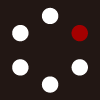
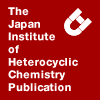
HETEROCYCLES
An International Journal for Reviews and Communications in Heterocyclic ChemistryWeb Edition ISSN: 1881-0942
Published online by The Japan Institute of Heterocyclic Chemistry
e-Journal
Full Text HTML
Received, 11th November, 2008, Accepted, 9th January, 2009, Published online, 15th January, 2009.
DOI: 10.3987/COM-08-11596
■ Copper-Mediated N-Arylation in the Synthesis of Aryladenines
Jan Krouželka and Igor Linhart*
Department of Organic Chemistry, Institute of Chemical Technology Prague, 166 28 Prague, Technická 1905, Czech Republic
Abstract
A series of N3 and N9 aryladenines was prepared by arylation of 8-bromoadenine under modified Chan-Lam-Evans coupling conditions followed by reductive debromination with hydrogen on palladium. Conditions of arylation were optimised to maximise the yield of N3-arylated products. The selectivity of the reaction varied with temperature the ligand used. The best results were obtained in DMF with phenanthroline as a ligand at 60°C.INTRODUCTION
Substituted purines attracted much interest as therapeutics, molecular tools and probes for investigating biological systems.1 Due to their similarity with adenosine, many 9-substituted adenines interact with adenosine receptors showing important biological activity including antiviral and cytostatic effect.2 On the other hand, little is known about biological activity of 3-substituted adenines. Arylation at purine ring nitrogens in the DNA may occur in vivo by the attack of arene oxides, reactive metabolic intermediates derived from aromatic hydrocarbons, followed by elimination of water.3 Modifications at the N3-position of adenine in the DNA are of special interest, because they result in destabilisation of the N-gylcosidic bond. N3-Alkyl- and aryladenines are then cleaved off the DNA strand and can be therefore found in body fluids, mainly in urine.4 Unlike in the double stranded DNA, the N3 position in adenine is not a prominent site of attack by alkylating or arylating reagents so that adenine itself cannot be used for preparation of 3-aryl-derivatives.5 In our previous work on 3-alkyladenines we found that 8-bromoadenine (1) can be successfully used as a precursor to adenine giving satisfactory yields of N3-alkylated products. After alkylation it can be easily converted to adenine derivatives by Pd-catalysed debromination.6 In this work we studied the selectivity of Chan-Lam-Evans arylation7 of 1 with arylboronic acids (2a-f) with the aim to prepare 3-aryladenines. Some of these, namely 3-phenyladenine, will be used in toxicological studies on the DNA adducts arising from exposure to benzene.
RESULTS AND DISCUSSION
N-Arylation of adenine and 8-bromoadenine. According to a previous report by Bakkestuen and Gundersen Cu(II)-mediated arylation of adenine by arylborobic acids in DCM was unsuccessful due to the lack of solubility.8 This problem was solved using bis-Boc-protected adenine as a more soluble adenine precursor.9 More recently, unprotected adenine was arylated efficiently at N9 position with arylboronic acids using Cu(II) and TMEDA in aqueous methanol.10 Similarly, we found that the protection of adenine was not needed when phenanthroline was used as a ligand instead of triethylamine and DMF as a solvent. Under these conditions the arylation of adenine with phenylboronic acid (2a), yielded 69 % of 9-phenyladenine (3a).
Similarly, 4-vinylphenylboronic acid at the same reaction conditions gave 60% of 9-(4-vinylphenyl)-adenine (3b). No aryladenines except 9-aryl isomers were formed by arylation of adenine. In the endeavour to obtain 3-aryladenines (4a-f) 8-bromoadenine was used as a precursor to adenine having a different charge distribution (Scheme 1). Reaction conditions for its arylation were first tested with 2a as an arylating reagent. The reaction in DCM at room temperature gave poor yields (< 5%) because of poor solubility of 1 in this solvent. Somewhat better yields were obtained in methanol (10 - 21%). These were further improved when DMF was used as a solvent. Selectivity of the reaction was greatly influenced by the ligand used and by reaction temperature (Table 1). Low reaction temperature and phenanthroline as the ligand led to an increase in the proportion of 3-phenyl-8-bromoadenine (4a). On the other hand,
N,N,N’,N’-Tetramethylethylenediamine (TMEDA) gave mainly 9-phenyl-8-bromoadenine (3a) while 2,2’-bipyridyl gave a 1:1 mixture of 3a and 4a. In the latter case, however, the yield of arylation was poor. Optimised reaction conditions were used for arylation of 1 with a series of six arylboronic acids (Table 2).
