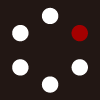
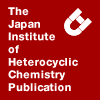
HETEROCYCLES
An International Journal for Reviews and Communications in Heterocyclic ChemistryWeb Edition ISSN: 1881-0942
Published online by The Japan Institute of Heterocyclic Chemistry
e-Journal
Full Text HTML
Received, 15th May, 2010, Accepted, 6th September, 2010, Published online, 7th September, 2010.
DOI: 10.3987/COM-10-S(E)30
■ Nucleophilic Reactions of 9-Isopropyl-2,4-dimethoxy-7,12-dimethyl-3-(phenylsulfonyl)benzo[a]heptalene with Lithium Dialkylamides
Samir El Rayes, Anthony Linden, Khaled Abou-Hadeed, and Hans-Jürgen Hansen*
Institute of Organic Chemistry, University of Zürich, Winterthurerstrasse 190, CH-8057 Zürich, Switzerland
Abstract
Treatment of the title compounds with lithium piperidinide in THF at –5 °C leads to an exchange of the two methoxy groups by piperidino substituents (Scheme 2). On the other hand, lithium diisopropylamide (LDA) in THF at –5 °C induces the formation heptaleno-annulated dibenzothiophenes.INTRODUCTION
Already several years ago, we found by chance a new benzo-annulation reaction of dimethyl heptalene-4,5- and -1,2-dicarboxylates with lithiomethyl sulfones as C1 carrier in the presence of an excess of butyl lithium (BuLi) to yield 2,4-dihydroxy-3-(X-sulfonyl)benzo[a]heptalenes.1 We have improved this new “one-pot access” to benzo[a]heptalenes in the meantime continuously (see reference 2 and literatures cited there), so that colchicine-like compounds of type 1 and 2 became available in yields of > 80% (Scheme 1). The sulfonyl group in 3 can be reductively removed3 and the third MeO group can be introduced by lithiation of C(3), followed by oxidation of the lithio species with air and then O-methylation.4 On the other hand, the sulfonyl substituent of 2 should allow nucleophilic substitution reactions at C(2) and/or C(4) with MeO as a leaving group. In the following part, we report on the reaction of 3a (R = 7-Me, 9-iPr) with lithium dialkylamides.
RESULTS AND DISCUSSION
The reaction of 3a with a 5-fold molar excess of lithium piperidide, generated by the reaction of piperidine with butyllithium (BuLi), took place smoothly at –5 °C in THF. The sole product that we isolated after recrystallization from CH2Cl2/Et2O in excellent yield was the corresponding 2,4-dipiperidino-3-(phenylsulfonyl)benzo[a]heptalene (4a) (Scheme 2). When we repeated the reaction with only one equivalent of lithium piperidide, we found beside non-reacted 3a only the bispiperidino substituted 4a. The reaction of 3a at –40 °C instead of –5 °C gave again only 4a, but the reaction took a longer time. It seems that the entrance of the first piperidino substituent, presumably at C(4), where C- or N-nucleophiles enter preferentially 3-sulfonylbenzo[a]heptalenes 3,5 accelerates appreciably the introduction of the second one.
Normally, lithium diisopropylamide (LDA), also generated by deprotonation of diisopropylamine in THF at –5 °C, is used as sterically hindered base for selective deprotonations. We checked its quality as a nucleophile in the reaction with 3a at –5 °C and got an astonishing result. The two main products that we isolated after chromatography and recrystallization in a pure form and in nearly equal amounts turned out to be the heptaleno-annulated dibenzothiophene dioxides 5a and 6a (Scheme 3).
