Richard Weinshilboum
Pharmacogenetics: The Future is Here!
Richard Weinshilboum has a no-nonsense attitude about pharmacogenetics. He is enthusiastic about the practicalities and ramifications of the field’s solid accomplishments, but he carefully measures statements that might feed the hype that is en courant about the brave new postgenomic world of drug therapy. Although the terms “pharmacogenomics” and “pharmacogenetics” are often used interchangeably (a linguistic quirk to which Weinshilboum does not object), he consistently avoids the latter, perhaps more glitzy, word. Weinshilboum has spent over thirty years as a clinical pharmacologist, exploring in particular the variability of drug metabolism that occurs among patients as a function of their genetic constitution. The research efforts from his line of work have materialized into clinical application and have helped to set the stage for the individualization of drug treatment according to each patient’s genetic constitution—not yet on the genomewide scale that Weinshilboum enthusiastically foresees, but certainly as pertains to multiple genes and drugs for any given patient. The interview with Weinshilboum occurred at this year’s annual meeting of ASPET, at which he was conferred the Harry Gold Award in Clinical Pharmacology.
MI: When you started your research career in 1964, was the term “pharmacogenetics” already known?
RW: Yes. Pharmacogenetics was a term that had been coined by Arno Motulsky in the 1950s. The first examples of pharmacogenetics related to drug metabolism and came, in part, from the work of Werner Kalow and his colleagues in the metabolism of succinylcholine, a muscle relaxant used for short-acting anesthetic procedures. Some patients had an inherited relative deficiency of the so-called butyrylcholinesterase (or “pseudocholinesterase”) activity, so that they would have prolonged paralysis (including apnea) after receiving succinylcholine. This variability in patient response helped lead to the concept of pharmacogenetics, that is, that inheritance plays a role in interindividual variation in drug efficacy and drug toxicity. There were a group of individuals who were pioneers in this area, people like Werner Kalow, Bert La Du, and Wendell Weber. In terms of drug metabolism, the two historical examples that are used most often are the butyrylcholinesterase example and the N -acetyltransferase 2 polymorphism. In the early days we didn’t realize that it was N -acetyltransferase “2” because we didn’t know there were two genes. We need to be quite clear that, particularly for the N -acetyltransferase polymorphisms, these were common polymorphisms. In those days we weren’t working at the level of DNA. We were looking at traits; we were examining phenotypes. For example, a drug—perhaps isoniazid—would be administered at a given dose to a group of patients, and after analysis of plasma levels of the drug, the population could be divided into distinct groups. Characteristic rates of drug metabolism became recognized as a heritable trait, verifiable by the techniques that Gregor Mendel developed, that is, segregation analysis. In other words, we were studying common polymorphisms.
MI: When you say “common” polymorphisms, what does that mean?
RW: Take, for example, the catechol-O -methyltransferase genetic polymorphism that helps determine the metabolism of drugs such as l -dopa, a drug that’s used to treat Parkinson’s disease, and methyldopa, an older antihypertensive. The allele frequencies in Caucasian populations are such that about twenty-five percent of individuals are homozygous for the trait of low enzyme activity, twenty-five percent are homozygous for the trait of high activity, and fifty percent are heterozygous and show intermediate activity—as balanced a polymorphism as you can find.
More generally, the definition of “polymorphism” from a population genetics point of view corresponds to an allele frequency of one percent or greater in the population, which is too common to be the result of random genetic mutation. In the old days—the Paleolithic era when I began my career—we clearly weren’t able to correlate such common genetic traits with changes in DNA sequence. Of course, with the ongoing transformation of pharmacogenetics based on biochemical characterization to its modern basis in genomics, we increasingly have the ability to begin with the phenotype, identify the responsible protein—drug-metabolizing enzymes have been predominant, but drug transporters and drug targets are increasingly studied—and clone the gene so we can understand at a molecular level why we see a given trait. With completion of the Human Genome Project, we can often identify multiple polymorphisms for many genes. In the early 90s, for example, it was thought that there were perhaps three cytosolic sulfotransferase enzymes and, thus, three genes in humans. As a result of a great deal of work from my laboratory and many others’, we knew of ten sulfotransferase genes by the end of the decade. Completion of the Human Genome Project indicates that there might be even more, so that we’re now in a position, without even knowing what some of these enzymes do, to determine common genetic variations at the level of DNA. I think that’s going to be a more common paradigm, certainly in the transition period we’re in presently, than to begin with the phenotype as we did years ago.