The reaction proceeded smoothly under aerobic conditions requiring at least one equivalent of copper(II) salt. In an argon atmosphere the yields were significantly lower. Final products were obtained by reductive debromination with hydrogen on palladium. However, in the case of three tolyl derivatives the N9 substituted adenines 5c-5e were formed in the course of arylation so that 8-bromo-9-tolyladenines 3c – 3e could not be isolated. In these cases a mixture of 8-bromo-3-tolyladenine (3c-3e) with 9-tolyladenine (5c-5e) was obtained. The mechanism of reductive debromination under the conditions of arylation is not known. Such a reaction can be explained by formation of an 8-purinylcuprate, which would give 8-H-purine derivative in the protic environment containing water from phenanthroline hydrate and acidic NH from purine. Reductive debromination of all aryl-8-bromoadenines obtained was accomplished with hydrogen on palladium with 25 – 36 % yield. Resulting isomeric aryladenines were separated by column chromatography. For vinylphenyl derivatives 3b and 4b, however, this method led to a simultaneous hydrogenation of the vinyl group yielding corresponding p-ethylphenyl derivatives 5g and 6g, respectively.
Further attempts to selectively remove bromine from 3b and 4b failed either for a lack of reactivity or for a parallel reduction of the vinyl group. So, reduction with tributyltin hydride in the presence of AIBN11 gave ethylphenyl-adenine derivatives 5g and 6g, respectively. On the other hand, 3b reacted with tert-butyllithium in toluene at 60°C to give expected product 5b whereas 3-aryl isomer 4b did not react at the same reaction conditions at all. Starting material was recovered from the reaction mixture (Scheme 2). Similarly, a model reaction of 9-benzyl-8-bromoadenine (7) with tert-butyllithium gave 9-benzyladenine (8) in a 50% yield whereas 3-benzyl-8-bromoadenine (9) did not react at all.
Structure assignment of the isomeric products 3 - 6 was done on the basis of 1D and 2D NMR spectra (HMQC and HMBC). The most apparent distinctive feature of 3-aryladenines 3a-f and 6a-f as compared to corresponding 9-aryl derivatives was the presence of two exchangable NH signals in 1H-NMR spectra as broad singlets at nearly 8.2 and 8.4 ppm (Figure 1).
These two signals indicate that at least in the DMSO solution 3-aryladenines are present in a tautomeric form with two different NH groups (Figure 2, structure A and B). However, according to previous quantum chemical calculations10 the most stable isomer is the one with exocyclic NH2 group (Figure 2, structure C).
Several diagnostic signals in one dimensional 1H NMR and 13C NMR spectra were found, which can be used to distinguish between N3 and N9 aryl adenines and bromoadenines. When comparing with corresponding chemical shift values in adenine, 3-aryladenines show a marked downfield shift for C2-H protons whereas C8-H protons are shifted upfield. In contrast, in 9-aryladenins both C2-H and C8-H signals are shifted downfield by nearly 0.08-0.11 (except for 5c, which is shifted upfield by 0.1 ppm) and 0.13-0.49 ppm, respectively.
Analogously, 3-aryl-8-bromodenines showed a strong downfield shift by 0.14-0.34 ppm for C2-H while N9-substitution has little effect on C2-H (0.07-0.08 ppm upfield). Hence, an aryl substitution at neighbouring nitrogen has a deshielding effect on purine ring hydrogens in both adenine and 8-bromoadenine.
An aryl substitution at distant nitrogen, however, may have either little effect or, in the case of 3-aryladenine even a shielding effect. In 13C NMR spectra C8 signals were strongly influenced by aryl substitution at N3 while C2 signals were not. On the other hand, N9 aryl substitution caused a significant downfield shift of C2 signals (about 8 ppm) and a somewhat weaker upfield shift (5-6 ppm) of C8 signals. Other signals remained almost unchanged. In 8-bromoadenines both N3 and N9 substitution caused a downfiled shift of the C8 signals whereas C2 signals were slightly shifted downfield by N9 aryl and upfiled by N3 aryl substitution. Diagnostic chemical shifts (Δ = δi - δa) are listed in the Table 3.
