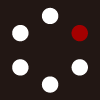
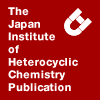
HETEROCYCLES
An International Journal for Reviews and Communications in Heterocyclic ChemistryWeb Edition ISSN: 1881-0942
Published online by The Japan Institute of Heterocyclic Chemistry
e-Journal
Full Text HTML
Received, 8th February, 2010, Accepted, 23rd March, 2010, Published online, 24th March, 2010.
DOI: 10.3987/COM-10-11922
■ Synthesis of (3S)-2,5-Diethoxy-3,6-dihydro-3-isopropyl-6-methylsulfanylpyrazines and Stereoselectivity in Alkylation of the Anions
Yoshihide Usami,* Masao Arimoto,* Chisaka Kioka, Masako Yamanaka, Naoko Matsuda, and Hayato Ichikawa
Osaka University of Pharmaceutical Sciences, 4-20-1 Nasahara, Takatsuki, Osaka 569-1094, Japan
Abstract
The introduction of a sulfur function into Schöllkopf’s bislactim ether was carried out to synthesize the diastereomers of (3S)-2,5-diethoxy-3,6-dihydro-3-isopropyl-6-methylsulfanylpyrazine. Stereoselectivity in the alkylation of the anions derived from those compounds was examined as a basic study of the synthesis of chiral α-sulfanylamino acids.INTRODUCTION
Sulfur-containing diketopiperazines,1 such as gliotoxins,2 gliovictin,3 sporidesmins,4 aranotins,5,6 verticillins,7 chaetocins,8 and leptosins9,10 (Figure 1), make up a large category of natural products with significant importance because they exhibit various biological activities. We also had reported the isolation of cytotoxic natural products possessing the diketopiperazine structure with a sulfur function at the α-position, as the metabolites of fungi originally isolated from marine resources.11,12 Several methods for the total synthesis of this type of natural products are available. However, most of them yielded racemic compounds.13-19 in spite of the intensive effort to synthesize non-natural but chiral diketopiperazines bearing sulfur functions20-27 Very recently, a great milestone was achieved by Movassaghi and co-workers who reported the total synthesis of (+)-11,11’-deoxyverticillin A.28
The asymmetric syntheses of sulfur-containing diketopiperazine natural products pose a great challenge for organic chemists and are a vital step in the development of new drugs. As we are interested in cyclic dipeptides consisting of two α-thioamino acids, the synthesis of the building block seems to be one possible way to achieve our goal. We had examined Schöllkopf’s bislactim ether methodology29-31 for the synthesis of chiral α-thioamino acids. Described herein is the synthesis of the diastereomers of (3S)-2,5-diethoxy-3,6-dihydro-3-isopropyl-6-methylsulfanylpyrazine and the stereoselective alkylation of the anions derived from them.32
RESULTS AND DISCUSSION
As summarized in Scheme 1, there are two ways to furnish α-sulfanylamino acid from Schöllkopf’s bislactim ether 1. Route A involves the initial alkylation, followed by α-sulfanylation. and route B involves the initial introduction of a sulfur function, followed by alkylation.
1. INTRODUCTION OF SULFUR FUNCTION INTO BENZYLATED BISLACTIM ETHER
Route A, our initial approach, is summarized in Scheme 2. Schöllkopf’s bislactim ether 1 was lithiated with n-BuLi, and this was followed by the addition of benzyl bromide to give a mixture of benzylated diastereomers 2a and 2b in 94% d.e. Purely isolated major trans-isomer 2a was treated with n-BuLi, and this was followed by the addition of dimethyl disulfide to afford products 3a and 3b in 95% yield with a ratio of approximately 70 : 30. The stereochemistry of 3a and 3b was deduced from the chemical shift of H-3, which was observed in the higher field at 2.98 ppm for 3a due to the anisotropic effect of the benzyl group located on the same side, compared to 3.78 ppm for 3b, as illustrated in Figure 2. This speculation was confirmed by NOESY analysis.
A NOESY cross peak between H-3 and 6-SMe was observed for 3b whereas 6-SMe/3-isopropyl methyls or 6-SMe/3-isopropyl methine cross peaks were observed for 3a. It is generally accepted that the carbanion derived from 1 has an sp2 hybrid and it mainly reacts with the electrophile at the side opposite to the 3-isopropyl group to yield excellent stereoselectivity. Thus, the low stereoselectivity may be explained by the preference of the sp3-like carbanion derived from 2a for the π-π stabilizing interaction between the bislactim ether ring and the benzyl group.
2. SYNTHESIS OF (3S)-6-METHYLSULFANYL-3-ISOPROPYLBISLACTIM ETHER
As the second approach via route B, bislactim ether 1 was treated with n-BuLi in THF at −78 oC, and this was followed by the addition of dimethyl disulfide to afford a mixture of diastereomers 4a and 4b in a ratio of ca. 1:2. To our surprise, major diastereomer 4b had a cis-configuration between the 3-isopropyl group and the newly introduced 6-methylsulfanyl group. A cross peak between 3-isopropyl methyl/6-SMe in the NOESY spectrum of 4b and cross peaks between H-3/6-SMe and 3-isopropyl methyl/6-H in the NOESY spectrum of 4a confirmed their relative stereochemistry, as illustrated in Scheme 3. One possible explanation for this unusual stereoselectivity is the re-deprotonation of initially formed 4a or 4b by methylthiolate anion in the reaction system, followed by protonation from the less hindered side during the quenching process. However, quenching of the reaction with D2O did not give any deuterated 4a or 4b, negating the above deduction. The reason why 4b was formed predominantly under kinetically controlled conditions remains unknown.
