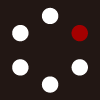
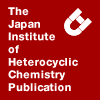
HETEROCYCLES
An International Journal for Reviews and Communications in Heterocyclic ChemistryWeb Edition ISSN: 1881-0942
Published online by The Japan Institute of Heterocyclic Chemistry
e-Journal
Full Text HTML
Received, 17th January, 2011, Accepted, 14th March, 2011, Published online, 25th March, 2011.
DOI: 10.3987/COM-11-12141
■ Synthesis, Theoretical Study, and Antimicrobial Activity of Novel Polysubstituted Thiazoles
Nadia Hanafy Metwally* and Sabry El-Taher
Department of Chemistry, Faculty of Science, Cairo University, Giza 12613, Egypt
Abstract
New 5-(9H-Fluoren-9-ylidene)- and 5-(1H-indan-1-ylidene)-2-thioxo-4-thiazolidinones 3a,b were synthesized Eco-friendly under microwave irradiation (MWI). These compounds undergo alkaline hydrolysis to afford the corresponding thiol derivatives 4a,b, which cyclized into polysubstituted thiazoles 6a-h using arylazomalononitriles 5a-d as nucleophilic agents. Some selected products were tested for their antimicrobial activities. The equilibrium geometries of the studied compounds were calculated at the B3LYP/6-31G(d) level of the density functional theory (DFT). Conformational analysis has been carried out to determine the most stable conformers. The relative stability of the azo and hydrazo tautomers has been investigated. The HOMO-LUMO energy gap is used in rationalizing the reactivity of the studied compounds.INTRODUCTION
Over the past years, 4-thiazolidinone derivatives are powerful class of biological active substances as potential drugs.1-7 The 2-thioxo-4-thiazolidinone (rhodanine) derivative-epalrestat is a highly active aldose reductase inhibitor, which possess a perspective for the treatment of diabetic complications.8-10 The 5-arylidene rhodanine derivatives are known as antibacterial compounds due to inhibition of bacterial cell wall peptidoglycan system.11-13 In view of the above wide applications of 4-thiazolidinone derivatives and in continuation of our previous studies directed towards the synthesis of new heterocyclic compounds of biological potentialities,14-20 we utilized here the microwave irradiation (MWI) as a rapid, safe, and Eco-friendly method, particularly, solvent-free reactions which provides an easy opportunity to scale-up the reaction21-23 to promote the solvent-free Knoevenagel condensation of C-5 active methylene of 2-thioxo-4-thiazolidinone (1) for the synthesis of new 5-(9H-fluoren-9-ylidene) and 5-(1H-inden-1-ylidene)-2-thioxo-4-thiazolidinones which undergo alkaline hydrolysis to afford polysubstituted thiazoles, hoping that they will find applications as new biologically active substances.
RESULTS AND DISCUSSION
The equimolar amounts of 2-thioxo-4-thiazolidinone (1) and 9-fluorenone (2a) or 1-indanone (2b) undergo Knoevenegel condensation under MWI for 1 min. to form highly colored solids (yield ∼95%). In contrast, conventional liquid-phase Knoevenagel reaction took much longer time with low yield. The IR spectrum of the isolated products 3a, as a typical example of the prepared compounds, showed absorption bands at νmax 3152 and 1686 cm-1, corresponding to NH and CO groups, respectively. The 1H NMR spectrum of the isolated product revealed a multiplet signals at the range δ = 7.17-7.68 corresponding to aromatic protons. In addition, a D2O exchangeable signal at δ = 9.05 attributed to the NH proton. Structures 3a,b were assigned to these products respectively, on the basis of their analytical and spectral data (Scheme 1).