EXPERIMENTAL
Column chromatography was performed on silica gel 60 purchased from Fluka, particle size 0.063-0.200 mm. For thin-layer chromatography Merck Silica gel 60 F254 plates were used. Dimethylformamide (DMF) was dried by vacuum distillation from phosphorus pentoxide and stored over molecular sieves. Other chemicals obtained from commercial sources were of analytical or synthetic grade and were used as received. 1H and 13C NMR spectra were recorded with Bruker Avance DRX500 (500 MHz for 1H) or with Varian Mercury 300 (300 MHz for 1H) Fourier transform NMR spectrometer. In the HMBC experiments, the parameters were set to show cross-peaks for nuclei interacting with a JH,C coupling constant of 7 Hz. High resolution mass spectra were measured on an Autospec Ultra sector mass spectrometer (Micromass).
Arylation of adenine: A mixture of adenine (1 mmol), boronic acid 2a or 2b (1.5 mmol), phenanthroline (1.5 mmol), and copper(II) acetate (1.5 mmol) in DMF (5 mL) was heated in an oil bath to 90°C under aerobic conditions for 24 h. Progress of the reaction was monitored by TLC with a mixture of CHCl3 and MeOH 10/1 as an eluent. DMF was distilled off in a vacuum, MeOH was added to the residue and the resulting suspension was filtred through a layer of Kieselguhr. After evaporation of the solvent in a vacuum column chromatography on silica gel was carried out using CHCl3/MeOH 20/1 followed by CHCl3/MeOH 10/1 for elution. Analytically pure compounds 5a and 5b were obtained by crystallization from toluene.
9-Phenyladenine (5a): white crystals, yield 69%, Rf = 0.48; mp 213-215°C; also obtained by debromination of 3a (yield 40%); 1H NMR (DMSO-d6): δ = 7.45 – 7.87 (m, 5H, Ar-H); 8.20 (s, 1H, C2-H); 8.57 (s, 1H, C8-H); 13C NMR (DMSO-d6): 119.3 (C5); 123.2, 127.7 and 129.7 (Ar CH); 135.2 (Ar C); 139.9 (C8); 149.3 (C4); 153.8 (C2); 156.3 (C6). Anal. Calcd for C11H9N5 × 1/3H2O: C, 60.8; H, 4.5; N, 32.1. Found: C, 60.5; H, 4.1; N, 32.2.
9-(4-Vinylphenyl)adenine (5b): white crystals, yield 60 %, Rf = 0.52; mp 234-236°C; also obtained by debromination of 3b by tert-butyllithium (yield 31%); 1H NMR (DMSO-d6): δ = 5.35 (d, 1H, J = 11.2 Hz, =CH2); 5.93 (d, 1H, J = 17.8 Hz, =CH2); 6.82 (dd, 2H, J = 11.2 and 17.8 Hz, =CH); 7.40 (br s, 2H, NH); 7.69 (d, 2H, J = 8.5 Hz, Ar-H); 7.91 (d, 2H, J = 8.5 Hz, Ar-H); 8,23 (s, 1H, C2-H); 8.62 (s, 1H, C8-H); 13C NMR (DMSO-d6): 116.0 (=CH2); 120.2 (C5); 123.7 and 128.0 (Ar CH); 135.4 (Ar CN); 136.5 (=CH); 137.0 (Ar CC); 140.3 (C8); 150.0 (C4); 154.0 (C2); 157.2 (C6). Anal. Calcd for C13H11N5: C, 65.8; H, 4.7; N, 29.5. Found: C, 65.6; H, 4.6; N, 29.7.
General procedure for the arylation of 8-bromoadenine: A mixture of 8-bromoadenine (1 mmol), arylboronic acid (1.5 mmol), phenanthroline (1.5 mmol), and copper(II) acetate (1.5 mmol) in DMF (5 mL) was heated in an oil bath to 90°C under aerobic conditions for 24 h. Progress of the reaction was monitored by TLC with a mixture of CHCl3 and MeOH 10/1 as an eluent. DMF was distilled off in a vacuum, MeOH was added to the residue and the resulting suspension was filtred through a layer of Kieselguhr. After evaporation of the solvent in a vacuum, column chromatography on silica gel was carried out using CHCl3/MeOH 20/1 followed by CHCl3/MeOH 10/1 for elution. N3 and N9 arylated products were either incompletely separated or co-eluted in the same fractions, thus their ratio was determined from integral intensities of appropriate signals in 1H NMR spectra. Pure samples of aryl-8-bromoadenines 3 and 4 were obtained by semi-preparative reversed phase HPLC on a 10 × 250 mm column Biospher PSI00 C18, particle size 10 µ using a MeOH – H2O mixture as an eluent at a flow rate of 2.5 mL/min.