3. ALKYLATION OF (3S)-6-METHYLSULFANYL-3-ISOPROPYLBISLACTIM ETHER
The stereoselectivity in the alkylation of the carbanion derived from 4a or 4b was investigated. The electrophiles selected here will be useful for the synthesis of natural sulfur-containing diketopiperazines. The results are summarized in Table 1. Benzylation showed remarkable stereoselectivity, giving single product 3a from either starting material 4a or 4b or mixture of both (entries 1-3). Methylation also showed the same stereoselectivity (5a:5b = ca. 3:1) from either starting material (entries 4 and 5). Similar low stereoselectivity in the methylation was seen in a previous study by Nagao and co-workers using (L)-serine-derived Schöllkopf’s bislactim ether.37 From the results described above, it became apparent that the stereochemistry of the starting material has no effect on the stereoselectivity of this reaction. Ethylation using iodoethane as an electrophile gave higher stereoselectivity than methylation whereas bromoethane did not yield the same result (entries 7 and 8). The reaction with iodopropane proceeded with excellent stereoselectivity (entry 9). The reaction with piperonyl bromide gave a single diastereomer 8d in 30% yield along with aromatized product 11 (entry 10). The stereoselectivity of this reaction seemed to be related to the bulkiness of the electrophiles.
4. ETHANOLYSIS OF 6-METHYLSULFANYL-BISLACTIM ETHER
The ethanolysis of bislactim ethers with trifluoroacetic acid was examined. Unfortunately, desulfanylated pyrazines were obtained as products 9−13 instead of the desired ethyl esters of α-methylsulfanylamino acids. Nevertheless, it is interesting that the resultant pyrazines, such as 9 or 13, are closely related to fungal metabolites terezine A38 and septorine.39
CONCLUSION
We have synthesized the diastereomers of (3S)-2,5-diethoxy-3-isopropyl-6-methylsulfanylpiperazine and examined the stereoselectivity in the alkylation of the anions derived from them. Benzylation showed excellent stereoselectivity whereas methylsulfanylation of benzylated bislactim ether showed low selectivity. Acid-catalyzed hydrolysis gave not the desired α-sulfur substituted amino acids but desulfanylated and aromatized pyrazines.
EXPERIMENTAL
General Procedure. IR spectra were obtained with a JEOL FT/IR-680 Plus spectrometer. HRMS were determined with a JEOL JMS-700 (2) mass spectrometer. NMR spectra were recorded at 27 oC on Varian UNITY INOVA-500, Gemini-2000, and Mercury-3000 spectrometers in CDCl3 with tetramethylsilane (TMS) as the internal standard. Melting points were determined on a Yanagimoto micromelting point apparatus and are uncorrected. Specific rotations were determined by ORD measurements using a JASCO J-820 spectrometer. Liquid column chromatography was conducted over silica gel (SILICYCLE, SiliaFlash® F60, mesh 230−400). Analytical TLC was performed on precoated Merck aluminum sheets (DC-Alufolien Kieselgel 60 F254), and compounds were viewed by spraying an ethanol solution of phosphomolybdic acid, followed by heating. Dry THF was distilled over sodium-benzophenone ketyl under argon atmosphere.
Benzylation of 1