Alkaline hydrolysis of the compounds 3a,b under MWI leads to the corresponding aryl-2-sulfanylacrylic acids 4a,b, which exist in two tautomeric forms. The IR spectrum of the hydrolyzed products 4b showed absorption band at 2619 (SH), 1704 (CO), 1020 (SH). 1H NMR spectrum revealed a singlet at δ 3.40 corresponding to the thiol proton and a broad band at δ 11.20 attributed to hydroxyl group of carboxylic acid, besides aromatic proton signals. Elemental analysis and mass spectra confirmed the structures 4a,b (Scheme 2). It is well known that the aryl-2-sulfanylacrylic acids are very important class of compounds due to their reactivity towards a variety of simple reagents to synthesize many products as α-thio acids24 and thiazoles.25-27 We reported here a simple method for the synthesis of novel substituted thiazoles of expected biological activity via the reaction of equimolar amounts of compounds 4a,b and α-aryl hydrazonomalononitriles 5a-d in acetic acid under MWI to afford the highly colored products in excellent yields (Scheme 2). The IR spectra of the isolated products in each case of 6a-h showed a broad absorption bands near νmax 3165-3185 and a strong band near νmax 1687-1690 cm-1. Such bands are assignable to intramolecular hydrogen bonded NH stretch and α,β-unsaturated lactam carbonyl group. The 1H NMR spectrum of 6d revealed a singlet at δ = 9.15 (exchangeable, D2O) assigned to the NH group. Furthermore, the 13C NMR spectrum of compound 6d revealed a signal at δ = 76.12 for C-6 and a characteristic signal at δ = 177.98 assigned for a lactam carbonyl carbon (C-4), beside the other signals as expected (see Scheme 2). The foregoing spectral data, when taken collectively, indicate that the synthesized compounds 6a-h have hydrazo structures 6A in solid (IR) and in solution (1H NMR and 13C NMR) which are stabilized by the hydrogen bonding interaction. The formation of 6 from the reaction of 4 with 5 is assumed to proceed via addition of thiol group in 4 to one of the cyano group in 5 affording an acyclic intermediate which then undergoes cyclization with elimination of water to afford the final isolable products 6a-h (Scheme 2).
ANTIMICROBIAL ACTIVITY
The compounds 6a, 6c, 6d, 6h, 6g were tested for their antimicrobial activities using two fungal species, namely Aspergillus flavus AF and Candida albicans CA as well as two bacteria species, Escherichia coli EC (Gram negative) and Staphylococcus aureus SA (Gram positive). A solution of each compound at a concentration of 20 mg/mL was prepared and the inhibition zone diameter in centimeter (IZD) was used as the criterion for antimicrobial activity. The fungicide amphotericin B and the bactericide tetracycline were used as references to evaluate the potency of the tested compounds under the same conditions. The results are given in Table 1. The results revealed that all compounds exhibited considerable inhibitory action against EC and SA. On the contrary, the compounds exhibited no antifungal activity.
THEORETICAL CALCULATION
The geometrical structures of compounds 6a-h have been fully optimized at the B3LYP/6-31G(d) level of DFT theory. The optimized geometries of the lowest energy conformations of compounds 6a and 6e and the corresponding numbering systems are depicted in Figure 1. Careful inspection of these geometries shows that the tricyclic 9H-fluoren-9-ylidene moiety (6a) as well as the bicyclic 1H-indan-1-ylidene moiety (6e) are coplanar with the thiazolidinone and the arylhydrazononitrile moieties. This coplanarity may be due to the extensive π-conjugation interaction throughout the whole molecule.
Figure 2 shows the energy barrier for rotation around the C1–C18 bond, with a change in the dihedral angle, N19=C18–C1=N2, from 0º to 180º. Two energy minima (at φ = 0º and φ = 150º) and one energy maximum (φ =100º) conformers have been detected during the course of rotation. It is important to note that the two energy minimum conformers are separated by a relatively high energy barrier of about 70 kJ/mol as compared to a free rotation around a C–C single bond. This may be explained in terms of the development of some double bond character due to the resonance between the azo/hydrazo forms. An alternative explanation based on delocalization of n- and π-electrons over the σ framework of the double bond character due to the resonance between the azo/hydrazo forms. An alternative explanation based on delocalization of n- and π-electrons over the σ framework of the molecule may be considered. This correlates well with the shortening of the C1–C18 bond (1.447 Å) as compared to the C–C single bond of ethane (1.536 Å) calculated at the same B3LYP/6-31G(d) level. It is worth noting that the energy minimum conformer at φ = 0º is much lower in energy than the conformer at φ = 150º by about 62 kJ/mol. This stabilization may be due to the intramolecular hydrogen bonding between the hydrogen atom of the hydrazo group and the nitrogen atom of the thiazolidinone moiety (H4···N19 = 1.861 Å).