The structure of both compounds could be derived unequivocally from their spectral data, in particular, their 1H-NMR spectra, in comparison with those of the starting material 3a. We observed in the IR spectra (CHCl3) of 5a and 6a, a shift in the wavenumbers of νas/νs of the SO2 group6 from 1375/1154 cm–1 of the 4-Me analog of 3a to 1306/1159 and 1304/1157 cm–1 of 5a and 6a, respectively, speaking for a change in the bonding situation of the SO2 fragment in going from 3a to 5a and 6a. Full support of the presence of a dibenzothiophene 5,5-dioxide substructure in 5a and 6a came from their 1H-NMR spectra (CDCl3). The general 1H-signal pattern of the phenylsulfonyl group of 3a (d for Ho, t for Hp and Hm in a ratio of 2 : 1 : 2) appeared in the products (5a and 6a) as d, t, t, d pattern in a ratio of 1 : 1 : 1 : 1, typical for non-symmetrically 1,2-substituted benzene derivatives. All further signals and coupling patterns could be derived from COSY and NOESY experiments, including the assignments of the 13C-signals of both new compounds. It was also of importance to note that 5a showed no signals for the presence of a MeO substituent, instead we found the signals of two further, non-equivalent that means diastereotopic with respect to the presence of the axis of chirality of the heptalene part, i-Pr groups. On the other hand, 6a exhibited the signals of one MeO group (1H: δ 4.19; 13C: δ 63.89), which showed, in 1H-NOE experiments, the spatial neighborhood of an H-atom at δ 7.10, appearing as d with 3J = 11.8 Hz, a fact, which was only in accordance with an H-atom linked to a heptalene C=C bond. These findings together with all other NMR data were in perfect agreement with the proposed structures of 5a and 6a. The structure of 5a, crystallized from CDCl3, was finally established by an X-ray crystal-structure analysis (Figure 1), which showed that the asymmetric unit contains one heptalene and one CDCl3 molecule.
The results with LDA demonstrate that LDA acts in a first line as a base that deprotonates the phenylsulfonyl substituent at C(3) of 3a in the ortho-position of the benzene ring. The nucleophile thus formed substitutes intramolecularly the adjacent MeO groups at C(2) as well as at C(4) via Meisenheimer-type intermediates (Scheme 4). The first step in the formation of 5a seems to be the appearance of the intermediate (7a), the MeO group of which is then substituted by LDA. We cannot exclude the possibility that 3a reacts first with LDA, followed by ring closure to 5a. However, the absence of the product derived by further reaction of 6a with LDA under the reaction conditions indicates that the first steps are the ring closure reaction to 6a and 7a, and only 7a carries a sterically less hindered MeO for the subsequent intermolecular substitution reaction with LDA.
Little is known about the formation of dibenzothiophene 5,5-dioxides by ring closure of corresponding diphenylsulfone precursors. The main procedures for the synthesis of dibenzothiophene 5,5-dioxides utilize the formation of dibenzothiophenes, which are then oxidized, for example, with 30% aqueous H2O2 in glacial acetic acid to the corresponding 5,5-dioxides (see, e.g.7). Nevertheless, Pol and Kulkarni reported on the synthesis of 2,3,7,8-tetramethoxy-dibenzothiophene 5,5-dioxide by heating (2-bromo-3',4,4',5-tetramethoxy)diphenyl sulfone in the presence of EtONa in Ph2O,8 which follows obviously a similar mechanism as discussed above for 3a with LDA as the base and MeO– as the leaving group. Lithiation in the ortho-position of diphenyl sulfone dioxide with BuLi in THF/Et2O at –60 °C and then heating at 45 °C has also been used for the synthesis of dibenzothiophene (72%), whereby formed LiH seems to be responsible for the reduction of the primarily generated dibenzothiophene 5,5-dioxide.9
In conclusion, we can say that the ortho-deprotonated (lithiated) diphenyl sulfones with the ortho-MeO group(s) at the second phenyl group react smoothly to the corresponding dibenzothiophene 5,5-dioxides, whereby LDA as the sterically hindered reactant acts primarily as the strong base and not as the nucleophile, thereby avoiding intermolecular nucleophilic substitution reactions with the ortho-MeO group(s).
EXPERIMENTAL
General: THF was purified and dried by reflux over sodium benzophenone and was distilled just before use. All reactions were performed under an atmosphere of Ar. Melting points (mp) were measured on a Büchi FP5 apparatus. They are not corrected. TLC on aluminum sheets coated with silica gel 60 F254 (Merck). Column chromatography (CC) was performed on silica gel 60 (40-63 µm; Chemie Uetikon AG). IR spectra were recorded on a Perkin Elmer 1600 FT-IR spectrometer. Band positions are given in wavenumbers (cm–1). Transmissions are classified as vs = very strong (< 10%), s = strong (10-30%), m = middle (30-50%), and w = weak (> 60%). 1H-NMR and 13C-NMR spectra (CDCl3) were measured at 300 K on a Bruker 600 AMX spectrometer; δ in ppm related to internal TMS (= 0 ppm) and adjusted to the solvent signals 7.26 ppm and 77.00 ppm, respectively, J in Hz. Assignments of the signals are based on additional DEPT 90, DEPT 135, COSY, NOSEY, NOE, HSQC, HMBC, and TOCSY measurements. Mass spectra (MS) were measured on a Finnigan MAT 95 instrument; chemical ionisation (CI) with NH3, 70 eV, at a temp. of 250 °C.