To a solution of 1 (862.5 mg, 4.1 mmol) in dry THF (50 mL) was added n-BuLi (1.6 M 2.8 mL, 4.5 mmol) at −78 oC under nitrogen atmosphere. After stirring for 30 min, benzyl bromide (0.53 mL, 4.5 mmol) was added and the reaction mixture was stirred overnight to allow the reaction temperature to reach rt. The reaction mixture was treated with aq NH4Cl, evaporated to reduce the organic solvent, and extracted with CH2Cl2. The organic layer was dried over MgSO4, filtered, and evaporated under reduced pressure to afford the crude product, which was purified by silica gel column chromatography (eluent: hexane:CH2Cl2 = 4:1) and preparative TLC (eluent: hexane:CH2Cl2 = 4:1) to give 2a in 86% yield (1.08 g) and 2b in 2% yield (23.2 mg). The product ratio appearing in the text was estimated from the 1NMR spectrum of the crude product. (3S,6R)-2,5-Diethoxy-3,6-dihydro-3-isopropyl-6-benzylpyrazine 2a; oil; [α]D25 −101.3 (c 0.43, EtOH); IR (liquid film) νmax 1693 (C=C) cm-1; 1H-NMR (CDCl3) δ 0.62 (3H, d, J = 6.9 Hz, iPr-CH3), 0.93 (3H, d, J = 6.9 Hz, iPr-CH3), 1.25 (3H, t, J = 7.1 Hz, -OCH2CH3), 1.32 (3H, t, J = 7.1 Hz, -OCH2CH3), 2.16 (1H, sept d, J = 6.9, 3.3 Hz, -CH(CH3)2), 3.07 (1H, dd, J = 13.2, 4.7 Hz, PhCHH-), 3.11 (1H, dd, J = 13.2, 4.7 Hz, PhCHH-), 3.24 (1H, t, J = 3.3 Hz, H-3), 4.05−4.21 (4H, m, 2x -OCH2CH3), 4.30 (1H, td, J = 4.7, 3.3 Hz, H-6), 7.09−7.22 (5H, m, Ph); 13C-NMR (CDCl3) δ 14.3 (q), 14.4 (q), 16.4 (q), 19.0 (q), 31.1 (d), 40.0 (t), 56.6 (d), 60.1 (t), 60.4 (t), 60.5 (d), 126.1 (d), 127.7 (d), 130.0 (d), 137.4 (s), 161.9 (s), 163.5 (s); HRMS m/z calcd for C18H26N2O2 (M)+ 302.1994, found 302.1993. (3S,6S)-2,5-Diethoxy-3,6-dihydro-3-isopropyl-6-benzylpyrazine 2b; oil; [α]D25 +105.5 (c 0.32, EtOH); IR (liquid film) νmax 1692 (C=C) cm-1; 1H-NMR (CDCl3) δ 0.35 (3H, d, J = 6.9 Hz, iPr-CH3), 0.91 (3H, d, J = 6.9 Hz, iPr-CH3), 1.25 (3H, t, J = 7.1 Hz, -OCH2CH3), 1.30 (3H, t, J = 7.1 Hz, -OCH2CH3), 1.86 (1H, sept d, J = 6.9, 4.6 Hz, -CH(CH3)2), 3.04 (1H, dd, J = 13.3, 6.9 Hz, PhCHH-), 3.13 (1H, dd, J = 13.3, 4.6 Hz, PhCHH-), 3.78 (1H, dd, J = 5.0, 4.3 Hz, H-3), 3.99−4.21 (4H, m, 2x -OCH2CH3), 4.27 (1H, dt, J = 6.9, 4.6 Hz, H-6), 7.09−7.25 (5H, m, Ph); 13C-NMR (CDCl3) δ 14.4 (q), 14.4 (q), 16.9 (q), 19.6 (q), 31.3 (d), 40.7 (t), 56.9 (d), 60.3 (t), 60.3 (t), 60.7 (d), 126.2 (d), 127.9 (d), 130.0 (d), 138.2 (s), 161.8 (s), 162.8 (s); HRMS m/z calcd for C18H26N2O2 (M)+ 302.1994, found 302.1991.
Introduction of sulfur function into 2a
To a solution of 2a (486.5 mg, 1.6 mmol) in THF (10 mL) was added n-BuLi (1.6 M 2.8 mL, 4.5 mmol) at −78 oC under nitrogen atmosphere. After stirring for 30 min, dimethyl disulfide (0.14 mL, 1.6 mmol) was added and the reaction mixture was stirred overnight to allow the reaction temperature to reach rt from −78 oC. The reaction mixture was evaporated to reduce the organic solvent, treated with aq Na2CO3, and extracted with CH2Cl2. The organic layer was dried over MgSO4, filtered, and evaporated under reduced pressure to afford the crude product, which was purified by silica gel column chromatography (eluent: hexane:CH2Cl2 = 4:1) to give 3a and 3b in 95% yield (531.0 mg). The product ratio appearing in the text was estimated from the 1H-NMR spectrum.
(3S,6S)-2,5-Diethoxy-3,6-dihydro-3-isopropyl-6-benzyl-6-methylsulfanylpyrazine 3a; pale yellow crystals (CH2Cl2); mp 65−73oC; [α]D25 −11.4 (c 1.16, EtOH); IR (KBr) νmax 1677 (C=C), 1495 (C=C), cm−1; 1H-NMR (CDCl3) δ 0.65 (3H, d, J = 7.0 Hz, iPr-CH3), 0.93 (3H, d, J = 7.0 Hz, iPr-CH3), 1.28 (3H, t, J = 7.1 Hz, -OCH2CH3), 1.38 (3H, t, J = 7.1 Hz, -OCH2CH3), 2.11 (3H, s, SCH3), 2.16 (1H, sept d, J = 7.0, 3.0 Hz, -CH(CH3)2), 2.98 (1H, d, J = 3.4 Hz, H-3), 3.10 (1H, d, J = 12.6 Hz, PhCHH-), 3.40 (1H, d, J = 12.6 Hz, PhCHH-), 4.07−4.29 (4H, m, -OCH2CH3), 7.03−7.07 (2H, m, Ar-H), 7.16−7.21 (3H, m, Ar -H); 13C-NMR (CDCl3) δ 12.8 (q), 14.3 (q), 14.5 (q), 17.1 (q), 19.4 (q), 30.4 (d), 45.9 (t), 60.2 (d), 60.91 (2 x t), 67.5 (s), 126.6 (d), 127.7 (d), 130.2 (d), 136.1 (s), 159.6 (s), 164.4 (s); HRMS m/z calcd for C19H29N2O2S (M+H)+ 349.1950, found 349.1949.