In addition, the extensive n, π-delocalization in the N19=C18C1=N2-N3-H4 entity significantly contributes to the stability of this conformer. The theoretical tautomeric structures of compounds 6a-h are shown in Scheme 2. The keto-hydrazo tautomers (6A) are found to be more stable significantly contributes to the stability of this conformer. The theoretical tautomeric structures of compounds 6a-h are shown in Scheme 2. The keto- hydrazo tautomers (6A) is found to be more stable than the enol-azo form (6C) by about 25.2 kcal.mol-1, calculated at B3LYP/6-31G(d) level. This is in agreement with the absence of typical signals of OH group in both IR and 1H NMR spectra. For the azo-hydrazo tautomeric equilibrium, it is worth noting that the hydrazo tautomer (6A) is lower in energy than the azo tautomer (6B) by 2.77 kcal.mol-1. In addition, the energy difference between the HOMO (highest occupied molecular orbital) and the LUMO (lowest unoccupied molecular orbital) has been shown to be useful in rationalizing the relative stability and reactivity of different species.28 Species having large HOMO-LUMO energy gap will be more stable and less reactive than those having small HOMO-LUMO energy gap. The hydrazo tautomer has HOMO-LUMO gap of 2.9 eV which is slightly higher by 0.2 eV than that of the azo tautomer. This means that the hydrazo-tautomer is more stable than the azo form. The Gibbs free energy difference between the hydrazo and azo tautomers (ΔG° = G° azo – G° hydraz) is 2.28 kcal.mol-1. The abundance of each tautomer is determined by substituting the calculated value of ΔG° in the Boltzmann equation at 298 K. The estimated population of hydrazo tautomer is found to be 97.9%. This indicates that the compounds 6a-h are presumed to mainly exist in the hydrazo form. This is in agreement with the analysis of IR and 1H NMR spectral data.
The energy barrier for rotation of the bicyclic 1H-indian-1-ylidene moiety in compounds 6e-h around the C23=C24 double bond is shown in Figure 3. Compounds 6e-h may exist as Z or E geometrical isomers. The two conformers are separated by a significant high energy barrier of 131.8 kJ.mol-1, which means that only one of them can exist at room temperature. The Z conformer (φ = 0º) is found to be lower in energy than the E conformer (φ = 180º) by 1.71 kcal.mol-1. The Gibbs free energy difference (ΔG°) between Z and E conformers calculated at 298 K is 1.16 kcal.mol-1. The substitution of the value of ΔG° in the Boltzmann equation results in an estimated population of Z conformer of 87.6%. This means that compound 6e-h mainly exist as Z conformers at room temperature. The isosurface plots of the HOMO and LUMO of compounds 6a and 6e are representatively depicted in Figure 4. In addition, the percent contributions of the different moieties to the corresponding HOMOs and LUMOs of 6a-h are given in Table 2. Careful inspection reveals that about 48% (47%) and 40% (36%) of the HOMO of 6a (6e) are localized on the arylhydrazononitrile and fluoren-9H-ylidene (indan-1H-ylidene), respectively, while only 13% (17%) is localized on the oxothiazole moiety. This means that the HOMOs of these compoundslocalized on the arylhydrazononitrile and fluoren-9H-ylidene (indan-1H-ylidene), respectively, while only 13% (17%) is localized on the oxothiazole moiety.
This means that the HOMOs of these compounds have mainly π conjugate systems, and hence may interact with the LUMO of the biological receptor via π-π interaction. On the other hand, the hydrazo and oxothiazole moieties contribute by about 57% (68%) to the LUMO of 6a (6e). This, in turn, suggests the participation of the LUMOs of these compounds via hydrogen bonding interactions with the HOMO of the receptor. In addition, the HOMO-LUMO energy gaps are relatively low (2.7-3.1 eV) as compared to that of tetracycline (3.98 eV) at calculated at B3LYP/6-31G(d) level suggesting that compounds 6a-h may show relatively high inhibitory effect on both gram-positive and gram-negative bacteria ( Table 1).
COMPUTATIONAL METHOD
Molecular orbital calculations were carried out using the Gaussian 98W package.29 An extensive search for lower energy conformations on the potential energy surface (PES) of compounds 6a and 6e was carried out using Becke’s hybrid Hartree-Fock (HF) density functional method30 with the Lee-Yang-Parr (LYP) correlation functional31 and the 6-31G(d) basis set [B3LYP/6-31G(d)]. Geometries of the studied 4-thiazolidinone derivatives were fully optimized and vibrational frequency calculations were performed.