One wonderful thing about pharmacogenetics is that it is an excellent example of a field that began at the bedside and went to the bench. We began with clinical observations of patients who could not breathe even after they woke up from anesthesia, or patients who developed profound myelosuppression when treated with “standard” doses of thiopurine drugs. Those phenotypes at the bedside led to the biochemical and, ultimately, genetic and molecular studies that enabled us to understand the underlying mechanisms of those adverse drug effects.
MI: Did you, as a young person, come from a scientific or medically trained family?
RW: My father ran a hardware store in Kansas. I was the first person from my family—from both my paternal and maternal families—who completed a college education. My favorite story with regard to the confusion that some of my family members felt about what I was doing was when I was in my fifties and I was home for a Thanksgiving dinner that included my father’s twin brother, who also ran a hardware store. By that time I was a department chair and a full professor, but at the Thanksgiving dinner table my uncle asked me, “Richard, you’re still in the lab, is that right?” And I said, “That’s right.” Whereupon he asked, “Well when do you graduate, anyway?” So there was some bafflement about what this career was all about.
MI: When you graduated from medical school, did you foresee your career in terms of a movement from the bedside to the laboratory bench?
RW: I knew that I would do research. This was during the Vietnam War. The vast majority of my colleagues who were interested in research found themselves at the National Institutes of Health [in order to fulfill the wartime obligation for national service]. I was exceedingly fortunate in that I was a PRAT Research Fellow in Julius Axelrod’s laboratory. I was in his laboratory when he received the Nobel Prize.
MI: That must have been encouraging!
RW: It certainly was. My experience at the NIH convinced me that medical research was what I wanted to do with my life.
MI: Julius Axelrod mentored a large number of successful pharmacologists. What is it about him that fostered such success in his trainees?
RW: When Julie won the Nobel Prize, Sol Snyder sent him a telegram, saying that they should give him a second Nobel Prize for his accomplishments as a mentor of young scientists. The man is inspiring. I don’t know whether you’ve seen the book Apprentice to Genius , written by Robert Kanigel, a science writer who was at Johns Hopkins [now at MIT]. It’s about the mentorship chain from Shannon to Brodie to Axelrod to Sol Snyder to Candace Pert. Mentorship is a very delicate relationship, probably second only to the parent-child relationship. It can be wonderful, but it can also go very wrong. It is a big responsibility.
MI: Has your role as mentor been an important aspect of your career?
RW: Frankly, when you reach my age, you’ve got to ask, “What’s really important?” Two things were really important in my career. First, science is just fun, and the other important aspect of my scientific life has been the opportunity to work with young people. The energy, enthusiasm, irreverence, total lack of worrying about all that other stuff, which the young people bring, is really what it’s all about. I take great pride in their accomplishments, in what they’ve gone on to do in diverse ways: everything from being full professors and department chairs to working at the FDA, to being involved in education. It has been the greatest privilege of my life to work with these young people from all over the world. Come on, how brave do you have to be to leave your country and come to a different culture! These kids basically rip themselves up by the roots and put themselves in a different culture. Why? Because of the excitement of pursuing their profession, because they want opportunity! So, of course being a mentor is important to me. The responsibilities of being a mentor are analogous to the responsibilities of being a parent. They never end. They go on and on and on forever. And that’s fine.
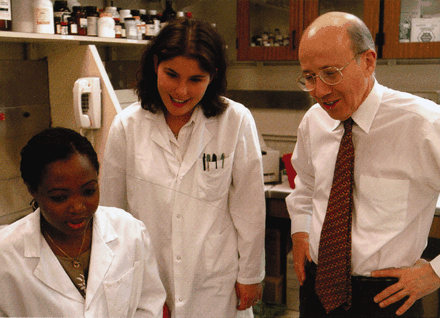
Dr. Weinshilboum discusses lab work with laboratory technologist Bianca Thomae and research associate Dr. Araba Adjei.
MI: What message do you as a mentor have for newcomers to the discipline of pharmacology?