(3S,6R)-2,5-Diethoxy-3,6-dihydro-3-isopropyl-6-benzyl-6-methylsulfanylpyrazine 3b; oil; [α]D25 −6.2 (c 0.33, CHCl3); IR (liquid film) νmax 1680 (C=C), 1495 (C=C) cm−1; 1H-NMR (CDCl3) δ −0.20 (3H, d, J = 6.9 Hz, iPr-CH3), 0.83 (3H, d, J = 6.9 Hz, iPr-CH3), 1.30 (3H, t, J = 7.1 Hz, -OCH2CH3), 1.42 (3H, t, J = 7.1 Hz, -OCH2CH3), 1.93 (3H, s, SCH3), 2.03 (1H, sept d, J = 6.9, 3.4 Hz, -CH(CH3)2 ), 3.12 (1H, d, J = 12.8 Hz, PhCHH-), 3.55 (1H, d, J = 12.8 Hz, PhCHH-), 3.80 (1H, d, J = 3.4 Hz, H-3), 4.14−4.28 (4H, m, -OCH2CH3), 7.11−7.21 (5H, m, Ar-H); 13C-NMR (CDCl3) δ 12.2 (q), 14.4 (q), 14.5 (q), 14.9 (q), 19.2 (q), 30.6 (d), 44.4 (t), 60.5 (d), 60.8 (t), 60.9 (t), 67.5 (s), 126.6 (d), 127.7 (d), 130.8 (d), 136.5 (s), 158.9 (s), 165.1 (s); HRMS m/z calcd for C19H29N2O2S (M+H)+ 349.1950, found 349.1944.
Introduction of sulfur function into 1
To a solution of 1 (202.2 mg, 0.9 mmol) in dry THF (4 mL) was added n-BuLi (1.6 M 0.62 mL, 0.99 mmol) at −78 oC under nitrogen atmosphere. After stirring for 30 min, dimethyl disulfide (0.1 mL, 1.08 mmol) was added and the reaction mixture was stirred overnight to allow the reaction temperature to reach rt. The reaction mixture was evaporated to reduce the organic solvent, treated with aq NH4Cl, and extracted with CH2Cl2. The organic layer was dried over MgSO4, filtered, and evaporated under reduced pressure to afford the crude product, which was purified by silica gel column chromatography (eluent: hexane:CH2Cl2 = 4:1) and preparative TLC (eluent: hexane:CH2Cl2 =10:1) to give a mixture of 4a and 4b in 84% yield (194.3 mg). The product ratio appearing in the text was estimated from the 1NMR spectrum of the crude product.
(3S,6R)-2,5-Diethoxy-3,6-dihydro-3-isopropyl-6-methylsulfanylpyrazine 4a; pale yellow oil; [α]D25 −94.2 (c 0.33, EtOH); IR (liquid film) νmax 1685 (C=C) cm-1; 1H-NMR (CDCl3) δ 0.67 (3H, d, J = 6.8 Hz, iPr-CH3), 1.07 (3H, d, J = 7.0 Hz, iPr-CH3), 1.30 (3H, t, J = 7.1 Hz, -OCH2CH3), 1.32 (3H, t, J = 7.1 Hz, -OCH2CH3), 2.02 (3H, s, 6-SCH3), 2.35−2.42 (1H, qqd, J = 7.0, 6.8, 3.3 Hz, -CH(CH3)2), 3.95 (1H, dd, J =3.3, 3.1 Hz, H-3), 4.13−4.25 (4H, m, -OCH2CH3), 4.97 (1H, d, J = 3.1 Hz, H-6); 13C-NMR (CDCl3) δ 11.8 (q), 14.2 (q), 14.3 (q), 16.3 (q), 19.1 (q), 31.2 (d), 58.2 (d), 60.4 (d), 61.2 (t), 61.3 (t), 159.6 (s), 166.2 (s); HRMS m/z calcd for C12H21N2O2S (M−H)+ 257.1324, found 257.1328.
(3S,6S)-2,5-Diethoxy-3,6-dihydro-3-isopropyl-6-methylsulfanylpyrazine 4b; pale yellow oil; [α]D25 −15.1 (c 0.11, EtOH); IR (liquid film) νmax 1685 (C=C) cm−1; 1H-NMR (CDCl3) δ 0.86 (3H, d, J = 6.8 Hz, iPr-CH3) 1.07 (3H, d, J = 6.8 Hz, iPr-CH3), 1.29 (3H, t, J = 7.1 Hz, -OCH2CH3), 1.32 (3H, t, J = 7.1 Hz, -OCH2CH3), 2.20 (1H, sept d, J = 6.8, 4.6 Hz, -CH(CH3)2), 2.36 (3H, s, 6-SCH3), 3.90 (1H, dd, J = 4.6, 3.7 Hz, H-3), 4.07−4.29 (4H, m, 2x -OCH2CH3), 4.89 (1H, d, J = 3.7 Hz, H-6); 13C-NMR (CDCl3) δ 14.2 (q), 14.3 (q), 15.9 (q), 18.4 (q), 19.8 (q), 32.1 (d), 58.9 (d), 61.2 (t), 61.7 (d), 161.2 (s), 166.8 (s); HRMS m/z calcd for C12H22N2O2S (M)+ 258.1383, found 258.1397.