8-Bromo-3-phenyladenine (4a): white solid; mp > 250°C; 1H NMR (DMSO-d6): δ = 7.56 – 7.74 (m, 5H, Ar-H); 8.30 and 8.44 (br s, 2H, NH2); 8.48 (s, 1H, C2-H); 13C NMR (DMSO-d6): δ = 121.1 (C5); 125.7, 129.2 and 129,4 (Ar C-H); 137.2 (Ar C); 139.4 (C8); 143.5 (C2); 149.6 (C4); 153.5 (C6); HMBC: Cross-peak of C2-H δ = 8.48 ppm with Ar C-N at 137.2 ppm. Anal. Calcd for C11H8N5Br × 2/5 H2O: C, 44.4; H, 3.0; N, 23.5. Found: C, 44.6; H, 2.6; N, 23.4.
8-Bromo-9-phenyladenine (3a): white solid; mp 242-243°C; 1H NMR (DMSO-d6): δ = 7.52 – 7.62 (m, 5H, Ar-H); 8.07 (s, 1H, C2-H); 13C NMR (DMSO-d6): 118.9 (C5); 126.4 (C8); 128.2, 129.4 and 129,5 (Ar CH); 133.8 (Ar C); 152.0 (C4); 153.3 (C2); 154.9 (C6). Anal. Calcd for C11H8N5Br: C, 45.5; H, 2.8; N, 24.1. Found: C, 45.8; H, 2.4; N, 24.4.
8-Bromo-3-(4-vinylphenyl)adenine (4b): yellowish solid; mp > 250°C; 1H NMR (DMSO-d6): δ = 5.38 (d, 1H, J = 10.8 Hz, =CH2); 5.96 (d, 1H, J = 17.8 Hz, =CH2); 6.84 (dd, 1H, J = 10.8 and 17.8 Hz, =CH); 7.69 (s, 4H, Ar-H); 8.28 (br s, 1H, NH); 8.43 (br s, 1H, NH); 8.49 (s, 1H, C2-H). 13C NMR (DMSO-d6): 116.1 (=CH2); 121.1 (C5); 125.8 and 127.0 (Ar CH); 135.5 (=CH); 136.4 (Ar CN); 138.0 (Ar CC); 139.4 (C8); 143.4 (C2); 149.5 (C4); 153.5 (C6); HMBC: Cross-peak of C2-H at δ = 8.49 ppm with Ar C-N at 136.4 ppm. Anal. Calcd for C13H10N5Br: C, 49.4; H, 3.2; N, 22.2. Found: C, 49.3; H, 3.2; N, 22.1.
8-Bromo-9-(4-vinylphenyl)adenine (3b): yellowish solid; mp 214-216°C; 1H NMR (DMSO-d6): δ = 5.40 (d, 1H, J = 10.9 Hz, =CH); 5.97 (d, 1H, J = 17.6 Hz, =CH); 6.85 (dd, 1H, J = 10.9 and 17.6 Hz, =CH); 7.49 (d, 2H, J = 7.9 Hz, Ar-H); 7.67 (d, 2H, J = 8.2 Hz, Ar-H); 8.06 (s, 1H, C2-H). 13C NMR (DMSO-d6): 116.3 (=CH2); 118.9 (C5); 126.5 (C8); 127.0 and 128.4 (Ar CH); 133.1 (Ar CN); 135.6 (=CH); 138.3 (Ar CC); 151.8 (C4); 153.4 (C2); 155.0 (C6). HRMS: Calcd for C13H10N5Br: 315.0120. Found 315.0105.
8-Bromo-3-(2-tolyl)adenine (4c): yellowish solid; decomposition at 235°C; 1H NMR (DMSO-d6): δ = 2.05 (s, 3H, CH3); 7.41 – 7.52 (m, 4H, Ar-H); 8.28 (br s, 1H, NH); 8.35 (s, 1H, C2-H); 8.45 (br s, 1H, NH). 13C NMR (DMSO-d6): 17.1 (CH3); 121.0 (C5); 127.1, 127.7, 130.1 and 131.1 (Ar C-H); 134.8 (Ar C-C); 136.3 (Ar C-N); 139.6 (C8); 144.0 (C2); 150.0 (C4); 153.7 (C6); HMBC: Cross-peak of C2-H at δ = 8.35 ppm with Ar C-N at 136.3 ppm. HRMS: Calcd for C12H10N5Br: 303.0120; Found 303.0122.