EXPERIMENTAL
Melting points were determined on an Electrothermal (9100) apparatus and are uncorrected. The IR spectra were recorded as KBr pellets on a Perkin Elmer 1430 spectrophotometer. 1H NMR spectra were recorded in DMSO-d6 using a Varian Gemini NMR spectrometer, and TMS as internal reference. Mass spectra were taken on a Shimadzu GCMS-QP 1000 Ex mass spectrometer at 70 eV. The MW oven was operated at reduced MW power level of 60%. Elemental analyses were carried out by the Microanalysis Center of Cairo University, Giza, Egypt.
General procedure for solvent-free Knoevanagel condensation under microwave-irradiation conditions. Equal molar equivalents of 1 (1.33 g, 0.01 mol), 2a or 2b (0.01 mol) and NH4OAc (0.07g, 0.01 mol) were mixed in a mortar. The reaction mixture was transferred to a Pyrex beaker and subjected to microwave irradiation oven for 1 min. The cooled reaction mixture was poured onto water and filtered off to afford the colored solids 3a,b which crystallized from EtOH/dioxane.
5-(9H-Fluoren-9-ylidene)-2-thioxo-4-thiazolidinone (3a). Orange crystals, yield 98%; mp 278 oC; IR (νmax/cm-1): 3152 (NH), 1686 (C=O). 1H NMR (DMSO) δH 7.17-7.68 (m, 8H, Ar), 9.05 (s, 1H, NH). Anal. Calcd for C16H9NOS2 (295): C, 65.06; H, 3.07; N, 4.74; S, 21.71. Found: C, 65.30; H. 3.25; N, 4.50; S, 21.43%.
5-(1H-Indan-1-ylidene)-2-thioxo-4-thiazolidinones (3b). Yellow crystals, yield 92%; mp 258 oC; IR (νmax/cm-1): 3154 (NH), 1687 (C=O). 1H NMR (DMSO) δH 3.0 (m, 6H, 2CH2), 7.05-7.28 (m, 4H, Ar), 9.10 (s, 1H, NH). Anal. Calcd for C12H7NOS2 (245): C, 58.75; H, 2.28; N, 5.71; S, 26.14. Found: C, 58.48; H. 3.05; N, 5.46; S, 26.28%.
General procedure for alkaline hydrolysis of 3a,b. 0.01 mol of 3a or 3b dissolve in 10 mL 10% of NaOH. The solution was transferred to a pyrex beaker and subjected to microwave irradiation in a domestic microwave oven for 4 min. The cooled reaction mixture was acidified by ice-cold HCl; the solid, so formed, was collected by filtration and washed with water.
2-(9H-Fluoren-9-ylidene)-2-mercaptoacetic acid (4a). Yellow crystals; yield 68%; mp 119 oC (toluene); IR (νmax/cm-1): 2595 (SH), 1698 (C=O), 1456, 1400, (O-CO), 1015 (SH), 754 (C-S). 1H NMR (DMSO) δH 3.35 (br., 1H, SH), 7.12-7.53 (m, 8H, Ar), 11.12 (br., 1H, OH). Anal. Calcd for C15H10O2S (254): C, 70.84; H, 3.96; S, 12.61. Found: C, 71.03; H, 3.75; S, 12.47%.
2-(1H-Indan-1-ylidene)-2-mercaptoacetic acid (4b). Orange crystals; yield 63%; mp 110 oC (toluene); IR (νmax/cm-1): 2619 (SH), 1704 (C=O), 1458, 1405, (O-CO), 1020 (SH), 760 (C-S). 1H NMR (DMSO) δH 2.95 (m, 6H, 2CH2), 3.40 (br., 1H, SH), 7.02-7.23 (m, 4H, Ar), 11.20 (br., 1H, OH). Anal. Calcd for C11H8O2S (204): C, 64.69; H, 3.95; S, 15.70. Found: C, 64.47; H, 3.75; S, 15.55%.
General procedure for synthesis of 5-(arylidene-4-oxothiazolidin-2-ylidene)(arylazo)acetonitriles 6a-h. Equal molar equivalents of 4a,b (0.01 mol) and α-arylhydrazononitriles 5a-d (0.01 mol) in 3 mL acetic acid. The reaction mixture was transferred to a Pyrex beaker and subjected to microwave irradiation oven for 2 min, then allowed to cool. The solid products, so formed, were collected by filtration and crystallized from proper solvent.