Alkylation of 4a or 4b
General procedure (Table 1): To a solution of 4a or 4b (ca. 50 mg) in dry THF (2.0 mL) was added n-BuLi (1.6 M 1.2 equiv.) at −78 oC under nitrogen atmosphere. After stirring for 30 min, an electrophile was added and the reaction mixture was stirred overnight to allow the reaction temperature to reach rt from −78 oC. The solvent was removed under reduced pressure to give a residue, which was treated with aq Na2CO3 and extracted with CH2Cl2. The organic layer was washed with brine, dried over MgSO4, filtered, and evaporated under reduced pressure to afford a crude product that was purified by chromatography (eluent: hexane:EtOAc = 10:1) to afford the desired products. The product yield appearing in Table 1 was estimated from the isolated yield of the product mixture and the integration of the signals in the 1H-NMR spectrum.
(3S,6S)-2,5-Diethoxy-3,6-dihydro-3-isopropyl-6-methyl-6-methylsulfanylpyrazine 5a; pale yellow oil; [α]D25 +133.8 (c 0.33, EtOH); IR (liquid film) νmax 1681 (C=C) cm−1; 1H-NMR (CDCl3) δ 0.79 ( 3H, d, J = 6.6 Hz, iPr-CH3) 1.10 (3H, d, J = 6.8 Hz, iPr-CH3), 1.28 (3H, t, J = 8.1Hz, -OCH2CH3), 1.31 (3H, t, J = 7.1Hz, -OCH2CH3), 1.64 (3H, s, 6-Me), 2.10 (3H, s, 6-SCH3), 2.33 (1H, m, -CH(CH3)2), 3.89 (1H, d, J = 3.9 Hz, H-3), 4.10−4.34 (4H, m, 2x -OCH2CH3); 13C-NMR (CDCl3) δ 13.1 (q), 14.2 (q), 14.3 (q), 17.6 (q), 19.7 (q), 28.2 (q), 31.1 (d), 60.9 (d), 61.00 (t), 61.02 (d), 62.7 (s), 162.3 (s), 163.7 (s); HRMS m/z calcd for C13H25N2O2S (M+H)+ 273.1637, found 273.1634.
(3S,6R)-2,5-Diethoxy-3,6-dihydro-6-ethyl-3-isopropyl-6-methylsulfanylpyrazine 5b; pale yellow oil; [α]D25 −78.2 (c 0.11, EtOH); IR (liquid film) νmax 1677 (C=C) cm−1; 1H-NMR (CDCl3) δ 0.66 ( 3H, d, J = 7.0 Hz, iPr-CH3), 1.08 (3H, d, J = 7.0 Hz, iPr-CH3), 1.28 (3H, t, J = 7.1 Hz, -OCH2CH3), 1.30 (3H, t, J = 7.1 Hz, -OCH2CH3), 1.66 (3H, s, 6-Me), 1.88 (3H, s, 6-SCH3), 2.32 (1H, sept d, J = 7.0, 3.2 Hz, -CH(CH3)2), 3.99 (1H, d, J = 3.2 Hz, H-3), 4.10−4.30 (4H, m, 2x -OCH2CH3); 13C-NMR (CDCl3) δ 12.4 (q), 14.2 (q), 14.4 (q), 16.3 (q), 19.2 (q), 27.6 (q), 31.1 (d), 61.0 (t), 61.04 (d), 63.6 (t), 63.6 (s), 161.4 (s), 164.0 (s); HRMS m/z calcd for C13H25N2O2S (M+H)+ 273.1637, found 273.1639.
(3S,6S)-2,5-Diethoxy-6-ethyl-3,6-dihydro-3-isopropyl-6-methylsulfanylpyrazine 6a; oil; [α]D25 +55.4 (c 0.73, EtOH); IR (liquid film) νmax 1681 (C=C) cm−1; 1H-NMR (CDCl3) δ 0.74 (3H, t, J = 7.3 Hz, 6-CH2CH3), 0.78 (3H, d, J = 6.9 Hz, iPr-CH3), 1.10 (3H, d, J = 6.9 Hz, iPr-CH3), 1.29 (3H, t, J = 7.1 Hz, -OCH2CH3), 1.30 (3H, t, J = 7.1 Hz, -OCH2CH3), 1.85 (1H, dq, J = 13.1, 7.3 Hz, 6-CHAHBCH3), 2.07 (3H, s, SCH3), 2.12 (1H, dq, J = 13.1, 7.3 Hz, 6-CHAHBCH3), 2.34 (1H, sept d, J = 6.9, 3.7 Hz, 3-CH(CH3)2), 3.89 (1H, d, J = 3.7 Hz, H-3), 4.12−4.28 (4H, m, -OCH2CH3); 13C-NMR (CDCl3) δ 8.8 (q), 12.7 (q), 14.3 (q), 14.4 (q), 17.5 (q), 19.7 (q), 31.0 (d), 33.1 (t), 60.9 (t), 60.95 (d), 60.96 (t), 66.7 (s), 160.8 (s), 164.4 (s); HRMS m/z calcd for C14H27N2O2S (M+H)+ 287.1794, found 287.1789.