RW: First I tell prospective students, “We need you!” Pharmacology has always had a problem because it hasn’t had visibility in the undergraduate curriculum, especially in comparison to biochemistry and physiology, which have long been part of the undergraduate curriculum. Interestingly, as a result of the genomic revolution, we’re seeing an increase in the numbers of students who find our science exciting, and they’re becoming increasingly interested in our discipline.
MI: You spoke about observations from the clinic inspiring your research at the bench. How are the research findings of pharmacogenetics now being brought back to patients in the clinic?
RW: Ultimately, the work is leading to the individualization of therapy based on an understanding of the genetic basis for variation in patient responses to drugs. For example, the Food and Drug Administration is currently deciding whether they will recommend individual genetic testing for thiopurine methyltransferase activity with the labeling of the thiopurine drugs. Pharmacogenetic testing would help make it possible to give the individual patient the dose that is appropriate based on his or her genetic ability to metabolize a thiopurine drug. There have been tragic deaths when patients, treated with standard doses of these drugs, prove to be genetically unable to metabolize them appropriately.
MI: Haven’t some people argued that pharmacogenetic data, rather than leading to individualized therapies, will instead spur drug companies to search for compounds unaffected by those drug-metabolizing enzymes that are prone to interpatient variability?
RW: It’s my understanding that drug companies are understandably concerned about drugs that are metabolized, for instance, by cytochrome P4502D6 [CYP2D6]. Ultimately, however, there will be recognition of—and pharmaceutical interest in—common genetic variation at the level of molecular drug targets, and although one might in theory be able to avoid interpatient variability in terms of drug metabolism and transport, such circumvention may not be practical for the drug target. In the long run, given that the targets will also be genetically variable, you clearly will have to deal with the glorious genetic diversity of our species. Patient populations will inevitably be stratified in terms of their metabolism of drugs as well as in their ability to respond as a function of target variation. Although the field of pharmacogenetics/pharmacogenomics has evolved out of variations in drug metabolism, the same degree of common variation is being found in drug transporters and in drug targets. So, frankly, there will probably be little way for the pharmaceutical/biotechnology industry to totally escape the variation that makes our species so wonderful.
MI: So pharmacogenomics as a field is here to stay?
RW: Absolutely—to the same extent that some of us are tall, some of us are short, and some of us are bald, there is also genetic variation in drug response. But above all, I want to be quite careful to emphasize that, regardless of pharmaceutical or biotech interests, pharmacogenetics is a research area that is driven fundamentally by science. Sure, it happens to be a field of science that deals with the diversity of our species, but ultimately we are driven scientifically by mechanistic questions. One that fascinates me is the mechanism by which human cells, as well as the cells of other mammals, recognize single changes in amino acid sequences resulting from genetic variation and often rapidly degrade these subtly altered proteins. What is the recognition system? What role do chaperone proteins play and how is protein folding involved? What about ubiquitination? Which E3 ligase is involved? What is the role of the proteasome in this process? Clinical pharmacology—and pharmacogenetics as a subdiscipline—is fundamentally a scientific field like any other, unique only in the nature of the specific questions addressed. The questions we ask have taken us from bedside to bench and back again to bedside, but this movement has been driven by science—not by the their implications for the FDA or the pharmaceutical industry.
MI: Do you have any particular view as to how pharmacogenetics—however basic a science it is—will influence drug development in the future?
RW: Drug development?—you’re asking the wrong person. I mean, what’s my history? I went to a state university medical school; next I went to Harvard teaching hospital; then I went to the NIH; and I spent the past thirty years at an academic medical center. I have a disclaimer that I use when I talk about what pharmacogenomics means in drug development. I say that I’ve never participated in the development of a drug in my life! I can tell you what it will mean at the level of the use of the drug, but not in terms of drug development. However, it’s clear, particularly at the level of the pharmaceutical industry, that the term “pharmacogenomics” has a multitude of definitions. In some settings, it’s being used as a term to apply to the use of genomic information to identify drug targets. It is also used within the pharmaceutical industry to talk about the individualization of therapy and why we see a range of variation in both therapeutic efficacy and toxicity during drug development. In this latter sense, testing for genetic variation, both in drug metabolism and in drug targets, is being incorporated into the overall process of drug development.