Reaction of 2,4-Dimethoxy-3-phenylsulfonylbenzo[a]heptalene (3) with Lithium Piperidide. – 9-Isopropyl-7,12-dimethyl-2,4-dipiperidino-3-(phenylsulfonyl)benzo[a]heptalene (4a). To a solution of piperidine (0.628 ml, 7 mmol) in THF (5 mL), 2.5M BuLi (2.84 mL, 7.1 mmol) were slowly added at –5°. The yellow solution was stirred for 30 min. This solution was added by using a cannula to a solution of 3a (0.50 g, 1.05 mmol) in THF (15 mL) at –5 °C. The reaction mixture was stirred for 15 min at –5 °C. Then, the mixture was treated with ice/4N HCl. The product was extracted with AcOEt. The organic phase was washed with brine and dried (Na2SO4). The solvent was distilled off in a rotatory evaporator (RE). The residue was purified by CC (silica gel; hexane/AcOEt 2 : 1) and crystallized from CH2Cl2/Et2O to yield 0.55 g (89%) of pure 4a.
Data of 4a: Yellow crystals, mp 214.2–214.6 °C. Rf (hexane/AcOEt 3 : 1) 0.69. IR (KBr): 3007m, 2926vs, 2853s, 1551vs, 1521s, 1464m, 1444vs, 1418s, 1381s, 1302vs, 1290s, 1275s, 1248m, 1213s, 1182m, 1140vs, 1123m, 1090s, 1074m, 1032m, 990s, 914w, 887w, 857m, 750s, 687s, 617s, 608vs, 562s. 1H-NMR: δ 7.52 (d, 3J = 7.4, Ho of Ph); 7.35 (t-like, Hp of Ph); 7.40 (t-like, Hm of Ph); 7.06 (d, 3J(5,6) = 11.9, H–C(5)); 6.40 (d, 3J(11,10) = 11.9, H–C(11)); 6.36 (dd, 3J(10,11) = 11.9, 4J(10,8) = 0.9, H–C(10)); 6.25 (d, 3J(6,5) = 11.8, H–C(6)); 5.73 (s, H-C(8)); 3.47, 2.36, 2.16, 1.60, 1.21 (H of two piperidino groups); 2.56 (sept, Me2CH–C(9)); 1.74 (s, Me–C(12)); 1.72 (s, Me–C(7)); 1.16, 1.15 (2d, 3J = 7.9 and 7.8, Me2CH–C(9)). 13C-NMR: δ 154.77 (s, C(2)); 154.35 (s, C(4)); 147.86 (s, Cip of Ph); 146.85 (s, C(9)); 144.91 (s, C(12b)); 135.74 (d, C(11)); 135.43 (s, C(12a)); 133.43 (s, C(7a)); 131.40 (d, C(10)); 131.12 (s, C(12)); 131.01 (d, C(6)); 130.74 (d, Cp of Ph); 129.81 (s, C(4a)); 128.90 (d, C(5)); 128.80 (s, C(7)); 127.94 (d, Cm of Ph); 125.19 (d, Co of Ph); 124.39 (s, C(3)); 122.20 (d, C(8)); 115.17 (d, C(1)); 58.73/50.64 (C of piperidino groups); 34.44 (d, Me2CH–C(9)); 25.28/24.45/24.35/23.83 (C of piperidino groups); 22.78/22.82 (2q, Me2CH–C(9)); 20.41 (q, Me–C(12)); 16.60 (q, Me–C(7)). CI-MS (C37H44N2O2S; 580.84): 584.5 (6, [M + 3]+); 583.5 (20, [M + 2]+); 582.5 (42, [M + 1]+); 581.5 (100, [M]+); 442.5 (17); 441.5 (50); 439.5 (21); 388.4 (7).
Reactions of 9-Isopropyl-2,4-dimethoxy-7,12-dimethyl-3-(phenylsulfonyl)benzo[a]heptalene (3a). 3,8-Dimethyl-N,N,5-tri(propan-2-yl)benzo[b]heptaleno[1’,2’:5,6]benzo[1,2-d]thiophen-10-amine 11, 11-dioxide (5a) and 9-Methoxy-1,6-dimethyl-4-(propan-2-yl)benzo[b]heptaleno[2’,1’:4,5]- benzo[1,2-d]thiophene 10,10-dioxide (6a). A solution of LDA (7.35 mmol) in THF (5 mL) was produced at –5 °C in the usual manner (see above). It was added slowly via a cannula to a solution of 3a (0.50 g, 1.05 mmol) in THF (15 mL). After 1 h stirring, the mixture was treated with chopped ice/4N HCl. The products were extracted with AcOEt and then, the organic layer was washed with brine and dried (Na2SO4). The solvent was distilled off (RE). The residue was subjected to CC (hexane/AcOEt 3 : 1) to yield in a first fraction 5a (0.20 g, 37%) and then 6a (0.13 g, 29%).