5-(9H-Fluoren-9-ylidene)-4-oxothiazolidin-2-ylidene-2-phenylhydrazoacetonitrile (6a). Reddish brown crystals, yield 87%; mp 265 oC (AcOH); IR (νmax/cm-1): 3180 (NH), 2216 (CN), 1686 (C=O). 1H NMR (DMSO) δH 7.05-7.55 (m, 13H, Ar), 9.15 (br., 1H, NH). Anal. Calcd for C24H14N4OS (406): C, 70.92; H, 3.47; N, 13.78, S, 7.89. Found: C, 70.68; H, 3.25; N, 13.55; S, 7.65%.
5-(9H-Fluoren-9-ylidene)-4-oxothiazolidin-2-ylidene-2-(4-methylphenylhydrazo)acetonitrile (6b). Brown crystals; yield (79%); mp 243 oC (AcOH); IR (νmax/cm-1): 3168 (NH), 2219 (CN), 1687 (C=O). 1H NMR (DMSO) δH 3.30 (s, 3H, CH3), 7.08-7.86 (m, 12H, Ar), 9.05 (br., 1H, NH). Anal. Calcd for C25H16N4OS (420): C, 71.41; H, 3.84; N, 13.32, S, 7.63. Found: C, 71.24; H, 3.68; N, 13.50; S, 7.41%.
2-(4-Chlorophenylhydrazo)-5(9H-fluoren-9-ylidene)-4-oxothiazolidin-2-ylideneacetonitrile (6c). Violet crystals; yield (90%); mp 285 oC (AcOH); IR (νmax/cm-1): 3187 (NH), 2221 (CN), 1690 (C=O). 1H NMR (DMSO) δH 7.12-8.13 (m, 12H, Ar), 9.33 (br., 1H, NH). Anal. Calcd for C24H13ClN4OS (440): C, 65.38; H, 2.97; Cl, 8.04; N, 12.71, S, 7.27. Found: C, 65.60; H, 2.75; N, 12.50; S, 7.50%.
5-(9H-Fluoren-9-ylidene)-4-oxothiazolidin-2-ylidene-2-(4-methoxyphenylhydrazo)acetonitrile (6d). Red crystals; yield (86%); mp 275 oC (AcOH); IR (νmax/cm-1): 3185 (NH), 2214 (CN), 1668 (C=O). 1H NMR (DMSO) δH 3.78 (s, 3H, OCH3), 7.11-7.97 (m, 12H, Ar), 9.15 (br., 1H, NH). Anal. Calcd for C25H16N4OS (436): C, 68.79; H, 3.69; N, 12.84, S, 7.35. Found: C, 68.55; H, 3.48; N, 12.620; S, 7.56%.
5-(1H-Indan-1-ylidene)-4-oxothiazolidin-2-ylidene-phenylhydrazoacetonitrile (5e). Brown crystals; yield (81%); mp 235 oC (EtOH/dioxane); IR (νmax/cm-1): 3183 (NH), 2218 (CN), 1687 (C=O); 1H NMR (DMSO) δH 3.10 (m, 6H, 2CH2), 7.05-7.75 (m, 9H, Ar), 9.13 (br., 1H, NH). Anal. Calcd for C20H12N4OS (356) C, 67.40; H, 3.39; N, 15.72, S, 9.0. Found: C, 67.18; H, 3.52; N, 15.50; S, 9.21%.
5-(1H-Indan-1-ylidene)-4-oxothiazolidin-2-ylidene-2-(4-methylphenyl)hydrazoacetonitrile (6f). Brownish yellow crystals; yield (76%); mp 219 oC (EtOH/dioxane); IR (νmax/cm-1): 3181 (NH), 2214 (CN), 1686 (C=O). 1H NMR (DMSO) δH 2.89 (m, 6H, 2CH2), 3.35 (s, 3H, CH3), 7.05-7.75 (m, 8H, Ar), 9.03 (br., 1H, NH). Anal. Calcd for C21H14N4OS (370): C, 68.09; H, 3.81; N, 15.12, S, 8.66. Found: C, 68.30; H, 3.65; N, 15.31; S, 8.48%.
2-(4-Chlorophenylhydrazo)-5(1H-indan-1-ylidene)-4-oxothiazolidin-2-ylideneacetonitrile (6g). Red crystals; yield (90%); mp 258 oC (AcOH); IR (νmax/cm-1): 3186 (NH), 2221 (CN), 1692 (C=O). 1H NMR (DMSO) δH 3.11 (m, 6H, 2CH2), 7.02-8.14 (m, 8H, Ar), 9.33 (br., 1H, NH). Anal. Calcd for C20H11ClN4OS (390): C, 61.46; H, 2.84; Cl, 9.07; N, 14.33, S, 8.20. Found: C, 61.25; H, 2.65; N, 14.58; S, 8.41%.