8-Bromo-3-(3-tolyl)adenine (4d): yellowish solid; mp 248-249°C; 1H NMR (DMSO-d6): δ = 2.43 (s, 3H, CH3); 7.39 – 7.56 (m, 4H, Ar-H); 8.30 (br s, 1H, NH); 8.43 (br s, 1H, NH); 8.46 (s, 1H, C2-H). 13C NMR (DMSO-d6): 21.7 (CH3); 122.0 (C5); 123.7, 126.9, 130.1 and 130.7 (Ar CH); 138.0 (Ar C-N); 140.0 (Ar CC); 140.3 (C8); 144.3 (C2); 150.5 (C4); 154.4 (C6); HMBC: Cross-peak of C2-H at δ = 8.46 ppm with Ar C-N at 138.0 ppm. HRMS: Calcd for C12H10N5Br: 303.0120; Found 303.0119.
8-Bromo-3-(4-tolyl)adenine (4e): yellowish solid; mp 244-245°C; 1H NMR (DMSO-d6): δ = 2.40 (s, 3H, CH3); 7.40 (d, 2H, J = 8.3 Hz, Ar-H); 7.58 (d, 2H, J = 8.3 Hz, Ar-H); 8.24 (br s, 1H, NH); 8.38 (br s, 1H, NH); 8.39 (s, 1H, C2-H). 13C NMR (DMSO-d6): 20.7 (CH3); 121.2 (C5); 125.5 and 129.8 (Ar C-H); 134.7 (Ar C-N); 138.9 (Ar C-C); 139.4 (C8); 143.5 (C2); 149.7 (C4); 153.5 (C6); HMBC: Cross-peak of C2-H at δ = 8.39 ppm with Ar C-N at 134.7 ppm. Anal. Calcd for C12H10N5Br: C, 47.4; H, 3.3; N, 23.0. Found: C, 47.6; H, 3.5; N, 23.4.
8-Bromo-3-(4-methoxyphenyl)adenine (4f): yellowish solid; mp > 250°C; 1H NMR (DMSO-d6): δ = 3.85 (s, 3H, CH3); 7.15 (d, 2H, J = 8.8 Hz, Ar-H); 7.65 (d, 2H, J = 8.8 Hz, Ar-H); 8.25 (br s, 1H, NH); 8.40 (br s, 1H, NH); 8.43 (s, 1H, C2-H). 13C NMR (DMSO-d6): 56.5 (CH3); 122.0 (C5); 115.4 and 127.9 (Ar C-H); 130.9 (Ar C-N); 140.3 (C8); 144.4 (C2); 150.8 (C4); 154.4 (C6); 160.5 (Ar C-O); HMBC: Cross-peak of C2-H at δ = 8.43 ppm with Ar C-N at 130.9 ppm. HRMS: Calcd for C12H10N5OBr: 319.0069. Found 319.0055.
8-Bromo-9-(4-methoxyphenyl)adenine (3f): yellowish solid; mp 248-249°C; 1H NMR (DMSO-d6): δ = 2.51 (s, 3H, OCH3); 7.14 (d, 2H, J = 8.7 Hz, Ar-H); 7.65 (d, 2H, J = 8.7 Hz, Ar-H); 7.47 (br s, 2H, NH); 8.07 (s, 1H, C2-H). 13C NMR (DMSO-d6): δ = 56.4 (CH3); 119.7 (C5); 127.3 (Ar CN); 115.4 and 130.3 (Ar C-H); 140.3 (C8); 152.8 (C4); 154.1 (C2); 155.8 (C6); 160.7 (Ar C-O). HRMS: Calcd for C12H10N5OBr: 319.0069. Found: 319.0063.
General procedure for reductive debromination of aryl-8-bromoadenines:
Pure isomers or a mixture of 3 and 4 (0.66mmol) and palladium catalyst (10 % Pd-C; 35mg; 5% mol.) and ammonium formate (250mg) in absolute MeOH (25mL) was heated in an oil bath to 60°C under argon atmosphere for 6 h. The catalyst was then filtred off and washed with aqueous MeOH. The solvent was evaporated in vacuo to yield crude product mixture of 5 and 6, which was separated by column chromatography on silica gel using a stepwise gradient of CHCl3/MeOH 10/1 to 6/1 for elution. Products were purified by crystallization from aqueous EtOH or MeOH.