Data of 5a: Orange crystals from CDCl3, mp 160.6–162.8 °C. Rf: 0.49 (hexane/AcOEt 3 : 1). IR (CHCl3): 2964m, 2916w, 1569m, 1459m, 1306vs, 1220m, 1159vs, 1132m, 1060m, 1030w. 1H-NMR: δ 8.01 (d, Jo= 8.0, H–(15)); 7.82 (dd, Jo = 7.7, Jm = 0.7, H–C(12)); 7.59 (td, Jo = 7.7, Jm = 1.2, H–C(14)); 7.50 (t, Jo = 7.5, H–C(13)); 7.30 (d, 3J(1,2) = 11.9, H–C(1)); 6.92 (s, H–C(9)); 6.51 (d, 3J(2,1) = 11.9, H-C(2)); 6.44 (d, 3J(7,6) = 12.0, H–C(7)); 6.43 (dd, 3J(6,7) = 12.1, 4J(6,4) = 1.1, H–C(6)); 5.79 (s, H–C(4)); 3.61 (sept, (Me2CH)2N–C(10)); 2.59 (sept, Me2CH–C(5)); 1.76 (s, Me–C(8)); 1.74 (s, Me–C(3)); 1.17, 1.18 (4d, 3J = 6.5, (Me2CH)2N–C(10) and 2d, 3J = 7.1, Me2CH–C(5) ). 13C-NMR: δ 148.23 (s, C(10)); 147.44 (s, C(8b)); 147.31 (s, C(5)); 139.65 (s, C (11a); 136.88 (s, C(10a)); 135.95 (s, C(3a)); 135.25 (d, C(2)); 135.11 (d, C(7)); 134.36 (s, C(8a)); 133.04 (d, C(14)); 131.96 (s, C(15c)); 131.85 (d, C(6)); 131.79 (d, C(9)); 131.55 (s, C (15a)); 131.37 (s, C(8)); 130.18 (s, C(15b); 129.46 (d, C(13)); 128.50 (d, C(1)); 128.00 (s, C(3)); 125.96 (d, C(15)); 121.94 (d, C(12)); 121.68 (d, C(4)); 50.72 (d, (Me2CH)2N–C(10)); 34.51 (d, Me2CH–C(5)); 22.76, 22.87 (2q, Me2CH–C(4)); 21.44, 21.56 (2q, (Me2CH)2N–C(10)); 19.97 (q, Me–C(8)); 16.40 (q, Me–C(3)). EI-MS (C33H37NO2S; 511.73): 512.1 (10, [M + 1]+); 511.1 (26, [M]+); 497.1 (34, [(M + 1) – Me]+); 496.0 (97, [M – Me]+); 469.0 (6); 456.0 (11); 454.0 (100, [M – iPrN]+); 288.9 (12); 275.9 (7); 227.1 (11); 192.5 (9).