5-(1H-Indan-1-ylidene)-4-oxothiazolidin-2-ylidene-2-(4-methoxyphenylhydrazo)acetonitrile (6h). Red crystals; yield (87%); mp 246 oC (EtOH/dioxane); IR (νmax/cm-1): 3186 (NH), 2217 (CN), 1690 (C=O). 1H NMR (DMSO) δH 3.14 (m, 6H, 2CH2), 3.75 (s, 3H, OCH3), 7.05-8.15 (m, 8H, Ar), 9.35 (br., s, 1H, NH). Anal. Calcd for C21H14N4O2S (386): C, 65.27; H, 3.65; N, 14.50, S, 8.30. Found: C, 65.48; H, 3.47; N, 14.68; S, 8.48%.
ANTIMICROBIAL ASSAY
Antimicrobial Activity of the samples was determined using a modified Kirby-Bauer disk diffusion method.32 Briefly, 100 µL of the test bacteria/fungi was grown in 10 mL of fresh media until a count of approximately 108 cells/ml for bacteria or 105 cells/mL for fungi was obtained.33 Then, 100 µL of microbial suspension was spread onto agar plates corresponding to the broth from which they were maintained. The inoculated plates were then treated with A flavurs, S aureus, Bacillus subtilis or E coli at 25 oC for 48 h. In the case of Pseudomonas, aeruginosa plates were incubated at 35-37 oC for 24-48 h and for C albicans, the plates were incubated at 30 oC for 24-48 h. After incubation the diameters of the inhibition zones were measured in millimeter. Standard disks of tetracycline (antibacterial agent), amphoericin B (antifungal agent) served as positive controls for antimicrobial activity and filter disk impregnated with 10 µL of solvent (distilled water, CHCl3, DMSO) were used as negative control. Agar-based method such as E test and disk diffusion can be good alternatives because they are simpler and faster than broth-based methods.34
References
1. R. Lakhan and R. L. Singh, J. Agric Food Chem., 1991, 39, 580. CrossRef
2. K. Mogilaiah, Indian J. Chem., 2000, 39B, 277.
3. R. T. Pardasani, P. Pardasani, A. Jain, and S. Kohli, Phoshorus, Sulfur, Silicon and the Related Elements, 2004, 179, 1569. CrossRef
4. N. S. Cutshall, C. O`Day, and M. Prezhdo, Bioorg. Med. Chem. Lett., 2005, 15, 3374. CrossRef
5. P. R. Amélia, M. Padilha, J. A. Figueiredo, M. I. Ismael, J. Justino, H. F. Maria, J. Ferreira, C. Rajendran, R. Wilkins, P. D Vaz, and M. J. Calhorda, J. Carbohydr. Chem., 2005, 24, 275. CrossRef
6. A. J. Alves, E. J. T De Melo, and A. J. S. Goes, Bioorg. Med. Chem. Lett., 2005, 15, 2575. CrossRef
7. J. H. Ahn, S. J. Kim, W. S. Park, S. Y. Cho, J. D. Ha, S. K. Kang, D. G. Jeong, S. K. Jung, S. H. Lee, H. M. Kim, S. K. Park, K. H. Lee, C. W. Lee, S. E. Ryu, and H. K. Choi, Bioorg. Med. Chem. Lett., 2006, 16, 2996. CrossRef
8. J. W. Steele, D. Faulds, and K. L. Goa, Drugs Aging, 1993, 3, 532. CrossRef
9. M. Kawamur and N. Hamanaka, J. Synth. Org. Chem. Japan, 1997, 37, 651.
10. M. Gualtier, L. Bastide, P. V. Guillot, S. C. Michaux, J. Latouche, and J. P. Leonetti, J. Antimicrob. Chemother., 2006, 58, 778. CrossRef