3-Phenyladenine (6a): Obtained by debromination of 4a (yield 37 %) or a from a mixture of 3a and 4a as white crystals; decomposition at 201°C; 1H NMR (DMSO-d6): δ = 7.54 – 7.79 (m, 5H, Ar-H); 7.78 (s, 1H, C8-H); 8.16 and 8.34 (br s, 2H, NH2); 8.50 (s, 1H, C2-H); 13C NMR (DMSO-d6): 120.1 (C5); 125.6, 129.0 and 129.3 (Ar CH); 137.8 (Ar C); 143.0 (C2); 149.6 (C4); 152.6 (C8); 154.9 (C6); HMBC: Cross-peak of C2-H (δ = 8.50 ppm with Ar C at 137.8 ppm. Anal. Calcd for C11H9N5 × 1.45 H2O: C, 55.67; H, 5.02; N, 29.52. Found: C, 55.35; H, 4.66; N, 29.87.
3-(2-Tolyl)adenine (6c): Obtained by debromination of 4c (yield 24 %) or a 1 : 1 mixture of 4c and 5c as yellowish crystals; Rf = 0.14; mp > 250°C; 1H NMR (DMSO-d6): δ = 2.04 (s, 3H, CH3); 7.40 – 7.53 (m, 4H, Ar-H); 7.76 (s, 1H, C8-H); 8.17 (br s, 1H, N-H); 8.23 (br s, 1H, N-H); 8.36 (s, 1H, C2-H). 13C NMR (DMSO-d6): δ = 17.2 (CH3); 119.3 (C5); 127.0, 127.7, 129.8 and 131.0 (Ar C-H); 134.9 (Ar C-C); 136.8 (Ar C-N); 143.8 (C2); 149.8 (C4); 152.3 (C8); 154.9 (C6); HMBC: Cross-peak of C2-H at δ = 8.36 ppm with Ar C-N at 136.8 ppm. Anal. Calcd for C12H11N5 × 1/4 H2O: C, 62.7; H, 5.1; N, 30.5. Found: C, 62.9; H, 4.9; N, 30.6.
9-(2-Tolyl)adenine (5c): Obtained by arylation of bromoadenine 1 with 2c followed by separation on a silica gel column (yield 15 %) as white crystals from aqueous EtOH; Rf = 0.48; mp 197-199°C; 1H NMR (DMSO-d6): δ = 2.08 (s, 3H, CH3); 7.35 (br s, 2H, NH); 7.39 – 7.46 (m, 4H, Ar-H); 8.11 (s, 1H, C2-H); 8.26 (s, 1H, C8-H); 13C NMR (DMSO-d6): 17.5 (CH3); 118.4 (C5); 126.9, 127.9, 129.1 and 131.0 (Ar CH); 133.7 (Ar CN); 134.9 (Ar CC); 140.8 (C8); 150.1 (C4); 153.0 (C2); 156.3 (C6). Anal. Calcd. for C12H11N5 × 1/4 EtOH: C, 63.4; H, 5.3; N, 29.6. Found: C, 63.7; H, 5.0; N, 29.8.
3-(3-Tolyl)adenine (6d): Obtained by debromination of 4d (yield 58 %) or a mixture of 4d and 5d as white crystals; Rf = 0.16; mp > 250°C; 1H NMR (DMSO-d6): δ = 2.41 (s, 3H, CH3); 7.36 – 7.60 (m, 4H, Ar-H); 7.76 (s, 1H, C8-H); 8.10 (br s, 1H, NH); 8.23 (br s, 1H, NH); 8.47 (s, 1H, C2-H). 13C NMR (DMSO-d6): 20.86 (CH3); 120.1 (C5); 122.6, 126.0, 129.1 and 129.5 (Ar CH); 137.7 (Ar CC); 139.0 (Ar CN); 142.8 (C2); 149.6 (C4); 152.6 (C8); 154.8 (C6); HMBC: Cross-peak of C2-H at δ = 8.47 ppm with Ar C-N at 139.0 ppm. Anal. Calcd for C12H11N5 × 1/8 H2O: C, 63.4; H, 5.0; N, 30.8. Found: C, 63.3; H, 4.9; N, 30.9.