Data of 6a: Orange crystals from Et2O/hexane, mp 244.4–246.7°. Rf: 0.45 (hexane/AcOEt 3 : 1). IR (CHCl3): 2959m, 2923m, 1592m, 1551m, 1470m, 1446m, 1373s, 1303vs, 1263s, 1239m, 1165m, 1157vs, 1128s, 1057m, 1001m, 736vs, 607s, 568s, 548m. 1H-NMR: δ 7.80 (d, Jo = 7.6, H–C(11)); 7.74 (d, Jo = 7.7, H–C(14)); 7.59 (t, Jo = 7.7 and 7.4, H–C(13)); 7.50 (t, Jo = 7.6 and 7.5, H–C(12)); 7.099 (s, H–C(15)); 7.097 (d, 3J(8,7) = 11.8, H–C(8)); 6.44 (m, H–C(2,3,7)); 5.78 (s, H–C(5)); 4.19 (s, MeO–C(9)); 2.59 (sept, Me2CH–C(4)); 1.74 (s, Me–C(6)); 1.68 (s, Me–C(1)); 1.17, 1.18 (2d, J = 7.1, Me2CH–C(4)). 13C-NMR: δ 154.70 (s, C(9)); 147.79 (s, C(4)); 144.53 (s, C(15a)); 138.32 (s, C(10a)); 135.56 (d, C(2)); 135.44 (d, C(7)); 134.31 (s, C(5a)); 133.75 (s, C(14b)); 133.59 (d, C(13) and s, C(15b)); 133.56 (s, C(8a)); 132.25 (s, C(1)); 132.17 (d, C(3)); 131.34 (s, C(14a)); 130.15 (d, C(12); 128.71 (s, C(6)); 127.45 (s, C(9a)); 124.61 (d, C(8)); 122.45 (d, C(11)); 122.12 (d, C(5)); 121.46 (d, C(14)); 117.33 (d, C(15)); 63.89 (q, MeO–C(9)); 34.57 (d, Me2CH–C(4)); 22.78 (q, Me2CH–C(4)); 19.36 (q, Me–C(1)); 16.99 (q, Me–C(6)). EI-MS (C28H26O3S, 442.58): 443 (31, [M + 1]+); 442 (100, [M]+) 427 (38, [M – Me]+); 402 (47, [M – MeC≡CH]+); 387 (25); 374 (18); 305 (14); 289 (17); 276 (16); 200 (17).
2. X-ray crystal structure determination of 5a·CDCl3. Crystal data: Crystals obtained from CDCl3, C33H37NO2·CDCl3, M = 631.10, monoclinic, space group: P21/c, a = 9.9303(1) Å, b = 13.6485(2) Å, c = 23.3724(3) Å, β = 98.1576(4)°, V = 3135.70(7) Å3, Z = 4, Dx = 1.337 g cm-3, 2θmax = 55°, T = –113 °C, crystal dimensions: 0.07 × 0.15 × 0.20 mm, Nonius KappaCCD area-detector diffractometer, Mo Kα radiation, λ = 0.71073 Å, µ(Mo Kα) = 0.391 mm-1, 71199 measured reflections, 7173 independent reflections, 4715 reflections with I > 2σ(I), refinement on F with teXsan,10 370 parameters, R(F) [I > 2s(I) reflections] = 0.0504, wR(F2) [I > 2σ(I) reflections] = 0.0535, goodness of fit = 1.986, Δρmax = 0.66 e Å-3. The asymmetric unit contains one molecule of 5a and one molecule of deuteriochloroform. The non-hydrogen atoms were refined anisotropically. All of the H-atoms were placed in geometrically calculated positions.
CCDC-775867 contains the supplementary crystallographic data for this compound. These data can be obtained free of charge from The Cambridge Crystallographic Data Centre via www.ccdc.cam.ac.uk/data_request/cif.
ACKNOWLEDGEMENTS
Support of this work by the Swiss National Science Foundation is gratefully acknowledged.
References
1. K. Abou-Hadeed and H.-J. Hansen, Helv. Chim. Acta, 1997, 80, 2535. CrossRef
2. K. Abou-Hadeed and H.-J. Hansen, ‘Science of Synthesis’, Vol. 45b, Georg Thieme Verlag KG, Stuttgart, New York, 2010, p. 1043.
3. Mohy eldin Abdel Fattah, S. El Rayes, El Sayed Ahmed Soliman, A. Linden, K. Abou-Hadeed, and H.-J. Hansen, Helv. Chim. Acta, 2005, 88, 1085. CrossRef
4. S. El Rayes, K. Abou-Hadeed, and H.-J. Hansen, Chimia, 2001, 55, 621.
5. S. El Rayes, A. Linden, K. Abou-Hadeed, and H.-J. Hansen, Helv. Chim. Acta, 2010, 93, submitted.
6. D. Lin-Vien, N. B. Colthup, W. G. Fateley, and J. G. Graselli, ‘The Handbook of Infrared and Raman Characteristic Frequencies of Organic Molecules’, Academic Press, Inc., San Diego 1991, p. 244.
7. H. Gilman and G. R. Wilder, J. Org. Chem., 1957, 22, 523. CrossRef
8. V. A. Pol and A. B. Kulkarni, Indian J. Chem., 1971, 9, 728.
9. M. C. Briñon, M. M. De Bertorello, and H. E. Bertorello, Anal. Asoc. Quim. Argent., 1983, 71, 571.
10. teXsan: Single Crystal Structure Analysis Software, Version 1.10, ‘Molecular Structure Corporation’, The Woodlands, Texas, 1999.