(3S,6S)-2,5-Diethoxy-3,6-dihydro-3,6-diisopropyl-6-methylsulfanylpyrazine 7a: oil; [α]D25 +59.0 (c 0.40, EtOH); IR (liquid film) νmax 1681 (C=C) cm−1; 1H-NMR (CDCl3) δ 0.75 (3H, d, J = 6.9 Hz, 6-iPr-CH3), 0.76 (3H, d, J = 6.9 Hz, 6-iPr-CH3), 1.10 (3H, d, J = 6.9 Hz, 3-iPr-CH3), 1.10 (3H, d, J = 6.9 Hz, 3-iPr-CH3), 1.29 (3H, t, J = 7.1 Hz, -OCH2CH3), 1.31 (3H, t, J = 7.1 Hz, -OCH2CH3), 2.01 (3H, s, -SCH3), 2.32 (1H, sept, J = 6.9 Hz, 6-CH(CH3)2), 2.35 (1H, sept d, J = 6.9, 3.7 Hz, 3-CH(CH3)2), 3.84 (1H, d, J = 3.7 Hz, H-3), 4.11−4.27 (4H, m, -OCH2CH3); 13C-NMR (CDCl3) δ 13.2 (q), 14.3 (q), 14.4 (q), 16.1 (q), 17.5 (q), 17.8 (q), 19.7 (q), 30.8 (d), 35.8 (d), 60.5 (d), 60.87 (t), 60.9 (t), 70.1 (s), 161.3 (s), 164.2 (s); HRMS m/z calcd for C15H29N2O2S (M+H)+, 301.1950, found 301.1950.
*Minor product 6b or 7b could not be isolated due to instability during the purification by silica gel chromatography or preparative TLC.
(3S,6S)-2,5-Diethoxy-3,6-dihydro-3-isopropyl-6-piperonyl-6-methylsulfanylpyrazine 8a: oil; [α]D25 −9.2 (c 0.33, CHCl3); IR (liquid film) νmax 1646 (C=C) cm−1; 1H-NMR (CDCl3) δ 0.68 (3H, d, J = 6.9 Hz, iPr-CH3), 0.97 (3H, d, J = 6.9 Hz, iPr-CH3), 1.29 (3H, t, J = 7.1 Hz, -OCH2CH3), 1.37 (3H, t, J = 7.1 Hz, -OCH2CH3), 2.11 (3H, s, -SCH3), 2.21 (1H, sept d, J = 6.9, 3.7 Hz, -CH(CH3)2), 3.01 (1H, d, J = 12.8 Hz, ArCHH-), 3.20 (1H, d, J = 3.7 Hz, H-3), 3.32 (1H, d, J = 12.8 Hz, ArCHH-), 4.06−4.30 (4H, m, -OCH2CH3), 5.89 (1H, d, J = 1.6 Hz, -OCHHO-), 5.90 (1H, d, J = 1.6 Hz, -OCHHO-), 6.52 (1H, dd, J = 7.8, 1.6 Hz, Ar-H), 6.55 (1H, d, J = 1.6 Hz, Ar-H), 6.62 (1H, d, J = 7.8 Hz, Ar-H); 13C-NMR (CDCl3) δ 12.8 (q), 14.4 (q), 14.5 (q), 17.2 (q), 19.5 (q), 30.6 (d), 45.6 (t), 60.4 (d), 60.9 (t), 61.0 (t), 67.3 (s), 100.7 (t), 107.7 (d), 110.5 (d), 123.3 (d), 129.9 (s), 146.2 (s), 146.9 (s), 159.7 (s), 164.5 (s); HRMS m/z calcd for C20H28N2O4S (M)+ 392.1769, found 392.1765.
Ethanolysis of 6-methylsulfanyl-bislactim ether
General procedure (Scheme 5): Compound 3a (19.3 mg, 0.061 mmol) was dissolved in 0.2 M TFA solution in ethanol (1.22 mL). After stirring for 6 days at rt, the reaction mixture was evaporated directly to afford a crude residue that was purified by preparative TLC (solvent: hexane:EtOAc = 10:1) to give 9 (15.7 mg, 86%).
6-Benzyl-2,5-diethoxy-3-isopropylpyrazine 9: oil; IR (liquid film) νmax 1636 (C=C), 1495 (C=C) cm-1; 1H-NMR (CDCl3) δ 1.18 (6H, d, J = 6.9 Hz, ArCH(CH3)2), 1.33 (3H, t, J = 7.1 Hz, -OCH2CH3), 1.35 (3H, t, J = 7.1 Hz, -OCH2CH3), 3.22 (1H, sept, J = 6.9 Hz, ArCH(CH3)2), 3.99 (2H, s, PhCH2-), 4.30 (2H, q, J = 7.1 Hz, -OCH2CH3), 4.32 (2H, q, J = 7.1 Hz, -OCH2CH3), 7.17 (1H, t, J = 7.1 Hz, Ar-H), 7.25(1H, t, J = 7.1 Hz, Ar-H), 7.35 (1H, t, J = 7.1 Hz, Ar-H); 13C-NMR (CDCl3) δ 14.7 (q), 14.8 (q), 20.7 (q), 28.8 (d), 37.8 (t), 61.7 (t), 61.8 (t), 125.9 (d), 128.1 (d), 129.2 (d), 136.1 (s), 139.5 (s), 143.9 (s), 151.3 (s), 151.9 (s); HRMS m/z calcd for C18H24N2O2 (M)+ 300.1837, found 300.1834.