9-(3-Tolyl)adenine (5d): Obtained by arylation of bromoadenine 1 with 2d followed by separation on a silica gel column (yield 26 %) as white crystals; Rf = 0.51; mp 225-226°C; 1H NMR (DMSO-d6): δ = 2.39 (s, 3H, CH3); 7.35 (br s, 2H, NH); 7.24 – 7.68 (m, 4H, Ar-H); 8.20 (s, 1H, C2-H); 8.53 (s, 1H, C8-H); 13C NMR (DMSO-d6): 20.9 (CH3); 119.1 (C5); 120.1, 123.4, 128.1 and 129.3 (Ar C-H); 134.9 (Ar CN); 139.1 (Ar CC); 139.7 (C8); 149.0 (C4); 153.1 (C2); 156.2 (C6). Anal. Calcd for C12H11N5: C, 64.0; H, 4.9; N, 31.1. Found: C, 63.8; H, 4.9; N, 31.1.
3-(4-Tolyl)adenine (6e): Obtained by debromination of 4e (yield 47 %) or a mixture of 4e and 5e as white crystals; Rf = 0.16; mp > 250°C; 1H NMR (DMSO-d6): 2.42 (s, 3H, CH3); 7.41 (d, 2H, J = 7.9 Hz, Ar-H); 7.65 (d, 2H, J = 7.9 Hz, Ar-H); 7.76 (s, 1H, C8-H); 8.10 (br s, 1H, NH); 8.17 (br s, 1H, NH); 8.46 (s, 1H, C2-H). 13C NMR (DMSO-d6): 20.66 (CH3); 119.5 (C5); 125.4 and 129.7 (Ar CH); 135.3 (Ar CC); 138.6 (Ar CN); 142.9 (C2); 152.6 (C8); 154.2 (C4); 154.8 (C6); HMBC: Cross-peak of C2-H at δ = 8.46 ppm with Ar C-N at 135.3 ppm. Anal. Calcd for C12H11N5 × 2 1/4 H2O: C, 54.2; H, 5.9; N, 26.4. Found: C, 54.3; H, 6.0; N, 26.3.
9-(4-Tolyl)adenine (5e): Obtained by arylation of bromoadenine 1 with 2e followed by separation on a silica gel column (yield 22 %) as white crystals; Rf = 0.49; mp > 250°C; 1H NMR (DMSO-d6): δ = 2.37 (s, 3H, CH3); 7.32 (br s, 2H, NH); 7.37 (d, 2H, J = 7.8 Hz, Ar-H); 7.71 (d, 2H, J = 7.8 Hz, Ar-H); 8.18 (s, 1H, C2-H); 8.50 (s, 1H, C8-H); 13C NMR (DMSO-d6): 20.8 (CH3); 119.3 (C5); 123.2 and 130.2 (Ar CH); 132.7 (Ar CN); 137.4 (Ar CC); 140.0 (C8); 149.3 (C4); 153.4 (C2); 156.4 (C6). Anal. Calcd for C12H11N5: C, 64.0; H, 4.9; N, 31.1. Found: C, 63.8; H, 5.1; N, 30.9.
3-(4-Methoxyphenyl)adenine (6f): Obtained by debromination of 4f (yield 43 %) or a mixture of 3f and 4f as white crystals from aqueous EtOH; Rf = 0.14; mp > 250°C; 1H NMR (DMSO-d6): δ = 3.85 (s, 3H, CH3); 7.14 (d, 2H, J = 8.7 Hz, Ar-H); 7.68 (d, 2H, J = 8.7 Hz, Ar-H); 7.77 (s, 1H, C8-H); 8.10 (br s, 1H, NH); 8.26 (br s, 1H, NH); 8.44 (s, 1H, C2-H). 13C NMR (DMSO-d6): 56.44 (CH3); 115.3 (Ar CH); 120.9 (C5); 127.8 (Ar CH); 131.5 (Ar CN); 143.9 (C2); 150.7 (C4); 153.4 (C8); 155.6 (C6); 160.3 (Ar C-O); HMBC: Cross-peak of C2-H at δ = 8.44 ppm with Ar C-N at 131.5 ppm. Anal. Calcd for C12H11N5O × 1/3 EtOH: C, 59.3; H, 5.1; N, 27.3. Found: C, 58.9; H, 5.2; N, 27.3.
