consisting of the kinases IKKα and IKKβ, and the linker IKKγ—is required for the initiation of this degradative process. Preliminary evidence suggests that additional IκB kinases (TBK1, IKKε) and scaffolds (TANK) participate in the degradation of IκB in response to TCR ligation or phorbol ester and calcium ionophore treatment. TCR, T cell receptor; ZAP-70, TCRζ-associated protein of 70-kD; IKK, inhibitor-of-κB kinase; SLP-76, SH2 domain-containing leukocyte protein of 76-kD; CARMA1, caspase recruitment domain-containing MAGUK protein-1; MALT1; mucosa-associated lymphoid tissue of B lymphoma 1; nuclear factor-κB, NF-κB; TANK, tumor necrosis factor receptor-associated factor (TRAF) family member-associated NF-κB activator; TBK1, TANK-binding kinase 1.
Two likely effectors of CARMA-1-dependent NF-κ B activation were first identified as proteins affected by translocations commonly found in MALT lymphomas (B cell lymphomas of mucosa-associated tissue). The first of these proteins, Bcl-10, contains a caspase recruitment domain (CARD) that specifically dimerizes with the CARD domains of CARMA family proteins (23-25) . Bcl-10 is essential for TCR- and CD28-induced NF-κ B activation, IL-2 production, and T cell proliferation, but is not required for TCR-induced tyrosine phosphorylation, increases in intracellular calcium concentrations, or extracellular-signal-regulated kinase (ERK) activation (26) . CARD-deleted variants of CARMA1 are incapable of binding Bcl-10 and are potent inhibitors of NF-κ B activation in T cells, suggesting that Bcl-10 is a critical effector of CARMA1 (18, 19) . Consistent with this hypothesis, Bcl-10 augments the NF-κ B activation induced by either PKC?? or CARMA1, colocalizes with CARMA1 and the TCR, and is recruited to lipid rafts in a CARMA1-dependent manner (17, 18) . The second protein associated with NF-κ B activation in MALT lymphomas is a caspase-related protein known as human paracaspase or MALT1 (27) . MALT1 interacts directly with the C-terminal domain of Bcl-10 through two immunoglobulin (Ig) domains and activates NF-κ B in a manner dependent on both its Bcl-10 interaction domain and its paracaspase domain. Although the role of MALT1 in T cells has not been clearly established, oligomers of the MALT1 paracaspase domain were able to bypass the inhibitory effects of a dominant-negative variant of a CARMA family member (expressed in nonlymphoid cells), suggesting that MALT1 functions downstream of Bcl-10 in T cells (25) .
CARMA proteins, Bcl-10, and MALT1 clearly effect changes in NF-κ B activity through the components of the canonical IKK complex, IKKα , IKKβ , and IKKγ (24, 25) . However, it is unclear whether CARMA–Bcl-10–MALT1 complexes facilitate IKK activation by providing a scaffold for their recruitment and/or oligomerization in lipid rafts, or by a more indirect mechanism involving additional intermediates (12) . Significantly, the reconstitution of phorbol ester and calcium ionophore-induced NF-κ B activation in IKKγ -deficient T cells requires a domain of IKKγ implicated in interactions with the adaptor TANK [TNF receptor-associated factor (TRAF)-associated NF-κ B activator] and two noncanonical IKKs, IKKε and TBK1 (TANK-binding kinase 1) (1, 28–30) . TRAF family members are also of interest, because several of these proteins interact with both Bcl-10 and TANK, and could link CARMA–Bcl-10–MALT1 complexes with IKK-containing complexes (31, 32) . Although a dominant-negative TBK1 failed to suppress CARMA1-dependent NF-κ B activation, further consideration of TRAFs, TANK, and IKKε as effectors of NF-κ B activation downstream of the TCR and CARMA1 is warranted (19) . Because the constitutive activation of NF-κ B results in lymphoid disorders, the capacity of CARMA1 to activate NF-κ B must be carefully regulated in vivo (4) . Downstream of CARMA1, the recruitment of Bcl-10 to the plasma membrane, the oligomerization of Bcl-10, and the oligomerization of MALT1 all promote the activation of NF-κ B (25, 33, 34) . Thus, CARMA1 may function by recruiting Bcl-10-MALT1 complexes to the plasma membrane and directing the oligomerization of these