2,5-Diethoxy-3-isopropyl-6-methylpyrazine 10: oil; IR (liquid film) νmax 1637 (C=C) cm-1; 1H-NMR (CDCl3) δ 1.20 (6H, d, J = 6.9 Hz, ArCH(CH3)2), 1.35 (3H, t, J = 7.1 Hz, -OCH2CH3), 1.36 (3H, t, J = 7.1Hz, -OCH2CH3), 2.32 (3H, s, 6-CH3), 3.25 (1H, sept, J = 6.9Hz, ArCH(CH3)2), 4.30 (2H, q, J = 7.1Hz, -OCH2CH3), 4.34 (2H, q, J = 7.1 Hz, -OCH2CH3); 13C-NMR (CDCl3) δ 14.8 (q), 14.9 (q), 18.0 (q), 20.8 (q), 28.7 (d), 61.7 (t), 61.8 (t), 134.4 (s), 143.0 (s), 151.1 (s), 152.4 (s); HRMS m/z calcd for C12H20N2O2 (M)+ 224.1524, found 224.1523.
2,5-Diethoxy-6-ethyl-3-isopropylpyrazine 11: oil; IR (liquid film) νmax 1635 (C=C) cm-1; 1H-NMR (CDCl3) δ 1.20 (6H, d, J = 6.9 Hz, ArCH(CH3)2), 1.22 (3H, t, J = 7.6 Hz, ArCH2CH3), 1.356 (3H, t, J = 7.1Hz, -OCH2CH3), 1.358 (3H, t, J = 7.1Hz, -OCH2CH3), 2.68 (2H, q, J = 7.6 Hz, ArCH2CH3), 3.25 (1H, sept, J = 6.9Hz, ArCH(CH3)2), 4.33 (2H, q, J = 7.1Hz, -OCH2CH3), 4.34 (2H, q, J = 7.1 Hz, -OCH2CH3); 13C-NMR (CDCl3) δ 11.8 (q), 14.8 (q), 20.8 (q), 24.5 (t), 28.7 (d), 61.6 (t), 61.7 (t), 134.4 (s), 139.0 (s), 142.7 (s), 151.2 (s), 151.9 (s); HRMS m/z calcd for C13H22N2O2 (M)+ 238.1681, found 238.1684.
2,5-Diethoxy-3,6-diisopropylpyrazine 12: oil; IR (liquid film) νmax 1635 (C=C) cm-1; 1H-NMR (CDCl3) δ 1.20 (12H, d, J = 6.9 Hz, ArCH(CH3)2), 1.35 (6H, t, J = 7.1Hz, -OCH2CH3), 3.24 (1H, sept, J = 6.9Hz, ArCH(CH3)2), 4.34 (4H, q, J = 7.1 Hz, -OCH2CH3); 13C-NMR (CDCl3) δ 14.9 (q), 20.9 (q), 28.7 (d), 61.5 (t), 142.4 (s), 151.1 (s); HRMS m/z calcd for C14H24N2O2 (M)+ 252.1837, found 252.1834.
2,5-Diethoxy-3-isopropyl-6-piperonylpyrazine 13: oil; IR (liquid film) νmax 1635 (C=C), 1504 (C=C) cm-1; 1H-NMR (CDCl3) δ 1.18 (6H, d, J = 6.9 Hz, ArCH(CH3)2), 1.34 (3H, t, J = 6.8 Hz, -OCH2CH3), 1.36 (3H, t, J = 6.8 Hz, -OCH2CH3), 3.23 (1H, sept, J = 6.9 Hz, ArCH(CH3)2), 3.89 (2H, s, -CH2Ar), 4.31 (2H, q, J = 6.8 Hz, -OCH2CH3), 4.33 (2H, q, J = 6.8 Hz, -OCH2CH3), 6.73 (1H, d, J = 8.0 Hz, Ar-H), 6.81 (1H, dd, J = 8.0, 1.6 Hz, Ar-H), 6.87 (1H, d, J = 1.6 Hz, Ar-H); 13C-NMR (CDCl3) δ 14.77 (q), 14.84 (q), 20.7 (2x q), 28.8 (d), 37.4 (t), 61.75 (t), 61.78 (t), 100.7 (t), 107.9 (d), 109.7 (d), 122.0 (d), 133.3 (s), 136.1 (s), 144.0 (s), 145.7 (s), 147.3 (s), 151.3 (s), 151.8 (s); HRMS m/z calcd for C19H24N2O4 (M)+ 344.1736, found 344.1739.
ACKNOWLEDGEMENTS
This paper is dedicated to Professor Manabu Node on the occasion of his retirement from Kyoto Pharmaceutical University. We are grateful to Dr. K. Minoura and Ms. M. Fujitake of this University for NMR and MS measurements, respectively. We also thank N. Kita and M. Takakuwa of our laboratory for some experimental supports.