9-(4-Methoxyphenyl)adenine (5f): Obtained by debromination of 3f (yield 52 %) or a mixture of 3f and 4f as white crystals from aqueous ethanol; Rf = 0.46; mp 233-234°C; 1H NMR (DMSO-d6): δ = 3.83 (s, 3H, CH3); 7.13 (d, 2H, J = 8.7 Hz, Ar-H); 7.32 (br s, 2H, NH); 7.76 (d, 2H, J = 8.8 Hz, Ar-H); 8.19 (s, 1H, C2-H); 8.48 (s, 1H, C8-H); 13C NMR (DMSO-d6): δ = 56.4 (CH3); 115.4 (Ar CH); 120.0 (C5); 125.5 (Ar CH); 128.9 (Ar CN); 140.6 (C8); 150.1 (C4); 153.9 (C2); 157.2 (C6); 159.3 (Ar C-O). Anal. Calcd. for C12H11N5O × 1/2 EtOH: C, 59.1; H, 5.3; N, 27.0. Found: C, 59.4; H, 5.2; N, 26.8.
ACKNOWLEDGEMENT
The financial support by grants No. 203/06/0888 from the Grant Agency of the Czech Republic and grants MSM 604 613 73 01 and LC06070 from the Ministry of Education of the Czech Republic is gratefully acknowledged.
References
1. J. J. Strouse, M. Ješlnik, and J. B. Arterburn, Acta Chim. Slov., 2005, 52, 187; M. Williams and M. F. Jarvis, Biochem. Pharmacol., 2000, 59, 1173; CrossRef J. T. Davis, Angew. Chem. Int. Ed., 2004, 43, 668. CrossRef
2. E. DeClercq, J. Deschamps, P. DeSomer, and A. Holý, Science, 1978, 200, 563; CrossRef A. Holý, Chem. Listy, 1987, 81, 461; V. I. Petrov, A. A. Ozerov, M. S. Novikov, C. Pannecouque, J. Balzarini, and E. De Clercq, Chem. Heterocycl. Comp., 2003, 39, 1218. CrossRef
3. A. M. Medeiros, M. G. Bird, and G. Witz, J. Toxicol. Environ. Health, 1997, 51, 519; CrossRef I. Oesch, D. M. Jerina, and J. W. Daly, Arch. Biochem. Biophys., 1971, 144, 253; CrossRef J. Angerer, M. Schildbach, and A. Kramer, J. Anal. Toxicol., 1998, 22, 211.
4. D. E. G. Shuker and P. B. Farmer, Chem. Res. Toxicol., 1992, 5, 450; CrossRef G. Eberle, K.-H. Gluesenkamp, W. Drosdziok, and M. F. Rajewsky, Carcinogenesis, 1990, 11, 1753. CrossRef
5. M. Koskinen, P. Vodicka, and K. Hemminki, Chem.-Biol. Interact., 2000, 124, 13. CrossRef
6. J. Krouželka, I. Linhart, and T. Tobrman, J. Heterocycl. Chem., 2008, 45, 789. CrossRef
7. D. M. T. Chan, K. L. Monaco, R.-P. Wang, and M. P. Winters, Tetrahedron Lett., 1998, 39, 2933; CrossRef D. A. Evans, J. L. Katz, and T. R. West, Tetrahedron Lett., 1998, 39, 2937; CrossRef P. Y. S. Lam, C. G. Clark, S. Saubern, J. Adams, M. P. Winter, D. M. T. Chan, and A. Combs, Tetrahedron Lett., 1998, 39, 2941; CrossRef S. V. Ley and A. W. Thomas, Angew. Chem. Int. Ed., 2003, 42, 5400. CrossRef
8. A. K. Bakkestuen and L.-L. Gundersen, Tetrahedron Lett., 2003, 44, 3359. CrossRef
9. M. F. Jacobsen, M. M. Knudsen, and K. V. Gothelf, J. Org. Chem., 2006, 71, 9183. CrossRef
10. L. Tao, Y. Yue, J. Zhang, S.-Y. Chen, and X. Q. Yu, Helv. Chim. Acta, 2008, 91, 1008. CrossRef
11. S. Tussetschlaeger, A. Baro, S. Laschat, and W. Frey, Eur. J. Org. Chem., 2007, 5590. CrossRef
12. K.-H. Gluesenkamp, K. Krueger, G. Eberle, W. Drosdziok, E. Jaehde, O. Gruendel, A. Neuhaus, R. Boese, P. Stellberg, and M. F. Rajewsky, Angew. Chem., Int. Ed. Engl., 1993, 32, 1640. CrossRef