MI: Leaving the problem of drug development to the side, how close are we to routinely genotyping people, so that when someone goes into the hospital, the physician can offer individualized therapies?
RW: It’s already available, on a limited scale. In academic referral centers, that type of information is being increasingly incorporated into clinical practice. At our place (Mayo Clinic), we determine the thiopurine methyltransferase phenotype of about five thousand patients per year. We also have available for the clinical staff a DNA-chip based test for CYP2D6, CYP2C9, and CYP2C19. Our clinicians are increasingly asking for that information. In psychiatry, where many of the drugs are metabolized by CYP2D6, our psychiatrists have begun to request that type of genotypic information. In the vascular clinics, where warfarin is metabolized by CYP2C9, the physicians are increasingly depending on pharmacogenetic information. It will not become universally practiced overnight, but it is there already. The future is here!
MI: Are genomics and bioinformatics being incorporated into the training of physicians?
RW: At Mayo, we have a very large genomics education program, which is directed by a former Chair of our Department of Biochemistry and Molecular Biology and is aimed at a clinical staff of 1400 and about 1200 residents in training. This genomic training is individualized with regard to specialties—what a urologic surgeon will need to know about genomics is different from what an endocrinologist will need to know. The program is supported mainly by development dollars, that is, by grateful patients’ donations, and is made available for the benefit of the patient in both diagnostics and therapeutics. I think that’s happening increasingly in major academic centers.
MI: Will the availability of new genomic data place new responsibilities—and liabilities—amid the activities of health-care providers?
RW: This kind of information should only enter clinical practice when it can be validated in the same way that any other information, like drug efficacy, that relates to patient response is validated. We’re talking about evidence-based medicine. But once we have this information, we should be obligated to use it for the benefit of patients. I personally believe that there is no excuse for the patient who is homozygous for the “*3A” variant of thiopurine methyltransferase [TPMT*3A] (that is, four to five percent of all TPMT alleles in Caucasians) to be endangered by being treated with standard doses of thiopurines. Speaking as a physician, we are responsible for using the scientific knowledge that pharmacogenetics has given us. We need to appreciate the common genetic polymorphisms within genes that encode drug-metabolizing enzymes, drug transporters, and drug targets. We then need to know which of them are of functional importance— and what are the underlying mechanisms for those functional implications? This is one of those situations in which it’s like a ping-pong match: You go back and forth and back and forth between bedside and bench observations. My worry is that we will lose sight of the fact that there is a tremendous amount of science that still needs to be done.
MI: You’ve stressed the science of pharmacogenetics, but aren’t there societal ramifications to the genotyping of humans that we should be aware of?
RW: I am a scientist, and the science of pharmacogenetics needs to be, to the extent that it’s possible, absolutely objective. We should not enthusiastically encourage the incorporation of testing that is of no clinical value. But that doesn’t mean that we can ignore the social, political, and economic implications—it’s just that we first need to understand the science very well.
From that point, then, it becomes a thicket of social, political, and economic issues that have to be dealt with. Frankly, I have little patience with the concern that, “Oh, gee, pharmacogenetic testing of patients is going to add to health care costs.” With that type of mindset, we never would have developed the Salk vaccine. I think it’s up to the scientific establishment and the academic medical centers and the NIH to take a leadership role to develop a scientific base that will promote translational biomedical research. We cannot just sit back and allow the chronic illnesses that are going to become increasingly prevalent as our population ages to overwhelm the gross domestic product. We must accept the challenge of trying to understand diseases, prevent them, and treat them in a way that is cost-effective and efficacious. Every new therapy that has ever come along has been relatively costly at its inception as compared to its eventual routine use.
We also have to deal with the issue of confidentiality of DNA-based information. If patients don’t think that this genetic information, which we will be increasingly gathering on them, is treated confidentially and will be used to their advantage, then the benefits of this science will not be allowed to flow to society at large. The societal issues are inseparable from the scientific advances. But by all means, let’s remember that pharmacogenetic developments are based on the scientific method and that we apply these developments as dictated by scientific principles. It just so happens that in clinical pharmacology we study Homo sapiens . It is my firm belief that the first place that the genomic revolution will have implications of broad practical importance for medicine is in therapeutics—therefore, pharmacogenomics.
- © American Society for Pharmacology and Experimental Theraputics 2003