References
1. C. Leigh and A. Taylor, Advances in Chemistry Series, 1976, 149, 228. CrossRef
2. M. R. Bell, J. R. Johnson, B. S. Wildi, and R. B. Woodward, J. Am. Chem. Soc., 1958, 80, 1001. CrossRef
3. F. Dorn and D. Arigoni, Experientia, 1974, 30, 134. CrossRef
4. L. M. N. Synge, E. P. White, and N. Z. J. Agric. Res., 1960, 3, 907.
5. N. Neuss, R. Nagarajan, B. B. Molloy, and L. L. Huckstep, Tetrahedron Lett., 1968, 4467. CrossRef
6. R. Nagarajan, L. L. Huckstep, D. H. Lively, D. C. DeLong, M. M. Marsh, and N. Neuss, J. Am. Chem. Soc., 1968, 90, 2980. CrossRef
7. H. Minato, M. Matsumoto, and T. Katayama, J. Chem. Soc., Perkin Trans. 1, 1973, 1819. CrossRef
8. D. Hauser, H. P. Weber, and H. P. Sigg, Helv. Chim. Acta, 1970, 53, 1061. CrossRef
9. T. Yamada, C. Iwamoto, N. Yamagaki, T. Yamanouchi, Takako, K. Minoura, S. Hagishita, Sanji, and A. Numata, Heterocycles, 2004, 63, 641. CrossRef
10. C. Iwamoto, T. Yamada, Y. Ito, K. Minoura, and A. Numata, Tetrahedron, 2001, 57, 2997. CrossRef
11. Y. Usami, S. Aoki, T. Hara, and A. Numata, J. Antibiot., 2002, 55, 655.
12. Y. Usami, J. Yamaguchi, and A. Numata, Heterocycles, 2004, 63, 1123. CrossRef
13. Y. Kishi, T. Fukuyama, and S. Nakatsuka, J. Am. Chem. Soc., 1973, 95, 6490. CrossRef
14. Y. Kishi, T. Fukuyama, and S. Nakatsuka, J. Am. Chem. Soc., 1973, 95, 6492. CrossRef
15. Y. Kishi, S. Nakatsuka, T. Fukuyama, and M. Havel, J. Am. Chem. Soc., 1973, 95, 6493. CrossRef
16. S. Nakatsuka, T. Fukuyama, and Y. Kishi, Tetrahedron Lett., 1974, 1549. CrossRef
17. Y. Kishi and T. Fukuyama, J. Am. Chem. Soc., 1976, 98, 6723. CrossRef
18. D. L. Coffen, D. A. Katonak, N. R. Nelson, and F. D. Sancilio, J. Org. Chem., 1977, 42, 948. CrossRef
19. Y. Yonezawa, K. Shimizu, M. Uchiyama, N. Kagawa, and C.-G. Shin, Heterocycles, 1997, 45, 1151. CrossRef
20. T. Sato and T. Hino, Chem. Pharm. Bull., 1976, 24, 285.
21. E. Poisel and U. Schmidt, Chem. Ber., 1971, 104, 1714. CrossRef
22. H. Poisel and U. Schmidt, Chem. Ber., 1972, 105, 625. CrossRef
23. E. Ohler, F. Tataruch, and U. Schmidt, Chem. Ber., 1972, 105, 3658. CrossRef
24. E. Ohler, F. Tataruch, and U. Schmidt, Chem. Ber., 1973, 106, 165. CrossRef
25. E. Ohler, H. Poisel, F. Tataruch, and U. Schmidt, Chem. Ber., 1972, 105, 635. CrossRef
26. R. E. Overnan and T. Sato, Org. Lett., 2007, 9, 5267. CrossRef
27. R. Dubey, N. W. Polaske, G. S. Nichol, and B. Olenyuk, Tetrahedron Lett., 2009, 50, 4310. CrossRef
28. J. Kim, J. A. Ashenhurst, and M. Movassaghi, Science, 2009, 324, 238. CrossRef
29. U. Groth, U. Schöllkopf, and Y.-C. Chiang, Angew. Chem., Int. Ed. Engl., 1981, 20, 798. CrossRef
30. U. Schöllkopf, U. Groth, and C. Deng, Synthesis, 1982, 864.
31. Y. Usami, M. Arimoto, H. Ichikawa, K. Kobayashi, M. Honjou, M. Yamanaka, M. Miyao, K. F. Bastow, and K. H. Lee, Heterocycles, 2009, 78, 2041. CrossRef
32. C. Kioka, M. Arimoto, H. Ichikawa, K. Masutani, and Y. Usami, Proceedings of the 22nd International Symposium of the Organic Chemistry of Sulfur (ISOCS-22) Abstract, 2006, p. 76, Saitama, Japan.
33. J. R. Hanson and M. A. O'Leary, J. Chem. Soc., Perkin Trans. 1, 1981, 218.
34. M. Chu, R. Mierzwa, I. Truumees, F. Gentile, M. Patel, V. Gullo, T.-M. Chan, and M. S. Puar, Tetrahedron Lett., 1993, 34, 7537. CrossRef
35. N. Kawahara, K. Nozawa, S. Nakajima, and K. Kawai, J. Chem. Soc., Perkin Trans. 1, 1987, 2099. CrossRef
36. M. S. C. Pedras and C. J. Biesenthal, Phytochemistry, 2001, 58, 905. CrossRef
37. S. Sano, T. Miwa, X.-K. Liu, T. Ishii, T. Takehisa, M. Shiro, and Y. Nagao, Tetrahedron: Asymmetry, 1998, 9, 3615. CrossRef
38. Y. Wang, J. B. Gloer, J. A. Scott, and D. Malloch, J. Nat. Prod., 1995, 58, 93. CrossRef
39. M. Devys, M. Barbier, A. Kollmann, and J.-F. Bousquet, Phytochemistry, 1992, 43, 4393. CrossRef