11. L. P. Awasth and S. P. Singh, Folia Microbiol., 1983, 28, 41. CrossRef
12. C. L. Lee and M. M. Sim, Tetrahedron Lett., 2000, 41, 5729. CrossRef
13. A. Zervosen, W. P. Lu, Z. R. Chen, E. White, and J. M. Frere, Antimicrob. Agent Chemother., 2004, 48, 961. CrossRef
14. S. O. Abdallah, , H. A. Ead, N. A. Kassb, and N. H. Metwally, Heterocycles, 1983, 20, 637. CrossRef
15. H. A. Ead, S. O. Abdallah, N. A. Kassab, N. H. Metwally, and Y. E. Saleh, Arch. Pharm. (Weinheim), 1987, 320, 1227.
16. H. A. Ead, S. O. Abdallah, N. A. Kassab, and N. H. Metwally, Sulfur Lett., 1989, 9, 23.
17. H. A. Ead and N. H. Metwally, Arch. Pharm., 1990, 323, 57. CrossRef
18. H. A. Ead, N. H. Metwally, and N. M. Morsy, Arch. Pharm., 1990, 13, 5. CrossRef
19. N. H. Metwally, Indian J. Chem., 2000, 39B, 757.
20. N. H. Metwally, M. A. Abdalla, M. A. Mosselhi, and E. A. M. El-Desoky, Carbohydr. Res., 2010, 345, 1135. CrossRef
21. S. Caddick, Tetrahedron, 1995, 51, 10403. CrossRef
22. P. T. Anastas and J. C. Warner, Green Chemistry: Theory and Practice, Oxford University Press: Oxford 1998.
23. K. Tanaka and F. Toda, Chem. Rev., 2000, 100, 1025. CrossRef
24. D. Villemin and B. A. Alloum, Phosphorus, Sulfur and Silicon, 1993, 79, 33. CrossRef
25. S. Kambe, K. Saito, A. Sakurai, and H. Midorikawa, Synthesis, 1981, 7, 531. CrossRef
26. M. H. Elnagdi, A. E. khalifa, M. K. Ibrahim, and M. R. H. EL-Moghayer, J. Heterocycl. Chem., 1981, 18, 887.
27. M. H. Elnagdi, M. R. H. EL-Moghayer, and A. G. Hammam, Synthesis, 1981, 8, 635.
28. D. F. V. Lewis, B. G. Lake, C. Ioannides, and D. V. Parke, Xenobiotica, 1994, 24, 829. CrossRef
29. M. J. Frisch, G. W. Trucks, H. B. Schlegel, G. E. Scuseria, M. A. Robb, J. R. Cheeseman, V. G. Zakrzewski, J. A. Montgomery, R. E. Stratmann, J. C.Burant, S. Dapprich, J. M. Millam, A. D. Daniels, K. N. Kudin, M. C. Strain, O. Farkas, J. Tomasi, V. Barone, M. Cossi, R. Cammi, B. Mennucci, C. Pomelli, C. Adamo, S. Clifford, J. Ochterski, G. A. Petersson, P. Y. Ayala, Q. Cui, K. Morokuma, D. K. Malick, A. D. Rabuck, K. Raghavachari, J. B. Foresman, J. Cioslowski, J. V. Ortiz, B. B. Stefanov, G. Liu, A. Liashenko, P. Piskorz; I. Komaromi, R. Gomperts, R. L. Martin, D. J. Fox, T. Keith, M. A. Al-Laham, C. Y. Peng, A. Nanayakkara, C. Gonzalez, M. Challacombe, P. M. W. Gill, B. G. Johnson, W. Chen, M. W. Wong, J. L. Andres, M. Head-Gordon, E. S. Replogle, and J. A. Pople Gaussian, 98W (Revision 5.1), Gaussian, Pittsburgh PA, 1998.
30. a) A. D. Becke, J. Chem. Phys., 1993, 98, 5648; CrossRef b) P. J. Stephens, F. J. Devlin, C. F. Chabalowski, and M. J. Frisch, J. Phys. Chem., 1994, 98, 11623. CrossRef
31. C. Lee, W. Yan, and R. G. Parr, Phys. Rev. B, 1988, 37, 785. CrossRef
32. M. A. Pfaller, L. Burmeister, M. A. Bartiett, and M. G. J. Rinaldi, Clin. Microbiol., 1988, 26, 1437.
33. L. D. Liebowitz, H. R. Ashbee, E. G. V. Evans, Y. Chong, N. Mallatova, and M. Zaidi, D. Gibbs, Microbiol. Infect. Dis., 2001, 4, 27. CrossRef
34. M. J. Matar, L. O. Zeichner, V. L. Paetznick, J. R. Rodriguez, E. Chen, and J. H. Rex, Antimicrob. Agents Chemother., 2003, 47, 1647. CrossRef