proteins. As described above, the interaction of CARMA1 with the TCR and associated membranes is likely to be mediated by the MAGUK homology region. The N-terminal region of CARMA1 containing the coiled-coil and CARD domains is sufficient to potently activate NF-κ B, and the coiled-coil and CARD domains are each required for the TCR-induced activation of NF-κ B (19, 23-25) . These observations suggest that the coiled-coil domain oligomerizes CARMA1 and that the Bcl-10-MALT1 complexes associated with the CARD domain of CARMA1. In this model, the oligomerizing activity of CARMA1 must be regulated in order to prevent the constitutive activation of NF-κ B. Because deletions that remove the MAGUK homology domain of CARMA1 dramatically increase the activation of NF-κ B, the coiled-coil and CARD domains of CARMA1 might be sequestered by intramolecular interactions with the MAGUK homology region (in the wild-type protein) in resting cells (23–25) . In this regard, it is interesting to note that activated PKC?? is sufficient to direct the recruitment of the canonical IKK complex (composed of IKKα , IKKβ , and IKKγ ) into lipid rafts (12) . Because CARMA1 is a critical mediator of signals from PKC?? to the IKK complex, PKC?? could regulate the localization of the IKK complex by modulating the conformation of CARMA1. In future studies, it will be crucial to determine if CARMA1 is phosphorylated by PKC?? .
Pharmacologic agents specific for the NF-κ B-activating pathways unique to lymphoid cells have significant potential as immunosuppressive compounds and as therapeutic agents for the treatment of lymphomas dependent on the proliferative and anti-apoptotic effects of NF-κ B. Potential exists for the treatment of chronic lymphocytic leukemia (CLL), adult T-cell leukemia (ATL), activated B cell-like diffuse large B-cell lymphoma (DLBCL), and multiple myeloma (MM) (35–37) . As a lymphoid specific protein, CARMA1 presents a promising target for the development of tissue specific NF-κ B inhibitors (23) . However, neither CARMA1 nor its downstream effector Bcl-10 posses catalytic activities toward which specific inhibitors could be directed. Although the concept has not yet been validated in vivo, it may be possible to develop agents that specifically inhibit the protein–protein interaction domains in the MAGUK homology region of CARMA1. In particular, the SH3 and GUK domains of CARMA1 have been validated as potential targets for drug development, whereas the PDZ domain is not required for the activation of NF-κ B by CARMA1 (19) . Alternately, it may be possible to develop inhibitors of kinases that specifically participate in the CARMA1 signaling pathway. The relatively limited tissue distribution of PKC?? and the critical role of PKC?? in TCR-induced NF-κ B activation recommend PKC?? as a target for drug development. Downstream of PKC?? , the degree to which Bcl-10 is phosphorylated corresponds proportionally to the degree of NF-κ B activation; if this phosphorylation of Bcl-10 is critical to NF-κ B activation, the physiological kinase of Bcl-10 may also prove an attractive target for drug development. However, caution will be required with agents affecting Bcl-10, as approximately one-third of bcl-10-/- embryos exhibit lethal defects in neural tube closure (26) . Finally, IKKε is expressed most highly in thymus, spleen, and peripheral blood lymphocytes (28) . Thus, if IKKε proves to play an essential role in TCR-mediated NF-κ B activation, this kinase could also serve as valuable target for molecular intervention.
- © American Society for Pharmacology and Experimental Theraputics 2002
References
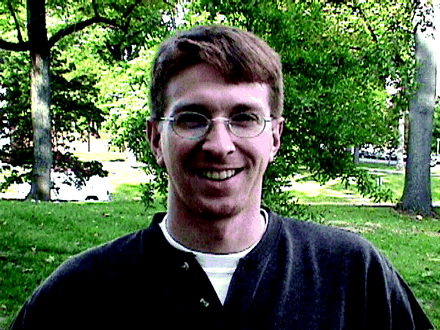
Stephen C. Bunnell, PhD, is currently a postdoctoral fellow with Larry Samelson at the Laboratory of Cellular and Molecular Biology at the National Cancer Institute of the National Institutes of Health. He has a long-standing interest in signal transduction in the immune system. His current work focuses on the dynamic visualization of TCR ligation-induced molecular interactions in live T cells. Address correspondence to SCB at sbunnell{at}helix.nih.gov